Abstract
The exact mechanism of hemoglobin-based oxygen carrier (HBOC)–related vasoactivity is still unclear. This study measured the isometric tension of dog arteries and large conductance Ca2+-activated K+ (BKCa) channel currents in vascular smooth muscle cells after exposure to HBOC with increasing concentrations. Data indicated that the net tensions of arteries were dramatically elevated and this elevation was more prominent in coronary artery. Moreover, HBOC exhibited inhibitory effect on BKCa channel, which is strongly correlated with changes in vascular tension. Collectively, HBOC-induced vasoconstriction in a dose-dependent manner and inhibition of BKCa channel is at least partially contributing to this effect.
Introduction
Hemoglobin-based oxygen carrier (HBOC) was initially developed as blood substitutes for surgical and trauma patients with hemorrhagic shock, especially in emergency and in military settings (CitationChang 2005). Although HBOC possesses inherent advantages compared to stored erythrocytes, significant problems associated with their infusion hindered clinical applications. These include brief circulating half-life, renal toxicity, and vasoactivity (CitationChen et al. 2009). HBOC half-life and renal issues have been addressed by increasing the molecular size of the drug via chemical cross-linkage, polymerization, pegylation, or liposome encapsulation, but HBOC vasoactivity remains unsolved. An important reason is that the exact mechanism for this effect has not been completely understood.
Large-conductance Ca2+-activated K+ (BKCa) channels regulate the physiological functions of many tissues, including smooth muscle tone and neuronal excitability (CitationJaggar et al. 2005, CitationSahoo et al. 2013). BKCa channels have a tetrameric structure that are typically composed of pore-forming α subunits encoded by the Slo1 (or KCNMA1) gene and modulatory β subunits (CitationLee and Cui 2010). In smooth muscle cells, BKCa channels regulate cellular membrane potential and modulate the levels of Ca2+ and K+ in cells, providing a mechanism to control cell excitability (CitationJaggar et al. 2000). Its activity can be regulated by a variety of signaling molecules, including intracellular Ca2+, protein kinases, and heme (CitationTang et al. 2003). Moreover, BKCa channels can be activated by physiologically relevant gases, including oxygen (O2), carbon monoxide (CO), and nitric oxide (NO), which have a close relationship with hemoglobin. However, the influence of HBOC on these channels has never been reported and whether this effect contributes to vasoactivity also needs investigation. Therefore, this study was designed to figure out the relationship between HBOC-induced vasoactivity and BKCa channels.
Materials and methods
All of the experiments were approved by the Institutional Animal Care and Use Committee of Sichuan University, and all of the animals received human care in compliance with the Guide for the Care and Use of Laboratory Animals published by the US National Institutes of Health (NIH Publication No. 85-23, revised 1996). Adult male beagle dogs, approximately 6–8 months old and weighing 8–10 kg, were housed at constant temperature (22 ± 3°C) on a 12-h light/dark cycle and were given free access to food and water.
Hemoglobin-based oxygen carrier
The HBOC used in this study was glutaraldehyde-polymerized human placenta hemoglobin (PolyPHb, 8–10 gHb/dL, methemoglobin < 3%, tetrameric hemoglobin < 1%), which was prepared as reported previously (CitationLi et al. 2006). Briefly, purified and viral inactivated fresh human placenta hemoglobin (Tianjin Union stem cell genetic engineering Ltd, Tianjin, China) was modified with bis(3,5-dibromosalicyl) fumarate to achieve optimal O2 affinity. After cross-linkage with glutaraldehyde, the mixture was subject to ultrafiltration and molecular sieve chromatography. The final product had a molecular weight of 64–600 kDa.
Measurement of vascular reactivity on isolated vessel rings
Arterial rings (3–4 mm in length) from beagle dog femoral artery, carotid artery, and coronary artery, free from fat and connective tissue, were mounted between two stainless steel hooks in organ bath chambers (PanLab Systems, Harvard apparatus, Barcelona, Spain). Each chamber contained 10 mL of Krebs-Henseleit (KH) solution (118 mM NaCl, 4.7 mM KCl, 1.2 mM KH2PO4, 1.2 mM MgSO4, 1.77 mM CaCl2, 25 mM NaHCO3, 11.4 mM glucose; pH 7.4, 37°C) and aerated continuously with 95% O2 and 5% CO2. Special attention was paid during the preparation to avoid damaging the endothelium. During 60 min of equilibration period, resting tension of 3.5 g was periodically adjusted and the KH solution was changed every 30 min. The arterial viability was checked by stable and reproducible constriction to the addition of potassium chloride (KCl, 60 mM). Contracted arteries were then washed and subjected to 30 min of equilibration. Subsequently, these arteries were incubated with different concentrations of HBOC (0.1, 0.5, 1, 2, and 3 gHb/dL) for 10 min and isometric tension of each vessel was recorded.
