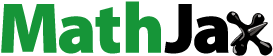
Abstract
The aim of this work was to evaluate the effect of various production parameters on the formation and particle size of poly (3-hydroxybutyrate-co-3-hydroxyvalerate) PHBV particles prepared by the emulsification-diffusion technique. The increase in homogenization time and speed caused a decrease in particle size. No particle formation was observed below 2% (w/v) PHBV in the organic phase. Smaller particle size and narrower size distribution were observed when polyvinyl alcohol (PVA) was used as a stabilizer, when compared to didodecyldimethylammonium bromide. Submicron particles of 531 ± 150 nm size were obtained with 2% (w/v) PVA at 17 500 rpm and 15 min homogenization conditions with dichloromethane as the organic solvent.
Introduction
Polymeric particles play a pivotal role in medical applications as carrier systems for the delivery of bioactive agents like drugs and growth factors (CitationKowalczuk et al. 2014). An optimal carrier should provide the spatiotemporal release of bioactive molecules and should also be biocompatible, noncytotoxic and biodegradable (CitationVo et al. 2012). Poly (hydroxybutyrate-co-hydroxyvalerate) (PHBV) is a microbial polyester synthesized by various microorganisms. It has been reported that PHBV shows good biocompatibility and has been attractive as a biomaterial for tissue engineering (CitationZhu et al. 2009) and particle based drug delivery systems (CitationVilos et al. 2013, CitationMasood et al. 2013). The chemical structure and physicochemical properties of PHBV are similar to the commonly studied polyester poly (lactide-co-glycolide) (PLGA) (CitationVroman and Tighzert 2009). On the other hand, it degrades at a much slower rate than PLGA (CitationYilgor et al. 2010) and it is a more cost effective alternative due to its biotechnological production (CitationVilos et al. 2013). Although there are plenty of studies on the production of PLGA particles as carrier systems, few studies have been reported on the physicochemical properties of PHBV particles prepared using different process parameters (CitationPich et al. 2006). CitationBaran et al. (2002) have used sodium borohydride pretreated PHBV to prepare enzyme encapsulated PHBV nanoparticles by the double emulsion solvent evaporation technique. In another study by CitationPoletto et al. (2008) PHBV nanospheres and oily nanocapsules were prepared by the emulsification-diffusion technique by employing binary mixtures of chloroform-ethanol in the organic phase.
The aim of this work was to evaluate the effects of various production parameters in the emulsification-diffusion technique on the formation and properties of PHBV particles prepared by using a preformed polymer without further modification. Homogenization conditions and polymer concentration were varied to evaluate their effects on particle formation. Didodecyldimethylammoniumbromide (DMAB) was used as a stabilizer for the first time in the preparation of PHBV particles and compared with poly (vinyl alcohol) (PVA) as stabilizer. A solvent type effect was introduced by using chloroform, DCM and binary mixtures of DCM with ethanol. Finally, submicron PHBV particles produced under optimized conditions were loaded with a model protein bovine serum albumin (BSA) to evaluate the encapsulation efficiency and protein release kinetics.
Materials and methods
Materials
Poly (3-hydroxybutyric acid-co-3-hydroxyvaleric acid) (PHBV) with 12 wt% PHV, polyvinyl alcohol (PVA) (average molecular wt. 13,000–23,000) and didodecyldimethylammonium bromide (DMAB, 98%, mol wt. 426.93) were obtained from Sigma-Aldrich (Germany). Chloroform was purchased from Merck (Germany). Bovine Serum Albumin (BSA, mol wt. 66 kDa), ethanol, dichloromethane (DCM) and Brilliant Blue G 250 were obtained from Sigma-Aldrich (Germany). Phosphate-buffered saline (PBS, pH: 7.4) tablets and Tris HCl (10 mM, pH: 7.4) were purchased from Sigma-Aldrich (Germany).
Determination of molar mass of PHBV
The viscosimetric molar mass (Mv) of PHBV was estimated by using an Ubbelohde type viscosimeter. Solutions of PHBV were prepared in chloroform with concentrations varied between 0.4 and 1% (w/v). Flow time of chloroform and solutions were measured to estimate the relative viscosities. The intrinsic viscosity was obtained and applied in the Mark-Houwink equation (CitationBaran et al. 2002).
