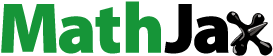
Abstract
The effect of different genetically engineered bacteria, Pseudomonas syringae, Pseudomonas savastanoi, and Ralostonia solanacerum and also a natural marine bacterial species, Vibrio fischeri NRRL B-11177, is studied in producing gold nanoparticles. This is the first report about the biosynthesis of gold nanoparticles by natural and genetically engineered luminescent bacteria. These microorganisms reduced gold ions and produced fairly monodisperse nanoparticles. TEM analysis indicated that spherical nano gold particles in the different diameters and shapes were obtained at pH values of 6.64. In this biosynthesis protocol, the gold nanoparticles with desired shape and size can be prepared.
Introduction
Nanometer-sized colloidal crystals have been explored for diverse applications since their electronic structure and optical and magnetic properties can be fine-tuned by manipulating their physical size. This renders nanoparticles (NPs) ideal materials for bioimaging, therapeutics, catalysis, and optoelectronics (CitationXia et al. 2009, CitationLee et al. 2010, CitationPiao et al. 2008, CitationEshghi et al. 2013b). However, their synthesis requires sophisticated skills, intuition, and extensive experimentation in order to obtain high-quality NPs. In addition, current synthesis methods of NPs require organic solvents or toxic chemicals, which potentially cause environmental problems (CitationHussain et al. 2005, CitationEshghi et al. 2013a). Therefore the development of reliable, nontoxic, and ecofriendly methods for synthesis of nanoparticles is of utmost importance to expand their biomedical applications. One of the options to achieve this goal is to use organisms ranging from bacteria to fungi and even plants to synthesize nanoparticles (CitationBhattacharya and Rajinder 2005, CitationMohammed Fayaza et al. 2011). Biological entities and inorganic materials have been in constant touch with each other ever since inception of life on the earth. Due to this regular interaction, life could sustain on this planet with a well-organized deposit of minerals. The biosynthesized nanoparticles have been used in a variety of applications including drug carriers for targeted delivery, cancer treatment, gene therapy and DNA analysis, antibacterial agents, biosensors, and magnetic resonance imaging (MRI) (CitationLi et al. 2011). Microorganisms have been exposed to a variety of pollutants in the environment (including water and soil). Metal ion, being nonbiodegradable and persistent in nature often causes toxicity and inhibits microbial growth. However, even at high metal ion concentration microorganisms can survive and grow due to their ability to fight the metal stress (CitationBhainsa and D’Souza 2006). These metal-microbe interactions have an important role in several biotechnological applications.
The “biogenic” approach for production of nanoparticles is supported by the fact that the majority of the bacteria inhabit ambient conditions of varying temperature, pH, and pressure. The particles generated by these processes have higher catalytic reactivity, greater specific surface area, and an improved contact between the enzyme and metal salt in question due to the bacterial carrier matrix (CitationBhattacharya and Mukherjee 2008, CitationSimkiss and Wilbur 1989). With an enzyme process, the use of expensive chemicals is eliminated, and the more acceptable “green” route is not as energy intensive as the chemical method and is also environmentally friendly.
Genetically modified bacteria were the first organisms to be modified in the laboratory, due to their simple genetics. These microorganisms are now used for several purposes, and are particularly important in producing large amounts of various compounds for medical applications especially pure human proteins. Recently, various types of metal NPs were synthesized by using microfluidic systems or bioengineered cells to overcome the conventional method to fabricate nanomaterials (CitationWhitesides 2006, CitationTeh et al. 2008). However, in vivo synthetic methods are to meet the objectives of improved size-selectivity, tighter control over particle properties, and better batch-to-batch reproducibility. Recently Park et al. have demonstrated the in vivo biosynthesis of diverse NPs including semiconductors, magnetic crystals, and noble metals, by constructing recombinant bacteria, Escherichia coli (E. coli) strains expressing phytochelatin synthase and metallothionein (CitationDischer and Eisenberg 2002, CitationTanaka and Sackmann 2005, CitationPark et al. 2010).
