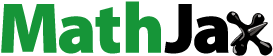
Abstract
Objectives
The repairing of large segmental bone defects is difficult for clinical orthopedists at present. Gene therapy is regarded as a promising method for bone defects repair. The present study aimed to construct an effective and controllable Tet-On expression system for transferring hBMP-2 gene into bone marrow mesenchymal progenitor cells (BMSCs). Meanwhile, with combination of alginate-poly-L-lysine-alginate (APA) microencapsulation technology, we attempted to reduce the influence of immunologic rejection and examine the effect of the Tet-On expression system on osteogenesis of BMSCs.
Methods
The adenovirus encoding hBMP-2 (ADV-hBMP2) was constructed using the means of molecular cloning. The ADV-hBMP2 and Adeno-X Tet-On virus was respectively transfected to the HEK293 for amplification and afterward BMSCs were co-infected with the virus of ADV-hBMP2 and the Adeno-X Tet-On. The expression of hBMP-2 was measured with induction by doxycycline (DOX) at different concentration by means of RT-PCR and ELISA. Combining Tet-On expression system and APA microcapsules with the use of the pulsed high-voltage electrostatic microcapsule instrument, we examined the expression level of hBMP-2 in APA microcapsules by ELISA as well as the osteogenesis by alizarin red S staining.
Key findings
An effective Tet-On expression system for transferring hBMP-2 gene into BMSCs was constructed successfully. Also, the expression of hBMP-2 could be regulated by concentration of DOX. The data exhibited that BMSCs in APA microcapsules maintained the capability of proliferation and differentiation. The combination of Tet-On expression system and APA microcapsules could promote the osteogenesis of BMSCs.
Conclusions
According to the results, microencapsulated Tet-On expression system showed the effective characteristics of secreting hBMP-2 and enhancing osteogenesis, which would provide a promising way for bone repair.
Introduction
Traditional methods for treatment of nonunion or bone defect include bone transplantation of autologous and allograft. However, each method has some limitations to fully meet clinical needs (CitationShegarfi and Reikeras 2009). In recent years, with the development of gene therapy and tissue engineering technology, it has become an investigative hot spot to repair bone defect using gene therapy or gene-enhanced tissue engineered bone (CitationHidaka et al. 2003, CitationYoung et al. 2002, CitationKhaled et al. 2011).
Among various osteogenic factors, bone morphogenetic proteins (BMPs) which belong to the TGF-β superfamily have been widely applied to bone defect via gene therapy and their function of the osteogenesis is most clear. Their repair function for bone defects had been proven in rabbits, mice, sheep, and dogs (CitationSchmoekel et al. 2005, CitationMurakami et al. 2003, CitationWang et al. 2010, CitationMaus et al. 2008, CitationHan et al. 2013). BMPs have powerful capability to stimulate osteogenesis and are important regulators during the formation of bone as well. Among various growth factors, BMP-2 was acknowledged as an effective osteogenesis-induced factor (CitationZhang et al. 2013, CitationQuarto et al. 2012, CitationFan et al. 2013, CitationMladenov et al. 2010). However, the limited ability of BMP-2 secretion by the body could not meet the need for bone repair and the properties of exogenous BMP-2 such as short pharmacological half-life and easy degradation by protease would greatly attenuate the effect of osteoinduction. Therefore, it would be crucial to effectively supply sufficient and sustained endogenous BMP-2 by means of gene delivery. To fulfill this task, adenovirus would be an ideal candidate as its advantages like broad host range, relatively low pathogenicity and high transfection efficiency (CitationYao et al. 2010). Nevertheless, concerns regarding their immunogenicity still remain. Micro-encapsulated cell technique has been proven as an effective method to reduce the immunologic rejection. Chang first proposed the conception of semi-permeable microcapsules in 1964. Since then, this technology has been applied in more and more studies (CitationLim and Sun 1980, CitationHernandez-Valdepena et al. 2005, CitationHortelano 2010, CitationCruz et al. 2010). Microencapsulation is regarded as a protective delivery technology using a biocompatible permselective hydrogel, such as alginate-poly-L-lysine-alginate (APA). It would prevent the detection of host immune system but allow diffusion of nutrients and the therapeutic gene products with significantly less loss or damage (CitationBarsoum et al. 2003, CitationTai and Sun 1993, CitationSantos et al. 2012).