Preparation of PHBV particles
PHBV particles were prepared by using the emulsification-solvent diffusion method (CitationDinarvand et al. 2011, CitationPalamoor and Jablonski 2014). PHBV (25, 50 and 100 mg) was dissolved in 5 ml of organic solvent at room temperature for 6 h. Chloroform and dichloromethane were used as organic solvents either individually or in combination with ethanol. The organic phase was then added to 10 ml aqueous phase containing either PVA (2, 4, 10% w/v) or DMAB (2, 3, 4% w/v) as stabilizer. The oil-in-water emulsion was emulsified by using a high-speed homogenizer (Heidolph, Germany) at various speeds (10,000, 15,000 and 17,500) and durations (7, 10 and 15 min). Each emulsion was added to either 50 mL of 3 mg mL− 1 PVA or 50 mL of 1 mg mL− 1 DMAB solution to prevent particle agglomeration. The mixture was stirred overnight to allow for diffusion of organic solvent into water. PHBV particles were collected by centrifugation (10,000 rpm for 10 min), washed twice with Tris HCl (pH: 7.4) and freeze-dried (Christ, Germany). The experimental parameters are summarized in .
Table I. Formulations for PHBV particles.
PHBV particles encapsulating model protein BSA were prepared by the double emulsion-solvent diffusion method (CitationCun et al. 2011). Briefly, 100 μl of an aqueous solution containing 10 mg BSA (w1) was emulsified in PHBV solution [o1, 2% (w/v) in DCM] by using a high-speed homogenizer (2,500 rpm, 2 min). The first emulsion was then added into aqueous PVA solution (w2, 10 ml 4%, w/v) to form double emulsion (w1/o1/w2) and emulsified at 17,500 rpm for 15 min (Heidolph, Germany). Subsequently, w1/o/w2 emulsion was added to 50 ml of 3 mg mL− 1 PVA solution. After stirring overnight, BSA encapsulated PHBV particles (PHBV-BSA) were collected by centrifugation (10,000 rpm for 10 min) and freeze-dried at − 80°C (Christ, Germany).
Characterization of PHBV particles
Scanning electron microscopy
The morphology of PHBV particles was characterized by scanning electron microscopy (SEM). The lyophilized powder of particles was mounted on aluminum stumps and sputter coated with gold-palladium prior to examination under SEM (FEI Quanta 400 F, USA). The average diameter of the particles was determined by measuring different points with Image J software (NIH, Bethesda, MD, USA). The diameters were presented as the average ± standard deviation.
Transmission electron microscopy
The morphology of the PHBV and BSA-loaded PHBV particles was examined by transmission electron microscopy (TEM) (FEI Tecnai G2 Spirit, Bio TWIN, USA) operated at an accelerating voltage of 80 kV. Before analysis, the samples were diluted in ethanol (1:3), placed on copper grids and covered with carbon films for observation.
Particle size distribution analysis
The particle size distribution was measured at an angle of 90° at 25° by a dynamic light scattering machine (Zeta Sizer Nano S, Malvern Instruments, UK). Ll measurements were performed in triplicate with particle suspensions diluted 1:100 in distilled water at room temperature.
BSA encapsulation efficiency and release kinetics
BSA entrapment efficiency and release kinetics were determined by using the Bradford Assay (CitationChaudhari et al. 2013). For encapsulation efficiency, the supernatants collected after particle synthesis were analyzed for unloaded amount of protein. The encapsulation efficiency (EE) was then calculated indirectly by using Eq. (1).
In vitro release measurement was performed using 10 mg BSA-loaded particles. The samples were incubated in 2 ml PBS (pH: 7.4) containing 0.1% (w/v) sodium azide at 37°C, 30 rpm shaking medium. At certain time intervals the supernatant was removed and analyzed with the Bradford Assay to determine the amount of protein released. The cumulative release profile was determined in triplicate measurements.