Nonspecific (metabolic) bioluminescent microbial biosensors encompass broad categories, like naturally luminescent, Vibrio fisheri NRRL B-11177 (V. fisheri NRRL B-11177) and genetically modified, Pseudomonas syringae, Pseudomonas savastanoi, and Ralostonia solanacerum which are used in this study. Hence, in the present work we have investigated biosynthesis of Au nanoparticles using wild type and genetically modified luminescent bacteria. The study includes biosynthesis, spectroscopic studies, and microscopic characterization of the Au nanoparticles. It has been demonstrated that these microorganisms can act as a potential biofactory for synthesis of gold nanoparticles.
Experimental
Materials
Gold chloride trihydrate (HAuCl4.3H2O) was purchased from Aldrich and used without any purification. Other chemical compounds used in this research were purchased from Merck. All aqueous solutions were prepared from Millipore water (resistivity, 18 MX; Millipore Systems). UV-visible absorption spectroscopic measurements were recorded on a UV-vis spectrometer, Shimadzu UV1700, using quartz cells of 1 cm path length. Transmission Electron Microscopic (TEM) images of the nanoparticles were taken with a LEO 912AB instrument operated at an accelerating voltage of 120 kV with line resolution of 0.3 nm at room temperature. The samples for TEM measurements were prepared by placing a droplet of the colloidal solution onto a carbon-coated copper grid and allowing it to dry in the air naturally. Based on the TEM images we determined the size distributions of the final product by counting at least 300 particles. Scanning electron microscopy (SEM) images were recorded by using a LEO-1530VP field-emission scanning electron microscope with an accelerating voltage of 5.0 kV. The FT-IR spectra were obtained on an Avatar 370 FT-IR Therma Nicolet spectrometer. Luminescence of bacteria was measured by a luminometer (Berthold FB12).
P. syringae, P. savastanoi, and R. solanacerum are of the traditional plant pathogens which have many phytopathological properties. V. fischeri is a gram-negative rod-shaped bacterium found globally in marine environments.
Bacterial strains and culture medium
V. fischeri has bioluminescent properties, and is found predominantly in symbiosis with various marine animals. The bacterium is a key research organism for examination of microbial bioluminescence, quorum sensing, and bacterial-animal symbiosis. V. fischeri NRRL B-11177 were cultured in the SWC medium containing 3 ml glycerol, 5 g peptone, 3 g yeast extract, and 24 g sea salt in 1 l distilled water at pH 7.3. The bacteria were incubated at 18–22°C for 72 h and separated from the broth by centrifugation (5000 rpm) for 10 min.
P. syringae is a rod shaped, gram-negative bacterium with polar flagella. It is a plant pathogen which can infect a wide range of plant species, and exists as over 50 different pathovars. P. syringae has come to represent an important model system for experimental characterization of the molecular dynamics of plant-pathogen interactions. P. savastanoi is a gram-negative plant pathogenic bacterium that infects a variety of plants. R. solanacerum is an aerobic non-sporing, gram-negative plant pathogenic bacterium. R. solanacerum is soil-borne and motile with a polar flagella tuft. It colonizes the xylem, causing bacterial wilt in a very wide range of potential host plants.
Strains P. savastonoi P48, P. syringae, and R. solanacerum were genetically lux-marked using miniTn-5 luxAB transposon. Purified strains were transformed with plasmid pUT containing luxAB gene using an electroporator (Eppendorf electroporator2510). Electroporation of above strains was performed as following settings: voltage 2.5 KV for 5 ms. All luxAB marked strains could grow on KB agar medium containing 50 microgram per milliliter tetracycline and their luminescence was measured by a luminometer (Berthold FB12).
Biological synthesis of gold nanoparticles by microorganisms
Studies have found that many microorganisms can produce inorganic nanoparticles through either intracellular or extracellular routes. This section describes the production of gold nanoparticles via biological method.