On the other hand, a strong constitutive promoter used in many vectors to drive the expression of the transgene may not reflect physiological expression levels and would, in fact, be harmful to the cells. Progress toward the clinical use of adenovirus vectors for gene therapy will require the ability to regulate the expression of the transgene for improved safety and efficacy. An ideal gene therapy vector should contain a regulatory system which can exhibit tight regulation and allow for rapid and repeatable induction in response to a clinically approved inducer molecule. The tetracycline-dependent transcriptional regulatory system is one of the best studied systems with proven efficacy in vitro and in vivo (CitationGossen and Bujard 1992, CitationGafni et al. 2004).
In this study, we attempted to construct an effective Tet-On expression system for transferring hBMP-2 gene into bone marrow mesenchymal progenitor cells (BMSCs), and investigate the feasibility of the BMP-2 regulation by doxycycline (DOX) in APA microcapsules. We hope that this would provide preliminary support for building engineered bone and open up a new platform for bone repair of large defects.
Materials and methods
Isolation and culture of rat BMSCs
The SD rat was anesthetized with CO2 inhalation and then euthanized with cervical dislocation. The femur was dissected followed by collection of bone marrow with a 10-ml syringe containing growth medium (DMEM/FM12 and 10% FBS) (Gibco, USA) from the bone marrow cavity. Then the cells were harvested using centrifuge at 1000 rpm for 5 min. Then the cells were resuspended with 6ml of growth medium and 4ml of Percoll separating solution (GE, USA). After centrifugation, the cells were transferred carefully from the surface of Percoll and washed with growth medium. Then the cells were collected by centrifuging and cultured in growth medium at 37°C under 5% CO2. The medium was changed every 3 days. When about 85% confluence subculture was performed. Cells at passage 3 were used in this study.
For osteogenic differentiation study, BMSCs with a specific treatment were cultured in osteogenesis medium, which contained high-glucose Dulbecco's modified Eagle medium (H-DMEM), supplemented with 100 nM dexamethasone (Sigma–Aldrich), 50 μg/mL ascorbic acid 2-phosphate (Sigma–Aldrich), and 10 mM β-glycerophosphate (Promega, USA), for 14 days.
Construction of recombinant adenovirus encoding hBMP-2 (ADV-hBMP2)
The whole process was carried out according to the user manual of Adeno-X™ Expression System 1. Briefly, the target gene hBMP-2 was obtained from pcDNA3.1-hBMP-2 plasmid DNA (supplied by Peking Union Medical College) using restriction enzyme XbaI (New England Biolabs). Afterward, hBMP-2 full length ORF was transferred to the pTRE-Shuttle2 plasmids (Clontech, USA) which were digested with XbaI. The linear recombinant pTRE-shuttle2-hBMP2 was obtained after digestion with restriction enzyme PI-Sce I and I-Ceu I (New England Biolabs) simultaneously. Then the digested product was cloned to BD Adeno-X System 1 Viral DNA (Clontech, USA). Recombinants were isolated and amplified by transforming DH10B E. coli cells. After that, the plasmids of recombinant adenoviral vector were digested with PI-Sce I and I-Ceu I enzyme or PCR (the primers were provided with the Adeno-X™ Expression System 1 kit), respectively to confirm whether the recombinants were constructed successfully. Then the adenoviral vector plasmids were linearized by digestion with Pac I. Then HEK 293 cells were transfected with Pac I-digested adenoviral vector complex using Lipofectamine™ 2000 (Invitrogen). After the appearance of CPE, recombinant ADV-hBMP2 was collected by lysing cells with three consecutive freeze-thaw cycles. The ADV-hBMP2 could be amplified with repeated transfection of HEK 293 cells. Last, the ADV-hBMP2 was purified before use.
