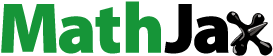
Abstract
This study aims to prepare spray-dried inhalable powders containing anti-tubercular drugs–loaded HPMC nanoaggregates for sustained delivery of drugs to the lung. Nanoaggregates were prepared by precipitation technique. Results showed that the powders obtained had excellent aerosolization property. High drug encapsulation efficiency was achieved in HPMC nano aggregates, ranging from 60% to 70%. A single pulmonary dose resulted in therapeutic drug concentrations 40% to 60% in the lungs and in other organs (< 5%) for 24 h. From this study, we can conclude that delivering drugs through pulmonary route is advantageous for local action in lungs.
Introduction
Tuberculosis (TB) is a communicable disease spread by breathing of the airborne droplets comprising tubercle bacilli, Mycobacterium tuberculosis. The prevalence of mycobacterial infections has increased rapidly in recent years; one-third of the world's population is infected with M. tuberculosis. Bacillus Calmette-Guerin (BCG) vaccination and chemoprophylaxis appear to be unsatisfactory options for control and treatment of tuberculosis (CitationKaur et al. 2014a). The utility of conventional chemotherapy is limited because of inability to deliver therapeutic drug concentration at the site of action or severe and toxic side effects on normal tissues and organs. The major reasons for limitations of current chemotherapy includes poor penetration, higher dosing, systemic toxicity, high failure rates, drug resistance, non-specific drug delivery, reduced bioavailability due to first-pass effect, and long duration of therapy (CitationKaur et al. 2014b). Different tactics have been endeavored to overcome these problems by providing selective delivery to the affected area. These can be achieved using inhalable or aerosolized nanocarrier systems delivered directly to the macrophages, resulting into many folds higher intracellular concentration of drug candidate. There are different routes of administration for the delivery of drugs. The choice of route depends on the properties of the drug, site of desired action, and the condition of the patient. Among these routes, the use of novel inhalable drug delivery systems has received considerable attention in the past few years (CitationToosky and Javid 2014). The major advantages of these routes are local delivery of drugs to lungs, avoidance of first-pass metabolism, enhanced bioavailability of drug, reduced systemic side effects, reduced the dose frequency, and shortened treatment period as compared to oral or systemic treatment protocols (CitationParida et al. 2014).
Colloidal carriers (nanocarrier systems) in pulmonary drug delivery compromise many advantages such as the potential to achieve relatively uniform distribution of drug dose among the alveoli, improved solubility of the drug from its own aqueous solubility, sustained drug release that consequently reduces dosing frequency, to improve patient compliance, decrease incidence of side effects, and improve the potential of drug internalization by cells (CitationGarg et al. 2012). However, nanoparticle delivery to the lungs faces many challenges, including formulation instability due to particle–particle interactions and poor delivery efficiency due to exhalation of low-inertia nanoparticles. Porous nano-aggregate particles (PNAPs) organize to be a novel particulate form incorporating nanoparticles into micron-scale structures to overcome the problems of loading and delivering nanoparticles to the lungs. These structures hold the assets of both nano- and micron-scale particles (CitationGarg and Goyal 2014). By formulating nanoparticles into larger hollow or porous structures, the dry powders are highly dispersible, easy to handle, and efficiently aerosolized to deposit in the respiratory tract and they can be delivered more easily into the lungs with a simple inhaler (CitationLi et al. 2014). HPMC (Hydroxy propyl methyl cellulose) is a semi-synthetic, inert, viscoelastic polymer used as an excipient and controlled-delivery component in medicaments. It is one of the most commonly used hydrophilic biodegradable polymers for developing controlled release formulations, because it works as a pH-independent gelling agent. Upon contact with water or biological fluid, the latter diffuses into the device, resulting in polymer chain relaxation with volume expansion. Subsequently, the incorporated drug diffuses out of the system. The present work describes the pharmacokinetic potential of pulmonary administered first-line antitubercular drugs; that is, isoniazid and rifampicin have been loaded in HPMC-based nanoaggregates for pulmonary delivery by dry powder inhalers (DPIs) (CitationGeorge et al. 2014).