Isolation of vascular smooth muscle cells
The vascular smooth muscle cells (VSMCs) were isolated from dog coronary arteries using an enzyme procedure with modifications (CitationJaggar et al. 2005). Briefly, arteries were placed into isolation solution (55 mM NaCl, 80 mM sodium glutamate, 5.6 mM KCl, 2 mM MgCl2, 10 mM HEPES, and 10 mM glucose, pH 7.3) containing 0.3 mg/mL papain, 1 mg/mL dithioerythreitol, and 1 mg/mL bovine serum albumin (BSA) for 20 min (at 37°C) and were immediately transferred to isolation solution containing 1 mg/mL collagenase, 100 mM CaCl2, and 1 mg/mL BSA for 10 min (at 37°C). Arteries were subsequently washed in ice-cold isolation solution for 10 min and triturated to yield single smooth muscle cells. After adhering to a glass coverslip at the bottom of a chamber, thosee VSMCs with appearing bright and smooth appearance were selected for recording.
Electrophysiology recording
Whole-cell large-conductance Ca2+-activated K+ (BKCa) currents were recorded at room temperature (22–24 °C) with the conventional voltage clamp configuration. Holding potential was set at –80 mV. Current densities were obtained by normalizing currents to the cell membrane capacitance. The extracellular (bath) solution contained 135 mM NaCl, 5.2 mM KCl, 1.0 mM MgCl2, 10 mM HEPES, 0.5 mM CaCl2, 10 mM glucose, and 5.0 mM 4-aminopyridine (4-AP), pH was adjusted to 7.2 by NaOH. 4-AP in the bath solution was used to exclude the interference from voltage-dependent K+(KV) channel currents. The internal (pipette) solution contained 70 mM KCl, 60 mM K+-Asp, 5 mM K2ATP, 2.5 mM EGTA, 1 mM MgCl2, 1.8 mM CaCl2, and 10 mM HEPES, pH was adjusted to 7.4 by KOH. Patch electrodes were made from the fire-polished borosilicate glass capillaries (Sutter Instrument Co., Novato, CA, USA) using a micropipette puller (model P-97, FLAMING/BROWN micropipette puller; Sutter Instrument Co. Novato, CA). Electrode resistance was 4 to 5 MΩ. Currents were sampled at 10 kHz using an Axon 200B amplifier, digitized via a Digidata 1440A interface and analyzed using pClamp 10.0 (Axon/Molecular Devices, Sunnyvale, CA). The properties of the BKCa channel were identified by exposure of VSCMs to 100 nM iberiotoxin (IBTX, a specific BKCa inhibitor) and high Ca2+. Next, HBOC solution was diluted with extracellular solution to required concentrations, and then applied locally to attached VSMCs at a speed of 150 μL/min through a perfusion pipette (diameter, 0.2 mm) positioned 30 to 50 μm away from the patched cells. BKCa channel currents of VSMCs were recorded before and after exposure to indicated treatment.
Statistical analysis
All values are presented as mean ± SD. One-way ANOVA was used to compare the differences among three groups, followed by Bonferroni's multiple comparison tests as applicable (SPSS 16.0 software). A simple linear regression analysis was performed to estimate the correlation between changes of vascular tension and BKCa channel currents. P values < 0.05 were considered statistically significant.
Results
HBOC constricts arteries in a dose-dependent manner
First, we tested the effect of HBOC on the net tension of arteries using an in vitro organ bath study. Isolated femoral, carotid and coronary arteries from dogs were incubated with increasing concentrations of HBOC at 37°C for 10 min. Data indicated that the net tensions of these arterial rings were dramatically elevated with increased HBOC concentration. Interestingly, this elevation was more prominent in the coronary artery (0.29 ± 0.06 g) compared with that in the femoral artery (0.16 ± 0.07 g, P < 0.05, ) and carotid artery (0.21 ± 0.08 g, P < 0.05).
