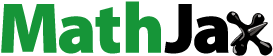
Abstract
The main objective of the present article was to prepare stable and well-dispersible budesonide (BUD) nanosuspensions by microfluidizer method. The morphology, particle size, and zeta potential of formulation were investigated and in vitro release and in vivo lung distribution were evaluated. Characterizations showed that BUD nanosuspensions were spherical in shape with a smooth surface. The measured average particle size was 122.5 ± 6.3 nm, and ζ potential was − 13.6 ± 0.4 mV. In vitro release behavior of three batches BUD nanosuspensions had a good reproduction. The deposition distribution of BUD different formulations was measured using a modified multi-stage liquid collision method. The data showed that BUD nanosuspensions have the most outstanding deposition distribution with fine particle ratio 82.2%. Compared with normal particle and micronized particles, nanosuspensions were easier to be distributed in lung. After inhalation of 1 h, the drug concentration can reach 872.9 ng/g, which was extremely significantly different from normal particles (p < 0.01) and significantly different from micronized particles (p < 0.05).
Introduction
Nowadays there are nearly 200 million asthma patients worldwide and the number keeps growing every year (CitationSpangler et al. 2013). In China, the number of patients has reached more than 10 millions in recent years (CitationXie and Wenzel 2013). According to reports (CitationPersson 2014, CitationBrightling and Desai 2013), bronchial asthma is a chronic airway inflammation, which involved a variety of inflammatory cells dominated by the eosinophils. Recurrent symptoms seriously impact the health and quality of life of patients. Clinically now, glucocorticoids are the most effective anti-allergic inflammatory drugs (CitationCarr 2013). Its administration method is mainly anapnotherapy, which means to deliver the drugs directly to the target organ – the lung. It helps to be absorbed in the lung receptor sites. Our new Bronchial Asthma Prevention Guide and the Global Initiative for Asthma considered inhalation corticosteroids as the most effective drug formulations to control airway inflammation in asthma (CitationOlaguibel et al. 2012, CitationKroegel 2009).
Currently, BUD is one of the mainly used corticosteroids clinically, which has two commercially available formulations including aerosol and dry powder inhalation (CitationDyer et al. 2006, CitationBarnes 2007, CitationPostma et al. 2008). Because of individual differences in the amount of air intake, dry powder inhalation is not as good as aerosol in terms of dose control, especially for children and elderly patients who find it more difficult to maintain a sufficient amount of the drug intake. Aerosol has good absorption effect, but its propellant contains Freon which is harmful to the environment and human bodies. In addition, since the drugs are water insoluble, when the drugs with a particle size of 0.5–7 μm enter the respiratory, some of the drugs may deposit in parts outside respiratory (mouth, throat and esophagus) leading to reduced effect of the drugs. Thus, doctors and patients both need a new kind of BUD delivery system.
Nanosuspensions is a stable colloidal dispersion of nanoparticles by using surfactant as suspending agent to spread drug kernel in water and crush or control crystallization technology (CitationKesisoglou and Mitra 2012, CitationLiu et al. 2012, CitationChavhan et al. 2011). It is different from the traditional matrix skeleton type nanosystems. Nanosuspensions technology can reduce the drug particle size, increase the drug-specific surface area, and make the drugs easier to be absorbed in the body. Thus, it contributes to increase the bioavailability of the drug. Nanosuspensions do not need carrier material, and with the stabilization of the surfactant, it can spread nano-drug particles in water to form a stable system.
The main objective of the present article was to prepare stable and well-dispersible BUD nanosuspensions and investigate the characteristic (morphology, particle size and zeta potential), in vitro release, and in vivo evaluation by using guinea pigs model.
Materials and methods
Materials
BUD APIs (Hangzhou Moon Fine Chemical Co., purity 99.6%, batch number 2010132); HPMC (hydroxypropyl methyl cellulose, Shanghai Colorcon Coating Technology Co., pharmaceutical grade); SLS (sodium lauryl sulfate, Shanghai chemical Reagent Co., domain research, pharmaceutical grade). All reagents for high performance liquid chromatography (HPLC) analysis, including acetonitrile and methanol were of HPLC grade. Other reagents were of analytical grade. Purified water from a Milli-Q system (Millipore, Bedford, MA, USA) was used throughout the experiment.
Preparation of nanosuspensions
HPMC (0.45 g) and SLS (0.3 g) were dissolved by stirring in an amount of water to swell overnight. After completely dissolved, 100 mg BUD were added into the solution and stirred until the final mix volume reached to 100 ml. Then the mixed solution was put in an ice bath and mixed probe sonication (220 W, 5 min, work 2 s, intermittent 1 s). After that, the mixed solution was homogenized by Nano DeBEE nano-pressure micro jet homogenizer (BEE International Company), parameters: pressure 22000 psi, the number of cycles was ten. Eventually, we get light blue opalescence BUD nanosuspensions.