Materials and methods
Chemicals and drugs
HPMC, ethanol, acetone, chloroform, mannitol, leucine and Tween 80 were purchased from CDH Laboratory reagents (New Delhi). Isoniazid (INH) and Rifampicin (RIF) were procured from BV Patel Centre (Ahmadabad, India). Methanol was purchased from HIMEDIA (Mumbai, India). All the materials used in this study were of analytical grade and obtained from standard companies.
Animals
Wistar rats of both sexes (180–220 g) obtained from ISF College of Pharmacy, Moga, India, were used in this study and these were housed in an animal house facility under natural light conditions. The animals were fed a standard pellet diet and water as required. The project was approved by the Institute Animal Ethics Committee.
Preparation of HPMC nanoporous aggregates
HPMC nanoporous aggregates (NPs) were prepared by precipitation method. In this method, HPMC (5cps) in the concentration range of 0.2–1% was dissolved in water and 0.1% of Tween 80 was added to it using a homogenizer (IKA, T-25 DS 22) for 5 min at 3000 rpm. Diethyl ether in the range of 0.3 to 0.9 ml was used and 1% span 80 solutions were added to it. Then to the HPMC solution, diethyl ether solution was added drop wise using a homogenizer for 5 min at 3000 rpm. Spray drying was done using mannitol and leucine. Drugs (RIF and INH)-loaded NPs were prepared by adding drugs in different amounts in ether and added drop wise to the HPMC solution using a homogenizer. The process yield of spray-dried powder can be calculated as follows:
Characterization of NPs
Morphological studies of NPs
The particle size distribution of drugs-loaded NPs and the corresponding NPs prepared with the same HPMC polymer in absence of the drug were determined by dynamic light scattering (DLS) using a Zetasizer Nano Instrument (Malvern Instruments, Zeta sizer, UK). Scanning electron microscopy (SEM) was used to examine the morphology of the NPs prepared in this study. SEM analysis was done in National Institute of Pharmaceutical Education & Research, Mohali, India. From SEM images, mean aerodynamic diameter was calculated.
Moisture content of NPs
The Karl Fisher volumetric titration technique was used for moisture content analysis of the dry powders. This technique is mainly designed to determine the water content in substances. In this technique, the moisture content in a sample is determined by measuring the quantity of electricity, which is required for the electrolysis (i.e., for the production of iodine), based on the quantitative reaction of the generated iodine and sulfur dioxide in the presence of a lower alcohol, such as methanol, and an organic base (B), such as imidazole or pyridine, with water. The water content was measured in triplicate.
Density profile analysis of NPs
The spray-dried formulations were taken and the bulk volume was noted. The formulations were then tapped 100 times and the tapped volume was noted. Furthermore, the Carr's index and Hausner's ratio calculated by using formula
Where Vb = bulk volume, Vt = tapped volume.
Angle of repose analysis of NPs
Angle of repose analysis was determined by fixed funnel method. In this technique, the pile of the powders was carefully built up by dropping the powder material through a funnel tip from a height of 2 cm. The angle of repose was calculated as follows:
Where, θ is angle of repose, h is height of the particles pile, and r is distance from the center of the pile to the edge.
Evaluation of drug entrapment
The drug-loaded NPs were separated from non-entrapped drug by ultracentrifugation at 18,000 rpm for 40 min at 4°C. Supernatant (1 ml) was taken and diluted (up to 100 ml) with water and methanolic phosphate buffer solution in case of INH and RIF, respectively. Finally, drug content was analyzed at 261.90 nm and 473 nm for INH and for RIF, respectively, by using UV-spectrophotometer (Perkin Elmer, Tokyo, Japan) (CitationParnami et al. 2013). The percent of entrapment efficiency (EE %) of NPs was calculated according to the following equations:
In vitro drug release studies
Dialysis tubing technique was used for the determination of in vitro release of antitubercular drugs (ATDs) from the optimized formulations. In brief, accurately weighed ATDs-loaded HPMC porous nanoaggregates were transferred into presoaked dialysis membrane and suspended in a conical flask (100 ml) containing 25 ml of methanolic PBS for simultaneous estimation of both the ATDs. The whole set was placed in a shaking incubator (constant speed 100 rpm) (Daihan Labtech Co. Ltd., Kyonggi-Do, Korea), maintained at a temperature of 37°C. Samples were collected at different time intervals of 24 h for HPMC NPs and assayed spectrophotometrically at 261.90 nm and 473 nm for INH and for RIF, respectively (CitationSingh et al. 2014).