BD Adeno-X Tet-On virus Stock was purchased from Clontech directly and amplified with repeated transfection of HEK 293 cells.
RNA isolation and RT-PCR analysis
Total RNA from monolayer culture was isolated using TRIZOL® Reagent (Promega, USA) following the manufacturer's instruction. The PCR reactions were performed using Taq Polymerase (TaKaRa, Japan) and the amplification products were visualized on 1.2% agarose gels with ethidium bromide (EB) staining. The primer for hBMP-2 was shown as follows:
The PCR was performed for 30 cycles by the following settings: denature for 30 s at 95°C, anneal for 30 s at 56°C, and extend for 45 s at 72°C, and finally extended at 72°C for 10 min. The target band was 278bp.
Co-transfection of rat BMSCs with ADV-hBMP2 and Adeno-X Tet-On
BMSCs were seeded into 24-well culture plates at a density of 3 × 104 cells per well 12–24 h before infection. Then, the cells in individual wells were incubated in 1 mL of serum-free DMEM with MOI 80 (defined as pfu per cell) of ADV-hBMP2 and Adeno-X Tet-On simultaneously in a cell culture incubator.
Quantitative analysis of BMP-2 expression by specific ELISA
Four hours after co-transfection of rat BMSCs with ADV-hBMP2 and Adeno-X Tet-On, the culture fluids were replaced with 3 mL of fresh DMEM with supplement of 2% FBS and DOX (Sigma–Aldrich) at the following concentration: 0, 10−4, 10−3, 10−2, 10−1, 1, 10 μg/mL. Following additional 48 h incubation, the culture medium was collected to evaluate the expression level of BMP-2 by means of ELISA kit (R&D) according the manufacture's manual.
APA micro-encapsulation of BMSCs
Uninfected or co-transfected BMSCs (1 × 106cells/mL) were collected and resuspended in a 1.5% (w/v) sterilized sodium alginate solution. The cell suspension was extruded through an encapsulator (settings: voltage at 3.0 × 104 V, speed 30 mm/h) into a 100 mM calcium chloride solution to produce calcium alginate gel beads. Then the produced gel beads were kept in 0.05% (w/v) poly-l-lysine (Sciencell, USA) to form alginate–poly-l-lysine membrane. Afterward, the beads were added into 0.15% (w/v) alginate to balance the excess charge around the surface of beads. In the end, the gel beads were further applied in 55 mM sodium citrate to dissolve the alginate gel core. The final microcapsules with cells were cultured in cell incubator for 14 days. Cell number was determined by FDA/EB staining. 100 μL of microcapsule solution was harvested and transferred to each well in a 24-well plate and cell number per well was approximately 3 × 104. Then FDA/EB solution was added to each well at day 0, 1, 2, 4, 6, 10, 14, respectively to measure fluorescence intensity using fluorescence spectrophotometer at △λ = 40 nm. Two peak values were obtained at the spectrum range of 500–650 nm. There, the peak value at 530nm represented living cells and the peak value at 590nm represented dead cells. Three samples for each time point were performed.
To evaluate the influence of APA microencapsulation on the osteogenic ability of BMSCs, the cells were recovered from the microspheres after day 14. Briefly, the samples were centrifuged at 2000 rpm for 10 min and the cell pellet was washed with PBS twice and harvested for osteogenic differentiation study described in previous part.
Alizarin red S staining
Alizarin red S (Sigma–Aldrich, USA) staining was performed for calcium deposition. After 7 days of culture in osteogenesis medium, medium was removed and cells were rinsed three times with PBS and then fixed in 4% paraformaldehyde for 30 min at room temperature. After three washes with deionized water, the sample was stained with 40 mM Alizarin red in deionized water for 10 min at room temperature. Finally, cells were washed three times with deionized water followed by observation under microscope.
Statistical analysis
Each experiment was performed 3 times (n = 3 each), and the results are indicated as mean ± SD. Comparisons between the induced and uninduced groups were made using the t-test. Where appropriate, x2-test was used to analyze results of the viability of BMSCs in APA microcapsules and difference was considered to be statistically significant at P < 0.05. Statistical evaluations were performed using SPSS 13.0 software.