In this study, AuNPs were synthesized using a reduction of aqueous Au3+ with the culture supernatants of four different bacteria at room temperature. The collected bacteria were washed five times with distilled water to obtain about 1 g wet weight of bacteria and then resuspended in 20 ml of 1 × 10− 3 M aqueous HAuCl4 solution in an Erlenmeyer flask. The reactants were adjusted to neutral pH using 0.1 M NaOH solution. In this experiment, the pH of the solution was adjusted to 6–7. All the experiments were conducted at room temperature on a rotary shaker (120 rpm) for a period of 48 h.
Results and discussion
Characterization of AuNPs
Recently scientists have become more and more interested in the interaction between inorganic molecules and biological species. In this present study, we tried to biosynthesis of AuNPs using a reduction of aqueous Au3+ with the culture supernatants of four different bacteria; V. fischeri is a bioluminescent bacterium and P. syringae, P. savastanoi, R. solanacerum are from the traditional plant pathogens. For this purpose, firstly, strains P. savastonoi P48,. P syringae, and R. solanacerum were genetically lux-marked. Then a weight of these collected wild and genetically modified bacteria were suspended in aqueous HAuCl4 solution and the pH of the solution was adjusted to neutral pH. The aqueous chloroaurate ions were reduced during exposure to bacteria biomass. The color of the reaction solution turned from pale yellow to red, which indicated the formation of gold nanoparticles. The change in color of the medium was noted by visual observation.
The color of the reaction solution remained red without any changes, and the gold nanoparticles were stable after 48 h of reaction. Control experiments without biomass addition stayed pale yellow, indicating that the production of gold nanoparticles is obtained by the microorganism reduction indeed. Moreover, the color change was also shown in .
The application of AuNPs was highly dependent on the chemical composition, shape, size, and monodispersity of particles. To broaden the application scope, the AuNPs obtained were systematically characterized using TEM and UV-Vis analysis.
shows the UV–Vis absorption spectra recorded from the gold nanoparticles solution after the reaction. The results indicate that the reaction mixture has an absorption maximum at about 540 nm attributed to the surface plasmon resonance band (SPR) of the gold nanoparticles. The plasmon band of gold nanoparticles synthesized by R. solanacerum, V. fischeri bacterial species and P. syringae and P. savastanoi is observed at 527, 533, 535, and 552 nm, respectively. These results indicated that the reduction of AuCl4− was caused by these bacteria to produce the gold nanoparticles. The peak intensity and position of the surface plasmon absorption band are dependent on the size and shape of the metal nanoparticles, the dielectric constant of the metals as well as the medium surrounding the particles (CitationLink and El-Sayed 2000). An intense absorption peak at 517 nm is generally attributed to the surface plasmon excitation of small spherical gold particles (CitationAbdelhalim et al. 2011). When the GNP size increases, the maximum extinction of Surface Plasmon Band (SPB) is red shifted slightly in the visible region which may be attributed to the surface plasmon oscillation of free electrons. However, it can be affected by four factors: density of electrons, electron mass, mass and size of the charge of the nanoparticles.
Figure 2. UV-Vis spectra of the prepared gold nanoparticles dispersed in water in the presence of four different bacteria.
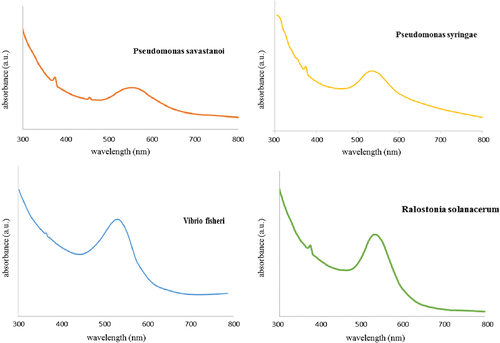
On the other hand, when the shape of the nanoparticles changes from nanosphere to nanorods, the surface plasmon absorption spectrum also changes (CitationLink et al. 1999). The surface plasmon absorption of gold nanorods has two bands: a strong long wavelength band in the near infrared region due to the longitudinal oscillation of electrons and a weak short wavelength band in the visible region around 520 nm due to the transverse electronic oscillation.