In vivo study
Wistar rats of either sex (200–220 gm) were used to study pharmacokinetics and organ distribution of optimized formulations. All experiments were conducted as per CPCSEA (Committee for Prevention, Control and Supervision of Experimental animals, approval No. ISF/CPCSEA/IEAC/2013/100) guidelines. Organ distribution and pharmacokinetic parameters were obtained after pulmonary administration of HPMC nanoaggregates. For the above purpose, dry powder insufflator (Model DP-4M), Penn-century, INC. instrument was used for the pulmonary delivery of drugs. First, standard curves were plotted in different organs (lungs, liver, and kidney). For organ distribution studies, various organs such as lungs, liver, and kidney were removed at fixed intervals of 1, 2, 4, 8, and 24 h after blood sampling. Organ homogenates were prepared in phosphate buffer using organ homogenizer (Dathan Scientific, SV Instruments Analytica Pvt. Ltd., New Delhi, India) at 6000 rpm for 30 min. Then, 0.2 ml of the supernatant from different organs was taken, followed by acetonitrile (purpose of deproteinization). Vortexing was done for 5 min, and then centrifugation was done for 15 min at 3000 rpm. Next, 1 ml of the supernatant was removed from the centrifuged samples and diluted with 1 ml of methanolic PBS and assayed spectrophotometrically (CitationGarg et al. 2013).
Results and discussion
Preparation and characterization of HPMC NPs
HPMC nanoporous aggregates were successfully prepared by precipitation technique. Optimized formulation was selected on the basis of various parameters such as solvent (), HPMC concentration (), and drug entrapment ( and ).
Table I. Optimization of solvent volume.
Table II. Optimization of HPMC concentration.
Table III. Isoniazid entrapment optimization in optimized HPMC batch (P-HNP 2).
Table IV. Rifampicin entrapment optimization in optimized guar gum batch (P-HNP 2).
Solvent volume was optimized from 0.3 to 0.9 ml on the basis of particle size and polydispersity index. The optimized formulation was found to be S-HNP 1. It was done using solvent from 0.3 to 0.9 ml by keeping the amount of polymer constant (1%). It was observed that formulation having code S-HNP 1 showed less particle size and PDI as compared with other formulations. Thus, S-HNP 1 was the optimized formulation with particle size ∼1419.0 nm and PDI ∼0.404. Results show that diethyl ether develops an environment for controlled precipitation of HPMC. As the diethyl ether concentration was increased, there was an abrupt increment of PDI and in size. This clearly indicated that diethyl ether at higher concentration replaces more of the water from the molecular space, thus resulting in an increased terminal hydrophobicity of polymer and leads to uncontrolled precipitation of HPMC.
It was observed that particle size was increased as the concentration of HPMC was varied from 0.2% w/v to 1% w/v, when solvent was kept constant (0.3 ml). The particle size was increased because the viscosity of the polymeric solution was increased with increasing amount, but on the basis of size and PDI alone it was difficult to determine the optimized formulation. So, drug loading was done and maximum loading was found with formulation having code P-HNP 2 (69%) with particle size ∼544.1 nm and PDI of ∼0.269.
Similarly, loading efficacy of the developed formulations was determined by varying the amount of drug, while the polymer concentration was kept constant ( and ). The drug to polymer ratio was varied from 1:4 to 5:4 for both the drugs and the optimized formulation was I-HPN 2 for isoniazid with particle size of ∼544.1 nm, PDI ∼0.269, drug entrapment 69.2%, and for rifampicin the optimized formulation was R-HPN 2 with particle size of ∼615.0 nm, PDI ∼0.338 and drug entrapment 69%. From the, it was observed that by increasing the amount of drug when polymer was kept Studies revealed that variation in concentration of drugs during the formulation of nanoparticles have regulated the overall morphology of resultant particulate systems. Although I-HPN-1 exhibit smaller particle size, narrow PDI and higher drug entrapment, I-HPN-1 was not selected for further investigation because of its poor yield and lower drug loading capacity. The formulation intended for pulmonary administration has poor loading capacity and requires higher quantities to attain therapeutic dose which is difficult to manage while carrying out the animal studies. Therefore, I-HPN-2 was selected for in vivo studies.