Results
Construction of Tet-On expression system
To determine whether the recombinant adenoviral vectors were constructed successfully, RT-PCR was performed using the Adeno-X forward PCR primer and reverse PCR primer for the recombinants. These primers specifically amplified a 287-bp sequence that spanned the I-Ceu I ligation site in pAdeno-X. The result shown in indicated that pTRE-Shuttle2-derived expression cassettes were cloned into the recombinants successfully. Also, the plasmids of recombinant adenoviral vector were digested with enzyme PI-Sce I and I-Ceu I. As shown in , both of the expected bands including 32.7 kb and 2828 bp appeared in the samples indicating successful construction of recombinant adenoviral vector. Additionally, the sequencing result from 1st BASE confirmed the success of construction of recombinant adenoviral vectors.
Figure 1. Gel electrophoresis images. (A) PCR was performed using the Adeno-X forward PCR primer and reverse PCR primer; (B) Plasmids of recombinant adenoviral vector were digested with enzyme PI-Sce I and I-Ceu I followed by electrophoresis on agarose gel. 1-DNA ladder; 2,3,4-the products of digestion; (C) After co-transfection of BMSCs, the expression of BMP-2 induced by DOX, was verified by RT-PCR. 1-DNA ladder; 2-BMP-2; 3-β-actin.
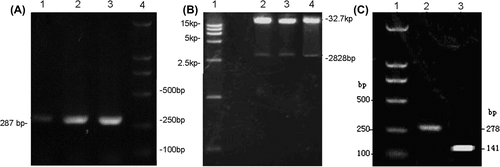
After amplification of ADV-hBMP2 and Adeno-X Tet-On, BMSCs were co-transfected with these two adenoviruses as described in Methods section. Four hours after co-transfection, the culture fluids were replaced with 3 mL of fresh DMEM with supplement of 2% FBS and 10 μg/mL DOX. Then total RNA was extracted from the cells followed by RT-PCR. It was shown in that the expression of BMP-2 could be triggered by addition of DOX, further verifying the successful construction of Tet-On expression system for transferring hBMP-2 gene into BMSCs.
Regulation of BMP-2 by Tet-On expression system in monolayer culture
To evaluate the effect of Tet-On expression system on regulation of BMP-2, DOX was added into the DMEM with escalating concentration. And then the medium was harvested to determine the expression level of BMP-2 by means of ELISA assay. As shown in , the concentration of BMP-2 increased with higher concentration of DOX, thus suggesting that the regulation of BMP-2 exhibited in a DOX dose-dependent manner.
Figure 2. Expression level of BMP-2 in different DOX concentrations by ELISA analysis. BMSCs were seeded into 24-well culture plates at a density of 3 × 104 cells per well, 4h after co-transfection of rat BMSCs with ADV-hBMP2 and Adeno-X Tet-On, the culture fluids were replaced with 3 mL of fresh DMEM with supplement of 2% FBS and DOX (Sigma–Aldrich) at the following concentration: 0, 10−4, 10−3, 10−2, 10−1, 1, 10 μg/mL. Following additional 48-h incubation, the culture medium was collected to evaluate the expression level of BMP-2 by means of ELISA kit (R&D) according the manufacturer's manual. Results show highly significant differences between the induced and uninduced samples (p < 0.0001) when DOX concentration was over 0.001 ug/ml.
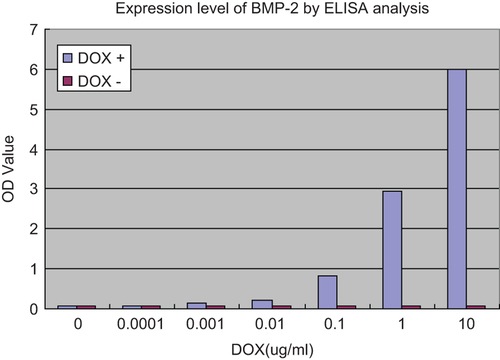
Viability of BMSCs in APA microcapsules
It was shown in and B that BMSCs displayed good viability after the process of microencapsulation. The curve of viability further demonstrated that cells in APA microcapsules would maintain considerable number.