These nanoparticles present in the aqueous medium were quite stable, even up to 4 months of incubation at room temperature. This is an important aspect of synthesis of nanoparticles, since the lack of sufficient stability of many nanoparticles preparation has to some extent impeded the development of the real world applications of nanomaterials.
The result obtained from the TEM study () gives a clear indication regarding the shape and size of the nanoparticles.
As shown in , well-separated gold nanoparticles are mainly spherical in different diameters in sizes 15, 20, 30, 40 nm at neutral pH in the presence of R. solanacerum, V. fischeri bacterial species and P. syringae and P. savastanoi, respectively. Such variation in shape and size of nanoparticles synthesized by biological systems is common. This result was consistent with the UV-Vis study.
For further characterization of these prepared gold nanoparticles and the medium around the particles, and for further studies on the mechanism and confirming if a possible internalization of gold-nanoparticles occurs, we chose the reaction accomplished in the presence of R. solanacerum bacteria.
indicates the TEM images of gold nanoparticles prepared by this method in the presence of R. solanacerum bacteria. These images were obtained from the wide area of a sample consisting of gold nanoparticles on the bacteria surface with a relatively low magnification. In other words, these images indicated a mass of the bacterial composition around gold nanoparticles. As shown, the particles do not get self-assembled because the natural compound acting as a capping agent.
Figure 4. Low-magnification TEM images of a sample consisting of gold nanoparticles on R. solanacerum bacteria surface.
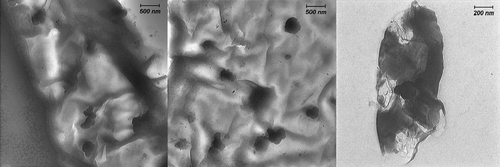
shows typical SEM images of the resulting nanoparticles. The image indicates that the precipitates consist of a mass of nanoparticles on the bacteria surfaces.
Figure 5. SEM images of the resulting nanoparticles in the presence R. solanacerum bacteria. The scale bars are 10 and 2 μm.
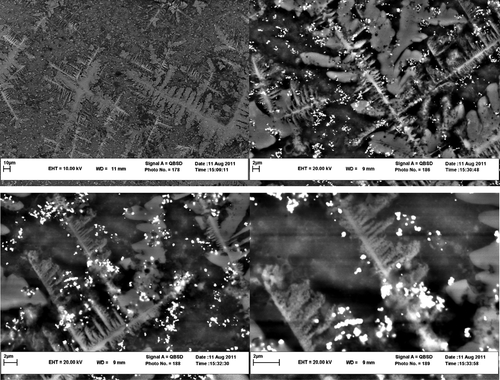
shows the FTIR spectrum of the dried powder of gold nanoparticles formed after 48 h of incubation with the R. solanacerum bacteria supernatant. The band seen at 3448 cm−1 and 2908 cm−1 were assigned to the stretching vibration of primary and secondary amines, respectively. The bands at 1629 cm−1 correspond to the stretch molecule vibration. The band existing at 1057 cm−1 can be assigned to the C–N stretching vibrations of amines. This FTIR spectrum supports the presence of proteins in the synthesis of gold nanoparticles. These proteins might be responsible for the formation of the nanoparticles and may play an important role in the stabilization of the formed nanoparticles.
Bioluminescent activity of V. fisheri
The light intensity was measured for a mixture of 450 μl of an aqueous solution of V. fisheri and 50 μl of biosensor using a luminometer before and after of the reaction. Results showed sharp decrease in light output in the way that luminescence decreased from 538859 RLU/s for 0.001 g/ml aqueous solution of V. fisheri before the reaction to 145 RLU/s after the reaction. It showed significant decrease in bioluminescent activity.