Characterization of optimized formulations after spray drying
To improve the yield and flow properties of powders, mannitol and leucine were used as excipients in the spray drying process. Mannitol was added to improve the yield and the flow properties of experimental formulation. Leucine was used because of its dispersibility-enhancing properties, which improved the aerosolization characteristic of powders. represents some characterization parameters of optimized formulations after spray drying.
Table V. Characterization of optimized formulations after spray drying.
The particle size of spray dried powders decreased significantly on addition of leucine, and there is increase in the process yield because of its anti-adherent properties. The optimized ratio of mannitol and leucine was 1% and 0.2% for HPMC nanoaggregates on the basis of desired flow properties. The inlet temperature was maintained at 80°C, outlet temperature at 40–50°C, and vacuum at 100–120 of WC. Results of the micrometrics study of spray-dried formulations indicated that all the parameters were found within the specification required for pulmonary administration. In particular, aerodynamic diameter below 5 μm is considered to be essential for the formulation to access the infection site. In the present study, both the formulations exhibit an aerodynamic diameter of 2.74 and 3.82 μm for isoniazid and rifampicin, respectively. The data observed from indicate good flow property of the powders.
SEM images of optimized HPMC nanoaggregates
Optimized formulations were visualized under SEM for surface morphology. SEM photographs revealed that spray-dried nanoaggregates were spherical. SEM images of optimized formulations are shown in . These images further revealed that the particles were spherical, homogeneous, and uniform in nature. Moreover, the optimized compositions provide a conducive atmosphere for controlled evaporation of solvent during the specified spray dried conditions, resulting in the homogeneous distribution of aggregates.
In vitro release studies
In vitro drug release profile of optimized formulations using dialysis bag in methanolic PBS (pH 7.4) was shown in . Release pattern of HPMC-based nanoparticles was faster and observed for 24 h, in which isoniazid showed 76% drug release and rifampicin showed 59%. The release rate of isoniazid was more that of rifampicin because of the more solubility of isoniazid in methanolic PBS. Further, relatively slow release of rifampicin could be attributed to the hydrophobic interaction between rifampicin and the hydrophobic site of HPMC, which is expected during precipitation process.
In vivo studies
Wistar rats of either sex (200–220 g) were used for pharmacokinetics and organ distribution studies of optimized formulations. represents the amount of drug distributed in the different organs (lungs, liver, and kidney). These results showed that a maximum amount of drug reaches lungs through the pulmonary route. Experimental formulations have showed greater accumulation of drug (formulation form) in the lungs compared to free drug and this statement is proved by organ distribution studies. Further, in case of plain drugs, no drug was detected in the lungs after 24 h. However, approximately 25.21% drug was estimated after 24 h of administration in case of experimental formulations. Results of organ distribution showed a significant low level of drug distribution (from formulations) in the vital organs as compared to plain drug due to a controlled release of the drugs from the developed formulation. The observed values further suggested that the pulmonary administration of prepared formulation using HPMC is effective in rapid attainment of high-drug concentrations in alveolar macrophages (lungs) as well as in maintaining the concentration over a prolonged period of time when compared to plain drug. This establishes the significance of the targeting potential of the developed systems.
Pharmacokinetic parameters
Peak plasma concentration (Cmax), time taken to reach Cmax (Tmax), elimination half-life (t1/2), area under plasma drug concentration over time curve (AUCtot), and mean residence time were calculated using Kinetic 5.0 software. represents various pharmacokinetic parameters of optimized formulations.
Table VI. Pharmacokinetic data of optimized formulations.
Peak plasma concentrations of the drug were achieved quickly in case of plain drug compared to the developed formulation. However, the mean residence time of the drug in plasma remained for a longer period with developed systems. This may be attributed to the controlled release of the drug from the prepared formulation. Further, the biological half-life (t1/2) of the prepared formulation was found to be significantly higher than the plain drugs, accounted to be 8.40 ± 1.15 h and 12.6 ± 1.24 h for isoniazid and rifampicin, respectively, against plain drugs, which was 2.91 ± 1.20 h and 3.7 ± 0.5 h, respectively. The results further supported the sustained release behavior of the developed formulation. The AUCs of prepared formulations were 187.4 ± 12.58 μg/ml/h and 349.8 ± 16.9 μg/ml/h for isoniazid and rifampicin, respectively, which was higher compared to plain drug, which could be due to maintenance of drug concentration within the pharmacologically effective range for longer period of time from the developed formulations. It could be concluded from the pharmacokinetic studies that the anti-tubercular drug-loaded HPMC nanoaggregates would be available at the infection site above MIC for a longer period to produce the desired pharmacological effect.