Particle degradation
In situ degradation of BSA-loaded PHBV-BSA particles was examined by SEM analysis. For this purpose, 5 mg of BSA- loaded particles were placed in 1 ml PBS (pH: 7.4) containing 0.1% (w/v) sodium azide at 37°C, 30 rpm shaking medium. At each sampling time (3, 10, 15 and 21 days) the supernatant was removed by centrifugation and the particles were washed twice with distilled water. Subsequently, the particles were lyophilized, sputter coated with gold-palladium and then examined under SEM (FEI, Quanta 400 F, USA).
Results and discussion
The aim of this study was to investigate the effect of various process parameters including PHBV concentration, stabilizer type and concentration, solvent type, and homogenization speed and time, on the production of PHBV particles from pre-formed PHBV polymer. Particle morphology and size were evaluated by using the image processing software, Image J. The effect of process parameters on the mean diameter of particles is summarized in . The viscosimetric molar mass, Mv of PHBV polymer used in these studies was calculated as 138.8 kDa.
Table II. Influence of various parameters on mean diameter of PHBV particles.
Effect of process parameters on PHBV particle formation
Effect of homogenization conditions
Higher homogenization speed and time cause stronger shear stress that leads to formation of smaller emulsion droplets and smaller particle size (CitationGasparini et al. 2008). To investigate the influence of these process parameters on the formation and size of PHBV particles, homogenizer speed and time were varied between to 10,000 and 17,500 rpm and 7 and 15 min respectively, while keeping other parameters constant. No particle formation was observed at a homogenization speed of 10,000 rpm, regardless of the homogenization time. In addition, at a homogenization time of 7 min at all speeds, particle formation could not be detected. shows the morphology of PHBV particles obtained by using different homogenization conditions. When the speed was 15,000 rpm, agglomerated structures were observed at 10 min, indicating that the time was not sufficient for particle formation (). As the homogenization time was increased to 15 min, particles of micron size were obtained at 15,000 rpm (1.57 ± 0.21 μm) (). The shear stress produced at 15,000 rpm homogenization speed was not enough to overcome the viscous forces for production of submicron PHBV particles. The increase in homogenization speed and time resulted in a gradual decrease in particle diameter and submicron PHBV particles were obtained at 17,500 rpm homogenization speed at 10 and 15 min of homogenization time (). The results are summarized in . Similar results have been reported for the production of poly (lactide-co-glycolide) (PLGA) particles. CitationKwon et al. (2001) have found a decrease in particle mean size of PLGA particles correlated with the increase of homogenizer speed up to 12,000 rpm. Above this value, no further decrease was observed. CitationChaisri et al. (2009) have also showed that decreasing the stirring rate from 10,000 to 8,000 rpm caused an increase in particle size of PLGA microspheres.
Figure 1. Effect of homogenization speed and time on particle formation: (a) 15,000 rpm 10 min, (b) 15,000 rpm 15 min, (c) 17,500 rpm 10 min and (d) 17,500 rpm 15 min) [2 % (w/v) PHBV in DCM, 4% PVA (w/v)].
![Figure 1. Effect of homogenization speed and time on particle formation: (a) 15,000 rpm 10 min, (b) 15,000 rpm 15 min, (c) 17,500 rpm 10 min and (d) 17,500 rpm 15 min) [2 % (w/v) PHBV in DCM, 4% PVA (w/v)].](/cms/asset/055d6422-0aa0-43d7-89cd-68c9da67ffbb/ianb_a_937869_f0001_b.gif)
Table III. Effect of homogenization parameters on particle formation.
Effect of polymer concentration
The effect of polymer concentration on particle size has been widely investigated and higher polymer concentrations resulted in an increase in the particle size (CitationKwon et al. 2001, CitationBudhian et al. 2007). This increase was mostly attributed to the increase in viscosity of the organic phase, which in turn causes a resistance to shear forces resulting in larger the size of particles (CitationChaisri et al. 2009, CitationTeng et al. 2010). shows the SEM images of PHBV particles obtained from PHBV-DCM solutions of 4, 2 and 1% (w/v). High polymer concentrations resist droplet breakdown due to high viscosity (CitationMainardes and Evangelista 2005). Besides, during the elimination of organic solvent, dispersion of PHBV solution into aqueous phase was restricted due to viscous interactions. In addition, surfactant molecules were incapable of preventing coalescence in the internal phase of the emulsion with high concentration. As a result, at 4% PHBV concentration, deformed globular agglomerates were observed. When the concentration was decreased to 2% PHBV, it was possible to obtain submicron particles of 531 ± 150 nm (). However, a limiting minimum value of PHBV concentration was obtained for particle formation. For concentrations below 2% PHBV, no particle formation was detected. Coagulated residues of irregular morphology were observed rather than spherical particles ().