Figure 3. BMSCs in APA microcapsules and viability of BMSCs in APA microcapsules. (A) BMSCs in APA microcapsules observed under light microscope; (B) FDA/EB staining for BMSCs in APA microcapsules observed under fluorescent microscope; (C) Curve of viability for BMSCs. 100 μL of microcapsule solution was harvested and transferred to each well in a 24-well plate and cell number per well was approximately 3 × 104. Then FDA/EB solution was added to each well at day 0, 1, 2, 4, 6, 10, 14, respectively to measure fluorescence intensity using fluorescence spectrophotometer at △ λ = 40 nm. Two peak values were obtained at the spectrum range of 500–650 nm. There, the peak value at 530 nm represented living cells and the peak value at 590 nm represented dead cells. Three samples for each time point were performed. No significant differences of the viability of BMSCs in APA microcapsules were observed after 14 days between the induced and uninduced samples (F = 15.486, P = 0.19).
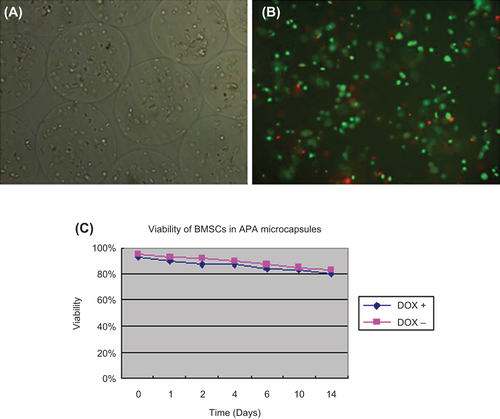
Adjustment of BMP-2 by Tet-On expression system in APA microcapsules
To investigate whether Tet-On expression system could work in APA microcapsules, BMSCs co-transfected with ADV-hBMP2 and Adeno-X Tet-On were seeded into APA microcapsules. Then DOX was added with increased concentration as indicated in . The data demonstrated that the more BMP-2 was expressed with the higher concentration of DOX which was within the range from 0 to 200 μg/mL. When beyond 200 μg/mL, the expression level of BMP-2 showed the trend of a decline.
Figure 4. Expression level of BMP-2 in APA microcapsules by ELISA analysis. BMSCs in APA microcapsules were seeded into 24-well culture plates at a density of 3 × 104 cells per well, 4h after co-transfection of rat BMSCs with ADV-hBMP2 and Adeno-X Tet-On, the culture fluids were replaced with 3 mL of fresh DMEM with supplement of 2% FBS and doxycycline (DOX) (Sigma–Aldrich) at the following concentration: 0, 10−1, 100, 101, 102, 2 × 102, 3 × 102 μg/mL. Following additional 48-h incubation, the culture medium was collected to evaluate the expression level of BMP-2 by means of ELISA kit (R&D) according the manufacture's manual. Results show highly significant differences between the induced and uninduced samples (p < 0.0001) when DOX concentration was over 0.1 ug/ml.
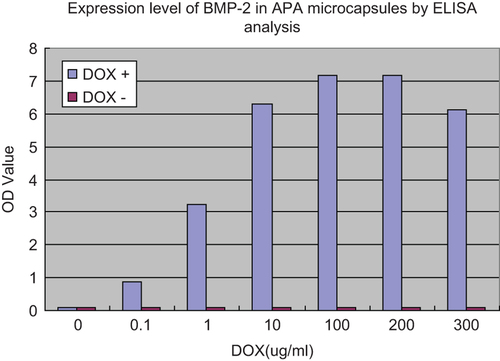
Alizarin red S staining for calcium deposition
BMSCs co-transfected with ADV-hBMP2 and Adeno-X Tet-On were encapsulated into APA and cultured in growth medium with DOX (1 μg/mL). The medium was changed every 2–3 days. After 2 weeks, the cells were recovered from APA microcapsules and cultured in osteogenic medium for 7 days. Then the cells were fixed followed by Alizarin red S staining. Meanwhile, uninfected BMSCs underwent in similar way. As shown in , BMSCs after treatment with both ADV-hBMP2 and Adeno-X Tet-On displayed more intensive staining than uninfected BMSCs did. And this result indicated that APA microencapsulation technology would not change the ability of BMSCs for differentiation.