Characterization
The morphological examination of the nanosuspensions was performed using a transmission electron microscope (Philips CM120, the Netherlands). In practice, a drop of BUD nanosuspensions diluted with water in room temperature condition was placed on a carbon film coated on a copper mesh and observed at 80 kV in the electron microscope. Particle size distribution and mean diameter of the prepared BUD nanosuspensions were determined by dynamic light scattering using a NICOMP 380 Submicron Particle Sizer (Santa Barbara, CA, USA) equipped with a 5-mW helium– neon laser at 632.8 nm. Sample solutions filtered through a 0.22-μm filter membrane were transferred into the light scattering cells. The intensity autocorrelation was measured at a scattering angle of 90o at room temperature. Data were analyzed in terms of intensity-weighted NICOMP distributions. Each reported experimental result was at the average of at least three dh values obtained from analysis of the autocorrelation function accumulated for at least 20 min.
In vitro release studies
In vitro release properties of BUD from the nanosuspensions were investigated in an aqueous release medium distilled water containing 0.1 N HCl solution by a dialysis method (CitationDaheb et al. 2013). Two milliliter of nanosuspensions were introduced into a dialysis bag (MWCO = 8–10 kDa). The end-sealed dialysis bag was immersed into 16 ml release medium at 37°C. The release medium was stirred at the speed of 60 rpm for 12 h. Samples of 0.2 ml were withdrawn at different time intervals and replaced with an equal volume of fresh release medium. The concentration of BUD in the samples was determined by the HPLC method described below.
Deposition distribution evaluation
The deposition distribution of BUD different formulations was measured using a modified multi-stage liquid collision method. was the schematic diagram of multi-stage liquid impinge (MSLI, Cop1ey, UK). Briefly, 0.1 ml BUD different formulations were sprayed into the MSLI. The drug with different particle sizes stay at different layers (throat and stages I–IV). The particle with diameter > 10 μm sediments in the throat and stage I layer, particle with 5–10 μm diameter mainly settles in the upper respiratory tract (stage II), particle with 1–5 μm diameter mainly settles in the lower respiratory tract and lungs (stage III), and the diameter < 1 μm deposition of inhaled particles settle in the lung (stage IV). After collecting all stages of the drug, the concentration of each layer containing BUD was calculated by HPLC method. The fine particle ratio (FPR) was used to evaluate the efficacy of the respiratory tract deposition distribution. FPR is defined in the formula described below; the higher the FPF, the more the particles that were smaller than 5 μm. It means that the more particles can reach the deep lung, the better effectiveness of the drugs. BUD powder and micronized particles were used as the contrast; each sample was parallel tested three times.
Lung distribution
Eighteen healthy Holstein guinea pigs (250–280 g, half male, purchased from Hospital Laboratory Animal Center) were used in the experiment to assess the effect of nanosuspensions on the distribution of BUD after inhale administration. The guinea pigs were divided into three groups at random and given a single 10 mg/kg dose of the BUD nanosuspensions, raw material particles or micronized particles. At 1 h after administration, each animal was euthanized, and lung samples were collected. Tissue samples were washed in ice-cold saline, blotted with paper towel to remove excess fluid, weighed and stored at − 70°C until assessed for drug concentration by HPLC.
HPLC analysis
The analysis of BUD levels in vitro and in vivo were carried out using an RP-HPLC method on a system equipped with a Agilent 1260 Series and a HS2000 interface (USA Agilent) operated at 245 nm. The column was Dikma Diamonsil C18 (5 μm, 200 × 4.6 mm). The mobile phase consisted of acetonitrile:water (40:60, v/v) and the flow rate for the mobile phase was 1.0 ml/min. The column temperature was 30°C. Tissue samples were homogenized in a mixed solution of 100 mg of lung tissue and 100 μl of water. Then stirring by a homogenizer, 100 μl mixed solution was added into 5 ml tube and 300 μl of acetonitrile was added for precipitation. After centrifugation at 12000 rpm for 10 min, the clear supernatant was removed and 20 μl of sample was used for HPLC analysis.
Results
As shown in , BUD nanosuspensions were spherical in shape with a smooth surface. The measured average particle size was 122.5 ± 6.3 nm, with a polydispersity of 0.19 ± 0.003. ζ potential was − 13.6 ± 0.4 mV. The chemical and physical stability assessment for BUD nanosuspensions was conducted to support long-term storage. Fresh-prepared formulations were stored at 25 ± 2°C for up to 12 months. Stability samples were then analyzed for physical change, drug content, particle size and zeta potential. The drug content in long-term storage conditions did not vary to a large extent in the nanosuspensions formulations; the maximum variation of 1.2% from the initial concentration was seen, 12 months from the date of manufacture. And the physical–chemical characteristics changes were found to be negligible and had no impact on the quality of the formulations. In this experiment, this release medium system could maintain a good sink condition for the in vitro release studies of BUD. showed the in vitro release profiles of three batches of BUD nanosuspensions. From the data, the in vitro release behavior had a good reproduction. In the initial 0.5 h, release was nearly linear with 10% released per day. Thereafter, a gradual slow release was observed and about 85% of the drug was released at 12 h. The in vitro release was kinetically analyzed according to zero-order, first-order, and the Higuchi release mechanism. The relative high correlation coefficient values obtained from the analysis of the amount of the drug released versus the square root of time indicated the release followed the Higuchi (CitationHiguchi 1962) kinetic model, as shown in .