Stabilizer type and concentration
Different types of stabilizers such as gelatin, dextran, polyethylene glycol, PVA, DMAB and Pluronic F-68 have been used for particle preparation by the emulsification-diffusion method using different kinds of polymers (CitationAstete and Sabliov 2006, CitationCheow and Hadinoto 2010). In this study, PVA and DMAB were chosen as stabilizers to investigate the stabilizer-type effect on PHBV particle formation. These molecules have been studied widely in the preparation of polyester based particles like PLGA (CitationJaklenec et al. 2008, CitationSahana et al. 2008). Meanwhile, they have different stabilization characteristics. The ionic stabilizer DMAB has a cationic character with a lower critical micelle concentration and prevents the coalescence of emulsion droplets by electrostatic repulsion (CitationSahana et al. 2008, CitationSong et al. 2008). On the other hand, PVA is a non-ionic surfactant and steric hindrance is the main mechanism for stabilization (CitationSahana et al. 2008, CitationSong et al. 2008).
In this study, PHBV particles were successfully synthesized by using DMAB as a stabilizer (). At DMAB concentrations of 2% (w/v), insufficient particle formation was observed in a disordered coagulated structure (). Increasing the DMAB concentration to 3% (w/v) resulted in the formation of spherical and discrete particles in the micrometer size range (1.17 ± 0.22 μm) (). With further increase of DMAB concentration to 4% (w/v), submicron particles with a size of 724 ± 228 nm were synthesized (). When PVA was used as a stabilizer, no particles were detected at 2% (w/v) PVA concentration (). The insufficient amount of PVA failed to stabilize the particles, which resulted in the formation of aggregates. With an increase to 4% (w/v), agglomeration was prevented and spherically shaped particles were obtained in the submicron range (531 ± 150 nm), as shown in . However, at a higher concentration of PVA, the increased viscosity of aqueous phase repressed the net shear stress required for particle formation. As a result, larger particles were formed in the form of coalescent globules at 10% (w/v) PVA concentration ().
Figure 3. Effect of stabilizer concentration and type on particle formation: (a) 2 % (w/v), (b) 3% (w/v), (c) 4% (w/v) DMAB, (d) 2 % (w/v), (e) 4% (w/v) and (f) 10% (w/v) PVA) [2% PHBV (w/v), 17,500 rpm 15 min and solvent type DCM].
![Figure 3. Effect of stabilizer concentration and type on particle formation: (a) 2 % (w/v), (b) 3% (w/v), (c) 4% (w/v) DMAB, (d) 2 % (w/v), (e) 4% (w/v) and (f) 10% (w/v) PVA) [2% PHBV (w/v), 17,500 rpm 15 min and solvent type DCM].](/cms/asset/2cb92916-b688-4029-9b55-7f72cc630296/ianb_a_937869_f0003_b.gif)
In a study by CitationSahana et al. (2008), DMAB and PVA were investigated as stabilizers in the synthesis of PLGA nanoparticles for oral delivery of hydrophobic drugs. Lower particle size was obtained when DMAB was used as a stabilizer, when a combination of DCM and ethyl acetate was used as an organic solvent (CitationSahana et al. 2008). Similar results were also obtained previously by CitationKwon et al. (2001). They have reported DMAB as a mild surface active agent. When propylene carbonate as a partially water soluble solvent was used, the particle size of PLGA prepared using DMAB was smaller than that of PVA. On the other hand, CitationSong et al. (2008) found that when DCM was the organic phase solvent, the mean particle size of PLGA nanoparticles increased in case DMAB was used as a stabilizer, compared to PVA. These different trends in particle size reveal the importance of the type of the organic solvent used, as stated by CitationSong et al. (2008). In our study, when DCM was used as the organic phase solvent, a smaller particle size was obtained with PVA when compared to DMAB. The stabilizer efficiency in terms of reduced particle size obtained in this study was in agreement with the result obtained by CitationSong et al. (2008). PHBV in a polyester structure like PLGA followed the similar trend in particle formation with DMAB and PVA when DCM was the organic solvent.