Discussion
According to the current studies, it is needed to build a vector for high expression of target gene to provide adequate osteogenic factor for bone defect, regardless of simple gene therapy or the combinational use of genic technology and tissue engineering. In addition, we also found in our previous study that it was a contradiction between the faster degradation of biological material and slower new bone growth when growth factors and biological material were integrated and then planted to the bone defect. Therefore, it is required to regulate the expression of target gene so that the rate of degradation and tissue growth could match.
To this end, tetracycline-controlled transcriptional activation would provide an effective tool to control gene expression. Tet-On expression system consists of tetracycline responsive element (TRE) and reverse Tet-controlled transcriptional activator (RTTA). Tetracycline or DOX (a tetracycline derivative) can bind to RTTA and then induce the transcription and expression of target genes connecting with TRE (CitationFreundlieb et al. 1999). Yossi Gafni et al. (CitationGafni et al. 2004) first applied Tet-on expression system to bone repair and the results demonstrated that Tet-regulated rAAV-hBMP-2 vector promoted the expression of BMP-2 in high level and led to bone formation. However, they did not elucidate the relationship between the dose of DOX and expression level of BMP-2. In the present study, the vectors which can be expressed BMP-2 and Tet-On control system were constructed successfully. The results shown in indicated BMP-2 could be secreted significantly with the addition of DOX raised to 0.1 μg/mL. And the concentration of DOX had positive correlation with secretion of BMP-2. This expression system was also effective in APA microcapsules. As indicated in , more BMP-2 was secreted for higher addition of DOX. The expression of BMP-2 reached the peak when the concentration of DOX was between 100 and 200μg/mL. Then the drop of BMP-2 secretion showed up with DOX addition higher than 200 μg/mL. Hence, Tet-on expression system did work on the regulation of BMP-2 expression. This was a good foundation for the next step in in vivo experiments. The amount and time of BMP-2 secretion could be controlled by the addition of DOX.
Microcapsules have a semi-permeable membrane structure, which can block off immunoreactive substances of large molecules while allow small molecules nutrients and secretion through, thus forming immune isolation barrier (CitationLim and Sun 1980). APA microcapsules consist of sodium alginate and poly-lysine that hinge together by a certain form. Sodium alginate, which is widely used in tissue engineering (CitationZeng et al. 2014, CitationLee et al. 2011, CitationZhou and Xu 2011), is extracted from natural water-soluble alginate with many advantages like low immunogenicity, good biocompatibility, biodegradability, and low prices. Polylysine has good film-forming properties and chemical stability. APA microencapsulation technology has been proven useful for enhancement of gene therapy (CitationLi et al. 2006, CitationZhang et al. 2007).
Our data showed that most of BMSCs survived in APA microcapsules and maintained a stable viability (). Our previous study indicated BMSCs recovered from APA microcapsules possessed similar proliferation rate to those in culture plate with identical cell morphology and characteristics. Moreover, after BMSCs were recovered from APA and cultured in osteogenesis medium, calcium nodules showed up by Alizarin red staining, suggesting BMSCs in APA kept the osteogenic potential. However, when BMSCs were treated with Tet-on expression system combined with APA microencapsulation technology, the extent of osteogenesis was higher as shown by more intensive staining ().