Investigation of the key factors of reaction
Bacteria have shown the ability to reduce metal ions to form metallic nanoparticles. The key factors in the supernatant of the bacteria govern the formation of AuNPs. Previous studies indicated that NADH might be a key factor for the synthesis of AuNPs by bacteria. But it is confirmed that NADH alone was not sufficient for the reaction to occur, and other active substances with higher molecular weight might also play important roles in the reduction of Au ions. In organisms, NADH is a widespread reduced coenzyme involved in redox reaction, and can be used as a reducing agent by many enzymes in vivo. Thus, a NADH-dependent reductase released by bacteria might account for the synthesis of AuNPs. In the process, NADH acted as an electron carrier, and the Au ions obtained electrons from NADH via the NADH-dependent reductase, and then were reduced to Au (). Also, there were other reports that NADH-dependent reductases were believed to be involved in the biosynthesis of AuNPs in Fusarium oxysporum and Penicillium fellutanum. It is indicated that secretion of some protein components into the medium by the biomass might play important role in the reduction of the metal ions to the form of nanoparticles. Consequently the proteins also may bind to the nanoparticles and enhance the stability.
The lux operon is a set of genes in luminescent bacteria. The lux operon is a 9-kilobase fragment that controls bioluminescence through the catalyzation of the enzyme luciferase. The lux operon has a known gene sequence of luxCDAB(F)E, where lux A and lux B are codes for the components of luciferase, and the lux CDE codes are for a fatty acid reductase complex that makes the fatty acids necessary for the luciferase mechanism. Lux C codes for the enzyme acyl-reductase, lux D codes for acyl-transferase, and lux E makes the proteins needed for the enzyme acyl-protein synthetase. Luciferase produces blue/green light through the oxidation of reduced flavin mononucleotide and a long-chain aldehyde by diatomic oxygen:
In order to generate the aldehyde needed in the reaction above, three additional enzymes are needed. The fatty acids needed for the reaction are pulled out from the fatty acid biosynthesis pathway by the enzyme acyl-transferase. Acyl-transferase reacts with acyl-ACP to release R–COOH, a free fatty acid. R–COOH is reduced by a two-enzyme system to an aldehyde:
Therefore luminescent bacteria that have cofactor NADH- and NADH dependent enzymes, is responsible for the reduction of Au (3+) to Au (0) and the subsequent formation of gold nanoparticles. On the other hand, our previous research assessed the effects of different concentrations of water and ethanolic extracts of two native Khorasan medicinal plants using a biosensor which can confirm the reducing property of these bacteria. In that work, we found that various concentrations of these extracts had more significant effects on bioluminescent activity and an enhanced resazurin reduction significantly. (Resazurin is used mainly as an oxidation-reduction indicator in cell viability assays for bacteria and mammalian cells).
In conclusion, it has been demonstrated that the luminescent bacteria is capable of producing gold nanoparticles and the gold nanoparticles are quite stable in solution. This is an efficient, ecofriendly and simple process for producing gold nanoparticles. But the exact mechanism of the reduction of gold ions is to be elucidated for these bacteria.
Conclusion
We demonstrated the in vitro synthesis of gold NPs using three different genetically engineered bacteria; P. syringae, P. savastanoi, and R. solanacerum and also a natural marine bacterial species: V. fischeri NRRL B-11177 that served as individual biofactories. We believe that this biomimetics is a powerful method for the biosynthesis of various metal NPs with the use of cellular behavior and a metal growing mechanism. In this study, AuNPs were synthesized by bacteria at room temperature. The AuNPs were quite stable without using any toxic chemicals as capping agents. The spherical AuNPs ranged in different diameter sizes as 15, 20, 30, 40 nm at neutral pH in the presence of R. solanacerum, V. fischeri bacterial species and P. syringae and P. savastanoi, respectively. NADH and NADH-dependent reductase were probably the key factors for the biosynthesis of AuNPs. The ability to synthesize AuNPs as potential anti-microbial agents using bacteria are highly promising for the green, sustainable production of nanometals, and also enhances its widespread application as an important strategy. However, development of simple and ecofriendly synthetic route would help promoting further interest in the synthesis and application of metallic nanoparticles.
Declaration of interest
The authors report no declarations of interest. The authors alone are responsible for the content and writing of the paper.
We are thankful to the Ferdowsi University Research Council for the financial support of this work (Grant P 53:24-01-89).