Solvent type
In the emulsification-solvent diffusion method, the organic phase containing the polymer and the aqueous phase containing the stabilizer are in thermodynamic equilibrium (CitationPoletto et al. 2008). Addition of water destabilizes this equilibrium state and causes the organic solvent to diffuse to the external water phase (CitationPoletto et al. 2008). The transportation of the solvent results in the formation of particles so the size of the particles is dependent on the type of organic solvent. Various studies have been reported on the effect of solvent type for production of the widely studied PLGA particles (CitationBilati et al. 2005, CitationChaisri et al. 2009). CitationSahana et al. (2008) have reported that it was not possible to synthesize stable nano-sized particles when DCM, chloroform, acetone and ethyl acetate were used alone, due to problems of high viscosity, interfacial tension and lack of miscibility with water. Instead, different combinations were used to design an optimum binary solvent. CitationSong et al. (2008) used organic solvents with different water miscibility to study the effect of organic solvent. They showed that when an ionic stabilizer such as DMAB was used, partially water soluble organic solvents were effective in reducing the particle size. In this study, chloroform and DCM having similar interfacial tension values (32.8 dyn/cm for chloroform, 28.3 dyn/cm for DCM) but different water miscibilities (chloroform 1:200, DCM 1:50) were used as the organic phase solvent (CitationWeast 1974). Ethanol was added to both DCM and chloroform for the preparation of solvent combinations, to increase the solubility of the organic phase in water and decrease the interfacial tension (CitationPoletto et al. 2008, CitationEsmaeili et al. 2008). shows the SEM micrographs of PHBV particles obtained by using different organic solvent systems. When DCM and chloroform were used alone, spherical particles were synthesized at a submicron scale. With PVA as a stabilizing agent, the particle sizes were measured as 531 ± 150 nm and 778.93 ± 162 nm for DCM and chloroform respectively (). The reduced size obtained with DCM when compared to chloroform is due to the higher miscibility of DCM with water during the solvent diffusion phase. Faster diffusion of organic solvent to water leads to the formation of smaller particles, as reported by CitationPoletto et al. (2008). Meanwhile, the addition of water-miscible solvents facilitates fast extraction of the solvent to water phase which in turn influences the particle diameter. CitationPoletto et al. (2008) used a binary mixture of organic solvents to prepare nano-sized PHBV particles. To control the interface instability between the organic and aqueous phase, ethanol was used in the solvent mixture. Different ratios of chloroform/ethanol mixtures were used to control the nanoparticle formation. Increased water miscibility in ethanol containing organic phase led to the formation of nanosized particles shown by optical micrographs of primary emulsions (CitationPoletto et al. 2008). In this study, ethanol was added in different ratios to DCM to increase the miscibility of organic solvent with water. When ethanol was used in 70% (v/v), the polymer precipitated as this is a non-solvent for PHBV. At volume fractions of 50:50 and 30:70 (ethanol: DCM) it was possible to obtain clear PHBV solutions. Although faster diffusion profiles were observed with the ethanol-DCM mixture, SEM studies revealed that the particle size increased in solvent combination systems. As can be seen in , the particle size increased to 5.15 ± 0.65 μm and 4.50 ± 0.93 μm for 50:50 and 30:70 combinations, respectively. These results do not correlate with the results obtained by CitationPoletto et al. (2008) for ethanol: chloroform mixtures. In this study, the presence of ethanol increased the diffusion rate of organic solvent to water but the solubility of polymer decreased in the solvent combinations as well. The decreased solubility, or in other words, the increased polymer concentration in the DCM part of the organic phase caused the increase in particle size. The polymer chain entanglement was restricted in the presence of ethanol. It was the polymer solubility dominating the formation of submicron size particles rather than the solvent diffusion rate.