High-dose HBOC inhibits BKCa channel currents of VSMCs
The effects of HBOC on BKCa channels were evaluated using a whole-cell patch-clamp technique (). The properties of the BKCa channel were identified by this technique and the recorded voltage-dependent outward currents were significantly blocked by 100 nM IBTX and enhanced by high Ca2+ (). As shown in , acute addition of 0.1% HBOC to the bath solution slightly increased whole-cell outward currents. In contrast, at concentrations greater than 0.5%, HBOC inhibited whole-cell currents in a dose-dependent fashion. After exposure to 1%, 2%, 3% HBOC, the BKCa channel currents were reduced by 26.84 ± 11.86%, 46.72 ± 14.02%, and 62.53 ± 11.32%, respectively.
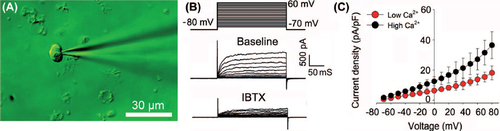
Inhibition of BKCa channel currents is strongly correlated to the vasoconstriction
Linear regression analysis indicated a strong correlation between HBOC-induced vasoconstriction and BKCa channel currents inhibition (). The related coefficients of these two parameters for femoral, carotid, and coronary arteries are all greater than 0.9 (). These data provide mechanistic evidence that inhibition of the BKCa channel may contribute to HBOC-induced vasoconstriction.
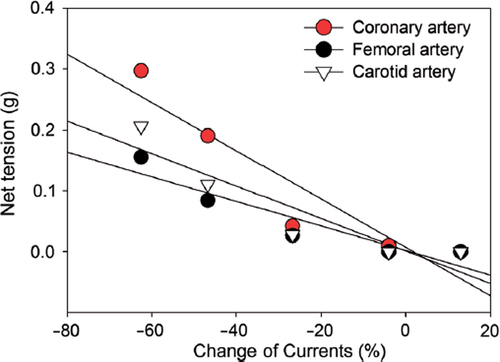
Table I. Linear regression analysis between BKCa channel inhibition and vasoconstriction.
Discussion
The well-known vasoactivity of HBOC is a major obstacle hindering its clinical development, which may contribute to gastrointestinal side effects, hypertension, chest pain, and even myocardial infarction (CitationHai 2012, CitationLi et al. 2014). Our current study confirmed this phenomenon and further indicated that the vasoactivity of HBOC is dose dependent. In addition, we first observed that the BKCa channel currents of VSMCs can also be inhibited after exposure to increasing concentrations of HBOC.
The exact mechanism of HBOC-induced vasoactivity has not been fully understood by now. The removal of vascular endothelial NO, a potent vasodilator molecule, has been attributed as the major cause of this effect. The findings that inhalation of NO or intravenous administration of an NO donor, such as sodium nitrite and nitroglycerin, prevent systemic hypertension induced by HBOC have strengthened this theory (CitationKatz et al. 2010, CitationYu et al. 2008). An alternative concept to the direct NO scavenging is oversupply of O2, a known vasoconstrictor. This theory points out that the current HBOC products, even at a low concentration, can greatly augment tissue O2 supply, which may engage protective mechanisms that include vasoconstriction to counteract the ‘poisonous’ O2, possibly through reactive oxygen species (ROS) formation and local destruction of NO (CitationAlayash 2014). Based on this theory, some researchers proposed that the HBOC should have a higher O2 affinity so as to reduce the oversupply of O2 and decrease vasoactivity (CitationWinslow 2008). Besides, our recent study found that HBOC elevated NAD(P)H oxidase-induced ROS production and reduced vascular NO bioavailability, which synergistically caused endothelial dysfunction and aggravated cardiovascular side effects (CitationLi et al. 2014).
Different from the aforementioned theories, the present study provided distinct evidence that HBOC, at concentrations greater than 0.5%, can dose-dependently inhibit BKCa channel currents. This provides a novel mechanism for HBOC-induced vasoactivity and hypertension. It is well known that BKCa channels contribute to the regulation of vascular tone in a negative feedback manner (CitationHill et al. 2010). Activation or opening of BKCa channels hyperpolarizes the membrane potential and leads to vasodilation, whereas inhibition of BKCa channels promotes depolarization and causes vasospasm or constriction. The data of linear regression analysis indicated that inhibition of BKCa channel currents is strongly correlated with HBOC-induced vasoconstriction, providing an alternative explanation for the hypertension and cardiovascular adverse effects observed in clinical studies about HBOC. However, in a normal vascular system, HBOC cannot easily interact with vascular smooth muscle due to the endothelial barrier. In pathological conditions, in which endothelial damage is a component, the likelihood of such interactions is greatly increased. That such vasoactivity and adverse outcomes of HBOCs can be synergized by hypertension, atherosclerosis and diabetes is therefore expected. Thus, protection of the vascular endothelium and stabilization of endothelial function can probably mitigate HBOC-related adverse cardiovascular effects.