References
- Abdelhalim MAK, Mady MM, Ghannam MM, Al-Ayed MS, Alhomida AS. 2011. The effects of gold nanoparticles size and concentration on viscosity, flow activation energy, dielectric and optical properties. Afr J Biotechnol. 10:13121–13127.
- Bhainsa KC, D’Souza SF. 2006. Extracellular biosynthesis of silver nanoparticles using the fungus Aspergillus fumigatus. Colloids Surf B. 47:160–164.
- Bhattacharya D, Rajinder G. 2005. Nanotechnology and potential of microorganisms. Crit Rev Biotechnol. 25:199–204.
- Bhattacharya R, Mukherjee P. 2008. Biological properties of “naked” metal nanoparticles. Adv Drug Delivery Rev. 60:1289–1306.
- Discher DE, Eisenberg A. 2002. Polymer vesicles. Science. 297:967–973.
- Eshghi H, Rahimizadeh M, Attaran N, Bakavoli M. 2013a. Dipyrromethane as a new organic reagent for the synthesis of gold nanoparticles: preparation and application. J Iran Chem Soc. 10:1151–1157.
- Eshghi H, Sazgarnia A, Rahimizadeh M, Attaran N, Bakavoli M, Soudmand S. 2013b. Protoporphyrin IX-gold nanoparticle conjugates as an efficient photosensitizer in cervical cancer therapy. Photodiagnosis Photodyn Ther. 10:304–312.
- Hussain I, Graham S, Wang Z, Tan B, Sherrington DC, Rannard SP, et al. 2005. Size-controlled synthesis of near-monodisperse gold nanoparticles in the 1–4 nm range using polymeric stabilizers. J Am Chem Soc. 127:16398–16399.
- Lee KG, Wi R, Park TJ, Yoon SH, Lee JB, Lee SJ, Kim DH. 2010. Synthesis and characterization of gold-deposited red, green and blue fluorescent silica nanoparticles for biosensor application. Chem Commun. 46:6374–6376.
- Li X, Xu H, Chen ZS, Chen G. 2011. Biosynthesis of nanoparticles by microorganisms and their applications. J Nanomater. 2011:1–16.
- Link S, El-Sayed MA. 2000. Shape and size dependence of radiative, non-radiative and photothermal properties of gold nanocrystals. Int Rev Phys Chem. 19:409–453.
- Link S, Mohamed MB, El-Sayed MA. 1999. Simulation of the optical absorption spectra of gold nanorods as a function of their aspect ratio and the effect of the medium dielectric constant. J Phys Chem B. 103:3073–3077.
- Mohammed Fayaza A, Girilal M, Rahman M, Venkatesan R, Kalaichelvan PT. 2011. Biosynthesis of silver and gold nanoparticles using thermophilic bacterium Geobacillus stearothermophilus. Process Biochem. 46:1958–1962.
- Park TJ, Lee SY, Heo NS, Seo TS. 2010. In vivo synthesis of diverse metal nanoparticles by recombinant Escherichia coli. Angew Chem Int Ed. 49:7019–7024.
- Piao Y, Kim J, Na HB, Kim D, Baek JS, Ko MK, et al. 2008. Wrap-bake-peel process for nanostructural transformation from beta-FeOOH nanorods to biocompatible iron oxide nanocapsules. Nat Mater. 7:242–247.
- Simkiss K, Wilbur KM. 1989. Biomineralization. Cell Biology and Mineral Deposition. New York, NY: Academic Press.
- Tanaka M, Sackmann E. 2005. Polymer-supported membranes as models of the cell surface. Nature. 437:656–663.
- Teh SY, Lin R, Hung L, Lee AP. 2008. Droplet microfluidics. Lab Chip. 8:198–220.
- Whitesides GM. 2006. The origins and the future of microfluidics. Nature. 442:368–373.
- Xia Y, Xiong Y, Lim B, Skrabalak SE. 2009. Shape-controlled synthesis of metal nanocrystals: simple chemistry meets complex physics. Angew Chem Int Ed. 48:60–103.