Characterization of optimal particle formation
Taking into account the smallest particle size and narrow size distribution, the preparation of PHBV particles from preformed polymer was realized under the following conditions: 4% (w/v) of PHBV in DCM was used as the organic phase solvent which was emulsified with 2% (w/v) PVA solution by a high speed homogenizator at 17 500 rpm for 15 min. The organic solvent was evaporated overnight in a 50 mL aqueous phase containing 3 mg mL− 1 PVA at 4°C under continuous stirring. The SEM micrographs of particles obtained under these conditions are given in . The image analysis from SEM showed that the particle size was 531 ± 150 nm. The particle size distribution was also analyzed with DLS. shows the distribution of sub-micron particles in the range of 300–400 nm, indicating a narrow size distribution.
BSA loaded PHBV characterization
The double emulsion method has been commonly used for entrapment of proteins and drug molecules in micro or nanoparticles, in which the EE is known to be dependent on chemical structure, molecular weight and hydrophobicity of the polymers (CitationYeo and Park 2004). PHBV nanoparticles have been recently used for encapsulation of paclitaxel and the loading capacity was found to be between 35 and 40% for this hydrophobic drug by CitationVilos et al. (2013). In another study by CitationMasood et al. (2013), high encapsulation of ∼45% was obtained for the hydrophobic Ellipticine anticancer drug. In this study, BSA was used as a model protein to determine the actual protein encapsulation of PHBV particles to investigate their possible use as growth factor carrier. BSA-loaded particles were prepared by the double emulsion-solvent evaporation method. The encapsulation efficiency was determined quantitatively by the Bradford assay and calculated as 21.14 ± 2.20%. The low encapsulation efficiency obtained in this study is attributed to the hydrophobic structure of PHBV which may not be fully compatible with BSA. In a study by CitationYilgor et al. (2010), an encapsulation efficiency of 16.72 ± 1.06% for BSA was obtained with pre-treated low molecular weight PHBV. The increased EE in our study shall be related to the higher molecular weight of PHBV used.
Particle morphology of BSA loaded PHBV
shows the TEM and SEM images of PHBV and BSA-loaded PHBV particles. shows the spherical shape of PHBV particles with a mean diameter of 367 ± 121 nm, consistent with the particle size obtained in DLS measurements. The TEM micrograph of BSA-loaded particles () with different densities show the homogeneous dispersion of BSA inside the spherical particles. When BSA was loaded, the particle diameter increased to 1.85 ± 0.30 μm ().
Particle degradation
The degradation mechanism of polyester based biodegradable polymers is based on a bulk degradation mechanism starting with the absorption of water. The cleavage of ester bonds in the polymer backbone takes place by hydrolysis followed by significant mass loss and mechanical integrity (CitationLao et al. 2011). The release of any bioactive molecule encapsulated in a polyester based particle shall take place by the bulk degradation of the structure. In this study, the bulk degradation of BSA-loaded PHBV particles were analyzed by SEM. show the degradation profile of PHBV particles. The particle shape was still preserved at day 10; meanwhile, loss in integrity and shape were obvious at the end of day 21 ().
In vitro release of BSA
The release profile of the model protein BSA from PHBV particles is given in as cumulative release percentage. Release of the entrapped BSA molecules shall occur through a diffusion-degradation mediated process. A substantial initial burst of 46.18 ± 0.314% was detected and the amount of BSA reached 73.94 ± 1.578% after 3 days. The subsequent release was quite slow and reached around 96.08 ± 0.069% at the end of 15 days. As to the low compatibility of BSA with PHBV, most of the encapsulated protein molecules shall be located near the surface, showing the fast diffusion behavior in the early phase. The dispersed BSA molecules within the particles were probably released by both diffusion and degradation of PHBV at the later phase. The kinetics of BSA release were investigated by fitting the data to various models (CitationPulat et al. 2006) and found to follow the Higuchi model (CitationLao et al. 2011). The Higuchi rate constant, k, was calculated from the early phase data prior to substantial degradation as 0.447 s− 1. During the release studies the pH of the release medium was not lower than pH: 6 and no peak shift from 285 nm was observed in UV measurements. This shall be interpreted to be so because BSA kept its structural properties (CitationEstey et al. 2006).