Conclusion
Anti-tubercular drugs (isoniazid and rifampicin)-loaded porous nano-aggregates were successfully prepared using HPMC as polymers by precipitation technique. The effect of polymer concentration, solvent ratio, and drug concentration affects the characteristics of nano-aggregates. The optimized inhalable formulations have demonstrated excellent flow properties. In vitro release studies showed sustained release of drugs up to 24 h from HPMC-based formulations. Further, alveolar deliveries of developed nanoparticulate formulations in animal have shown predominant deposition of drugs within the lungs. Optimized formulations showed sustained drug release pattern, which could be beneficial for reducing the drug dose or frequency of dosing, thus helpful in improving patient compliance.
Declaration of interest
The authors report no declarations of interest. The authors alone are responsible for the content and writing of the paper.
Tarun Garg is thankful to Punjab Technical University (PTU), Kapurthala, Punjab, for providing an opportunity to carry out the research work. Dr. Amit K. Goyal (under IYBA scheme; BT/01/IYBA/2009 dated 24/05/2010) is thankful to the Department of Biotechnology (DBT), New Delhi, India, for providing financial assistance to carry out the research work.
References
- Garg T, Goyal AK. 2014. Biomaterial-based scaffolds–current status and future directions. Expert Opin Drug Deliv. 11:767–789.
- Garg T, Singh O, Arora S, Murthy R. 2012. Scaffold: a novel carrier for cell and drug delivery. Crit Rev Ther Drug Carrier Syst. 29:1–63.
- Garg T, Singh S, Goyal AK. 2013. Stimuli-sensitive hydrogels: an excellent carrier for drug and cell delivery. Crit Rev Ther Drug Carrier Syst. 30:369–409.
- George J, Kumar R, Sajeevkumar VA, Ramana KV, Rajamanickam R, Abhishek V, et al. 2014. Hybrid HPMC nanocomposites containing bacterial cellulose nanocrystals and silver nanoparticles. Carbohydr Polym. 105:285–292.
- Kaur M, Garg T, Rath G, Goyal AK. 2014a. Current nanotechnological strategies for effective delivery of bioactive drug molecules in the treatment of tuberculosis. Crit Rev Ther Drug Carrier Syst. 31: 49–88.
- Kaur M, Malik B, Garg T, Rath G, Goyal AK. 2014b. Development and characterization of guar gum nanoparticles for oral immunization against tuberculosis. Drug Deliv. [Epub ahead of print]
- Li X, Vogt FG, Hayes D Jr, Mansour HM. 2014. Design, characterization, and aerosol dispersion performance modeling of advanced spray-dried microparticulate/nanoparticulate mannitol powders for targeted pulmonary delivery as dry powder inhalers. J Aerosol Med Pulm Drug Deliv. 27:81–93.
- Parida SK, Axelsson-Robertson R, Rao MV, Singh N, Master I, Lutckii A, et al. 2014. Totally-drug resistant tuberculosis and adjunct therapies. J Intern Med. doi:https://doi.org/10.1111/joim.12264. [Epub ahead of print]
- Parnami N, Garg T, Rath G, Goyal AK. 2013. Development and characterization of nanocarriers for topical treatment of psoriasis by using combination therapy. Artif Cells Nanomed Biotechnol. [Epub ahead of print]
- Singh H, Sharma R, Joshi M, Garg T, Goyal AK, Rath G. 2014. Transmucosal delivery of Docetaxel by mucoadhesive polymeric nanofibers. Artif Cells Nanomed Biotechnol. [Epub ahead of print]
- Toosky M, Javid B. 2014. Novel diagnostics and therapeutics for drug-resistant tuberculosis. Br Med Bull. 110:129–140.