In summary, our study demonstrated that HBOC-induced vasoconstriction in a dose-dependent manner and inhibition of BKCa channel currents is at least partially contributing to this effect.
Notice of Correction
The version of this article published online ahead of print on 01 Jul 2014 contained an error in the declaration of interest. The grant number “81070117” should have read “81100180 and 81471042”. The error has been corrected for this version.
Declaration of interest
The authors report no declarations of interest. The authors alone are responsible for the content and writing of the paper.
This study was supported by grants from the National Natural Science Foundation of China (81100180 and 81471042), the Specialized Research Fund for the Doctoral Program of Higher Education (20100181120090), the National High Technology Research and Development Program of China (863 Program, 2012AA021903), the Science and Technology Support Program of Sichuan Province (2013SZ0059), the Special Foundation for Technology Innovation Program of Sichuan Province (2013ZZ0006), the Major Program of the Clinical High and New Technology of PLA (2010gxjs039) and Research Funds in Chengdu Military Hospital (2011YG-B08 and 2013YG-B028).
References
- Alayash AI. 2014. Blood substitutes: why haven't we been more successful?Trends Biotechnol. 32:177–185.
- Chang TMS. 2005. Therapeutic applications of polymeric artificial cells. Nat Rev Drug Discov. 4:221–235.
- Chen JY, Scerbo M, Kramer G. 2009. A review of blood substitutes: examining the history, clinical trial results, and ethics of hemoglobin-based oxygen carriers. Clinics. 64:803–813.
- Hai CM. 2012. Systems biology of HBOC-induced vasoconstriction. Curr Drug Discov Technol. 9:204–211.
- Hill MA, Yang Y, Ella SR, Davis MJ, Braun AP. 2010. Large conductance, Ca2+-activated K+ channels (BKCa) and arteriolar myogenic signaling. FEBS Lett. 584:2033–2042.
- Jaggar JH, Li A, Parfenova H, Liu J, Umstot ES, Dopico AM, Leffler CW. 2005. Heme is a carbon monoxide receptor for large-conductance Ca2+-activated K+ channels. Circ Res. 97:805–812.
- Jaggar JH, Porter VA, Lederer WJ, Nelson MT. 2000. Calcium sparks in smooth muscle. Am J Physiol Cell Physiol. 278: C235–C256.
- Katz LM, Manning JE, McCurdy S, Sproule C, McGwin G Jr, Moon-Massat P, et al. 2010. Nitroglycerin attenuates vasoconstriction of HBOC-201 during hemorrhagic shock resuscitation. Resuscitation. 81:481–487.
- Lee US, Cui J. 2010. BK channel activation: structural and functional insights. Trends Neurosci. 33:415–423.
- Li T, Yu R, Zhang HH, Wang H, Liang WG, Yang XM, Yang CM. 2006. A method for purification and viral inactivation of human placenta hemoglobin. Artif Cells Blood Substit Immobil Biotechnol. 34:175–188.
- Li T, Zhou R, Yao Y, Yang Q, Zhou C, Wu W, et al. 2014. Angiotensin-converting enzyme inhibitor captopril reverses the adverse cardiovascular effects of polymerized hemoglobin. Antioxid Redox Signal. [Epub ahead of print]
- Sahoo N, Hoshi T, Heinemann SH. 2013. Oxidative Modulation of Voltage-Gated Potassium Channels. Antioxid Redox Signal. [Epub ahead of print]
- Tang XD, Xu R, Reynolds MF, Garcia ML, Heinemann SH, Hoshi T. 2003. Haem can bind to and inhibit mammalian calcium-dependent Slo1 BK channels. Nature. 425:531–535.
- Winslow RM. 2008. Cell-free oxygen carriers: scientific foundations, clinical development, and new directions. Biochim Biophys Acta. 1784:1382–1386.
- Yu B, Raher MJ, Volpato GP, Bloch KD, Ichinose F, Zapol WM. 2008. Inhaled nitric oxide enables artificial blood transfusion without hypertension. Circulation. 117:1982–1990.