Conclusions
The effects of various emulsification-diffusion production parameters were evaluated on the formation of PHBV particles from a preformed polymer without any pretreatment. The increase in homogenization speed and time caused a decrease in particle size, as expected. Submicron particles were synthesized successfully when DMAB was used as a cationic stabilizer. However, PVA lead the formation of smaller size particles when DCM was used as a solvent. An optimum polymer concentration was found for the production of submicron sized particle formation. The higher miscibility of organic solvent with water phase was important for obtaining smaller PHBV particles but the use of solvent combinations-including non-solvents for PHBV-to increase miscibility with water resulted in micron-sized particles. Although BSA encapsulation efficiency was low due to the hydrophobic nature of PHBV, the slow degradation profile, low cost and biocompatible structure shall highlight the naturally synthesized PHBV as an alternative carrier for growth factor molecules.
Declaration of interest
The authors report no declarations of interest. The authors alone are responsible for the content and writing of the paper.
This study is supported by Ankara University Research Foundation (Project No: 11B4343006).
References
- Astete CE, Sabliov CM. 2006. Synthesis and characterization of PLGA nanoparticles. J Biomater Sci Polym Ed. 17:247–289.
- Baran ET, Özer N, Hasirci V. 2002. Poly(hydroxybutyrate-co-hydroxyvalerate) nanocapsules as enzyme carriers for cancer therapy: an in vitro study. J Microencapsulation. 19:363–376.
- Bilati U, Alleman E, Doelker E. 2005. Development of a nanoprecipitaion method intended for the entrapment of hydrophilic drugs into nanoparticles. Eur J Pharm Sci. 24:67–75.
- Budhian A, Siegel S, Winey K. 2007. Haloperidol-loaded PLGA nanoparticles: systemic study of particle size and drug content. Int J Pharm. 336:367–375.
- Chaisri W, Hennink WE, Okonogi S. 2009. Preparation and characterization of cephalexin loaded PLGA microspheres. Curr Drug Deliv. 6:69–75.
- Chaudhari A, Vanmellart L, Bauwens M, Vermaelen P, Deroose CM, Naert I, et al. 2013. In vitro and in vivo investigation of the potential of amorphous micro porous silica as a protein delivery vehicle. BioMed Res Int. 2013:10.
- Cheow WS, Hadinoto K. 2010. Enhancing encapsulation efficiency of highly water-soluble antibiotic in poly(lactic-co-glycolid acid) nanoparticles: Modifications of standard nanoparticle preparation methods. Colloids Surf A. 370:79–86.
- Cun D, Jensen DK, Maltesen MJ, Bunker M, Whiteside P, Scurr D, et al. 2011. High loading efficiency and sustained release of siRNA encapsulated in PLGA nanoparticles: quality by design optimization and characterization. Eur J Pharm Biopaharm. 77:26–35.
- Dinarvand R, Sepehri N, Manoochehri S, Rouhani H, Atyabi F. 2011. Polylactide-co-glycolide nanoparticles for controlled delivery of anticancer agents. Int J Nanomedicine. 6:877–895.
- Esmaeili F, Atyabi F, Dinarvand R. 2008. Preparation and characterization of estradiol-loaded PLGA nanoparticles using homogenization-solvent diffusion method. DARU. 26:196–202.
- Estey T, Kang J, Schwendeman P, Carpenter J. 2006. BSA degradation under acidic conditions: a model for protein instability during release from PLGA delivery systems. J Pharm Sci. 95:1626–1639.
- Gasparini G, Kosvintsev SR, Stillwell MT, Holdich RG. 2008. Preparation and characterization of PLGA particles for subcutaneous controlled drug release by membrane emulsification. Colloids Surf B Biointerfaces. 61:199–207.
- Jaklenec A, Hinckfuss A, Bilgen B, Ciombor DM, Aoron R, Mathiowits E. 2008. Sequential release of bioactive IGF-I and TGF-β1 from PLGA microsphere-based scaffolds. Biomaterials. 29:1518–1525.