In conclusion, we successfully constructed Tet-On expression system to regulate BMP-2 secretion and combined it with APA microencapsulation technology to improve the ability of immune isolation. In this study, adenovirus with high transfection efficiency and without teratogenicity and canceration was used as a vector in our control system. Microcapsule technology was also applied to avoiding the risk of in vivo immune attack. To our knowledge, the combinational use of microcapsule and Tet-On expression system has not been reported. The results showed that high level expression of BMP-2 with effective control by DOX could be realized in this system. The relationship between BMP-2 expression and DOX concentration has been explored and would provide an experimental basis for further study. In the future study, we would improve the efficacy of this system. For example, we would construct the vector with both BMP-2 and Tet-On expression system, thus enhancing the efficiency of co-transfection. In future we would aim to repair large bone defects by using tissue-engineered bone in animal models. We would compound calcium phosphate cement (CPC) and the Tet-On expression system to form a certain shape of complex using 3D printing. We expect to achieve a balanced condition between bone repair and degradation of CPC .The experiment results we have acquired provide applying technique for the in vivo bone tissue engineering and this treatment modality could be a promising approach for orthopedic applications.
Declaration of interest
The authors report no declarations of interest. The authors alone are responsible for the content and writing of the paper.
The authors thank the National Natural Science Foundation of China (fund num: 81371978) and Guangdong Natural Science Foundation (fund num: s2013010014787) for funds to the study.
References
- Barsoum SC, Milgram W, Mackay W, Coblentz C, Delaney KH, Kwiecien JM, et al. 2003. Delivery of recombinant gene product to canine brain with the use of microencapsulation. J Lab Clin Med. 142:399–413.
- Basic D, Vacek I, Sun AM. 1996. Microencapsulation and transplantation of genetically engineered cells: a new approach to somatic gene therapy. Artif Cells Blood Substit Immobil Biotechnol. 24:219–255.
- Chang TM. 1964. Semipermeable microcapsules. Science. 146:524–525.
- Cruz L, Assumpcao E, Andrade SF, Conrado DJ, Guterres SS, Pohlmann AR. 2010. Microencapsulation of sodium alendronate reduces drug mucosal damage in rats. Drug Deliv. 17:231–237.
- Fan J, Park H, Tan S, Lee M. 2013. Enhanced osteogenesis of adipose derived stem cells with Noggin suppression and delivery of BMP-2. PLoS One. 8:e72474.
- Freundlieb S, Schirra-Muller C, Bujard H. 1999. A tetracycline controlled activation/repression system with increased potential for gene transfer into mammalian cells. J Gene Med. 1:4–12.
- Gafni Y, Pelled G, Zilberman Y, Turgeman G, Apparailly F, Yotvat H, et al. 2004. Gene therapy platform for bone regeneration using an exogenously regulated, AAV-2-based gene expression system. Mol Ther. 9:587–595.
- Gossen M, Bujard H. 1992. Tight control of gene expression in mammalian cells by tetracycline-responsive promoters. Proc Natl Acad Sci U S A. 89:5547–5551.
- Han Q, Zhang B, Chen B, Dai J, Xu J, Wang C, Wang Z. 2013. Evaluation of a bioactive bone-inducing material consisting of collagen scaffolds and collagen-binding BMP2. J Biomed Mater Res A.
- Hernandez-Valdepena I, Braud C, Coudane J, Domurado D, Vert M. 2005. Molecular microencapsulation for in vivo transport of water-insoluble drugs. J Control Release. 101:296–297.
- Hidaka C, Goshi K, Rawlins B, Boachie-Adjei O, Crystal RG. 2003. Enhancement of spine fusion using combined gene therapy and tissue engineering BMP-7-expressing bone marrow cells and allograft bone. Spine (Phila Pa 1976). 28:2049–2057.
- Hortelano G. 2010. Therapeutic applications of cell microencapsulation. Foreword. Adv Exp Med Biol. 670:vii–viii.
- Khaled EG, Saleh M, Hindocha S, Griffin M, Khan WS. 2011. Tissue engineering for bone production- stem cells, gene therapy and scaffolds. Open Orthop J. 5:289–295.
- Lee GS, Park JH, Shin US, Kim HW. 2011. Direct deposited porous scaffolds of calcium phosphate cement with alginate for drug delivery and bone tissue engineering. Acta Biomater. 7:3178–3186.