- Kowalczuk A, Trzcinska R, Trzebicka B, Müller AHE, Dworak A, Tsvetanov CB. 2014. Loading of polymer nanocarriers: Factors, mechanisms and applications. Prog Poly Sci. 39:43–86.
- Kwon H, Lee J, Choi S, Jang Y, Kim J. 2001. Preperation of PLGA nanoparticles containing estrogen by emulsification-diffusion method. Colloids Surf A. 182:123–130.
- Lao LL, Peppas NA, Boey FYC, Venkatraman SS. 2011. Modeling drug release from bulk-degrading polymers. Int J Pharm. 418:28–41.
- Masood F, Chen P, Yasin T, Fatima N, Hasan F, Hameed A. 2013. Encapsulation of Ellipticine in poly-(3-hydroxybutyrate-co-3-hydroxyvalerate) based nanoparticles and its in vitro application. Mater Sci Eng C Mater Biol Appl. 33:1054–1060.
- Mainardes R, Evangelista R. 2005. PLGA nanoparticles containing praziquantel: effect of formulation variables on size distribution. Int J Pharm. 290:137–144.
- Palamoor M, Jablonski MM. 2014. Comparative study on diffusion and evaporation emulsion methods used to load hydrophilic drugs in poly(ortho ester) nanoparticle emulsions. Powder Technol. 253:53–62.
- Pich A, Schiemenz N, Corten C, Adler HJP. 2006. Thermoreversible gelation of biodegradable polyester (PHBV) in toluen. Polymer. 47:1912–1920.
- Poletto FS, Fiel L, Donida B, Re M. 2008. Controlling the size of PHBV nanoparticles prepared by emulsification-diffusion technique using ethanol as surfactant agent. Colloids Surf A. 324:105–112.
- Pulat M, Ekmekçi A, Aslim B. 2006. The release of bovine serum albumin from polyurethane based hydrophilic and hydrophobic disks and microbiological interactions. Bio-Med Mater Eng. 16: 147–156.
- Sahana DK, Mıttal G, Bhardwaj V, Ravi Kumar MN. 2008. PLGA nanoparticles for oral delivery of hydrophobic drugs: influence of organic solvent on nanoparticle formation and release behavior in vitro and in vivo using Estradiol as a model drug. J Pharm Sci. 97:1530–1542.
- Song X, Zhao Y, Hou S, Xu F, Zhao R, He J, et al. 2008. Dual agents loaded PLGA nanoparticles: Systemic study of particle size and drug entrapment efficiency. Eur J Pharm Biopaharm. 69:445–453.
- Teng L, Jiang C, Lin X, Dong Y, Li C, Meng Q, Li Y. 2010. Preperation of tolterodine metabolite loaded biodegradable PLGA microspheres. Chem Res Univ. 26:75–80.
- Vilos C, Morales FA, Solar PA, Herrera NS, Gonzalez-Nilo FD, Aguayo DA, et al. 2013. Paclitaxel-PHBV nanoparticles and their toxicity to endometrial and primary ovarian cancer cells. Biomaterials. 34:4098–4108.
- Vroman I, Tighzert L. 2009. Biodegradable polymers. Materials. 2:307–344.
- Yilgor P, Hasirci N, Hasirci V. 2010. Sequential BMP-2/BMP-7 delivery from polyester nanocapsules. J Biomed Mater Res A. 93: 528–536.
- Vo TN, Kasper FK, Mikos AG. 2012. Strategies for controlled delivery of growth factors and cells for bone regeneration. Adv Drug Deliv Rev. 64:1292–1309.
- Yeo Y, Park K. 2004. Control of encapsulation efficiency and initial burst in polymeric microparticle systems. Arch Pharm Res. 27:1–12.
- Weast RC. 1974. Handbook of Chemistry and Physics. Boca Raton, FL: CRC Press Inc.
- Zhu XH, Wang C, Tong YW. 2009. In-vitro characteriztion of hepatocyte growth factor release from PHBV/PLGA microsphere scaffold. J Biomed Mater Res Part A. 89:411–429.