- Li AA, Shen F, Zhang T, Cirone P, Potter M, Chang PL. 2006. Enhancement of myoblast microencapsulation for gene therapy. J Biomed Mater Res B Appl Biomater. 77:296–306.
- Lim F, Sun AM. 1980. Microencapsulated islets as bioartificial endocrine pancreas. Science (New York, NY). 210:908–910.
- Maus U, Andereya S, Gravius S, Ohnsorge JA, Niedhart C, Siebert CH. 2008. BMP-2 incorporated in a tricalcium phosphate bone substitute enhances bone remodeling in sheep. J Biomater Appl. 22:559–576.
- Mladenov KV, Kunkel P, Stuecker R. 2010. The use of recombinant human BMP-2 as a salvage procedure in the pediatric spine: a report on 3 cases. Eur Spine J. 19:S135–S139.
- Murakami N, Saito N, Takahashi J, Ota H, Horiuchi H, Nawata M, et al. 2003. Repair of a proximal femoral bone defect in dogs using a porous surfaced prosthesis in combination with recombinant BMP-2 and a synthetic polymer carrier. Biomaterials. 24:2153–2159.
- Quarto N, Li S, Renda A, Longaker MT. 2012. Exogenous activation of BMP-2 signaling overcomes TGFbeta-mediated inhibition of osteogenesis in Marfan embryonic stem cells and Marfan patient-specific induced pluripotent stem cells. Stem Cells. 30:2709–2719.
- Santos E, Orive G, Calvo A, Catena R, Fernandez-Robredo P, Layana AG, et al. 2012. Optimization of 100 mum alginate-poly-L-lysine-alginate capsules for intravitreous administration. J Control Release. 158:443–450.
- Schmoekel HG, Weber FE, Schense JC, Gratz KW, Schawalder P, Hubbell JA. 2005. Bone repair with a form of BMP-2 engineered for incorporation into fibrin cell ingrowth matrices. Biotechnol Bioeng. 89:253–262.
- Shegarfi H, Reikeras O. 2009. Review article: bone transplantation and immune response. J Orthop Surg (Hong Kong). 17:206–211.
- Tai IT, Sun AM. 1993. Microencapsulation of recombinant cells: a new delivery system for gene therapy. FASEB J. 7:1061–1069.
- Wang CJ, Weng LH, Hsu SL, Sun YC, Yang YJ, Chan YS, Yang YL. 2010. pCMV-BMP-2-transfected cell-mediated gene therapy in anterior cruciate ligament reconstruction in rabbits. Arthroscopy. 26:968–976.
- Yao Y, Zhang F, Zhou R, Su K, Fan J, Wang DA. 2010. Effects of combinational adenoviral vector-mediated TGF beta 3 transgene and shRNA silencing type I collagen on articular chondrogenesis of synovium-derived mesenchymal stem cells. Biotechnol Bioeng. 106:818–828.
- Young BH, Peng H, Huard J. 2002. Muscle-based gene therapy and tissue engineering to improve bone healing. Clin Orthop Relat Res.S243–251.
- Zeng L, Yao Y, Wang DA, Chen X. 2014. Effect of microcavitary alginate hydrogel with different pore sizes on chondrocyte culture for cartilage tissue engineering. Mater Sci Eng C Mater Biol Appl. 34: 168–175.
- Zhang J, Neoh KG, Hu X, Kang ET, Wang W. 2013. Combined effects of direct current stimulation and immobilized BMP-2 for enhancement of osteogenesis. Biotechnol Bioeng. 110: 1466–1475.
- Zhang Y, Wang W, Zhou J, Yu W, Zhang X, Guo X, Ma X. 2007. Tumor anti-angiogenic gene therapy with microencapsulated recombinant CHO cells. Ann Biomed Eng. 35:605–614.
- Zhou H, Xu HH. 2011. The fast release of stem cells from alginate-fibrin microbeads in injectable scaffolds for bone tissue engineering. Biomaterials. 32:7503–7513.