Figure 3. In vitro release profiles of budesonide nanosuspensions (ϒ), powder (△) and micronization (◊) formulations (n = 3).
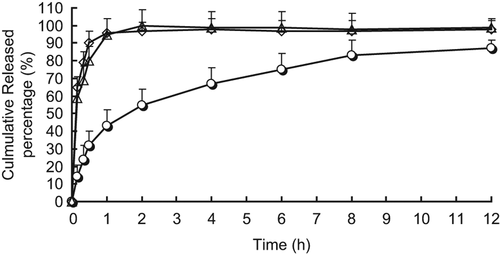
Table I. Correlation coefficients for kinetic analysis of release data for budesonide nanosuspensions.
and showed the ratio of the value of BUD deposition distribution of different formulations. It can be seen from BUD powder group that since most of the drugs had particles larger than 10 μm, nearly half of the particles were deposited in the throat layer and could not enter the lower layer of MSLI, with FPF only 6.1%. Secondly, after the BUD micronized group, most of its particle size was maintained around 1–5 μm; therefore, it can exhibit good deposition distribution, with particle size distribution mainly in the stage III layer (36%) and FPF reached 57.5%. BUD nanosuspensions have reached the level of nanoscale, so the distribution of the particles was the most outstanding, with only 2% and 5% of the particles distributed in the throat layer and stage I layer. A large number of particles were distributed in stage IV layer (70%) and FPF was 82.2%.
Table II. Fine particle ratio (FPR) for three different budesonide formulations (n = 3).
In vivo biodistribution behavior of BUD after inhale administration of nanosuspensions to rats was investigated with BUD powder and BUD micronized as a control. The amounts of drug distributed in unit mass of lung were measured (). Compared with normal particle and micronized particles, nanosuspensions were easier to be distributed in lung. After inhalation of 1 h, the drug concentration can reach 872.9 ng/g, which was extremely significantly different from normal particles (p < 0.01) and significantly different from micronized particles (p < 0.05).
Table III. Budesonide concentration in lung of three different budesonide formulations (n = 6).
Discussion
The BUD nanosuspensions had an average size around 100 nm with a narrow polydispersity. Surface charge is an important indication of the stability of a colloidal nanoparticle system in medium. The repulsion among the nanoparticles with same type of surface charge provides extra stability. The zeta potential of the BUD nanosuspensions indicated negative charges on the nanoparticle surface (− 13.6 ± 0.4 mV).
There are currently several classic methods for evaluating lung deposition of particles inhaled, one is in vitro airway simulation method, using artificial airway particle by particle size stratification to predict lung deposition model, and another method is for the in vivo distribution, direct evaluation of in vivo drug concentrations in lung tissue after administration by inhalation. This study combined the two methods to evaluate the advantage of BUD nanosuspensions.
As we all know, the size of particles is the key element that affect drug deposition in lung. Only the drug particles with diameter less than or equal to 1–5 μm can be deposited in the deep lung in order to play a pharmacodynamics. Thus, drug powder pulmonary is closely related with drug micronization technology. Many production methods are currently available for the preparation of the nanosuspensions. The study showed that the mechanical pulverization method is the most widely used pharmaceutical powder technology. However, it has disadvantages with large energy consumption, low efficiency, wide particle size distribution, and heat is not easily stabilizing drugs such as structural damage and degradation shortcomings. Supercritical technology is one of the hotspots of ultrafine particles in the preparation of 1–2 μm BUD formulations (CitationLobo et al. 2005, CitationVelaga et al. 2002). However, the manufacturing process performed under high pressure and high equipment requirements (CitationRasenack et al. 2003) is prepared to get BUD ultrafine particles by liquid precipitation, but the resulting particles were greater than 20% of 10 μm, which did not apply to pulmonary administration. Compared with traditional high-speed mixer, ultrasonic instrument and homogenization, the new high-pressure homogenizer (HPH) can produce smaller particle size with good distribution and stability. Also, the new HPH updates rapidly. The new generation of HPH and microfluidizer has better performance with higher efficiency and higher process stability. They are also suitable for industrial production and have been widely accepted and applied to many research institutions and production enterprises.
Declaration of interest
The authors report no declarations of interest. The authors alone are responsible for the content and writing of the paper.
The work is supported, in part, by a project from Shanghai Committee of Science and Technology, China (14JC1492000), and a grant from Shanghai Jiao Tong University Affiliated Sixth People’s Hospital, China (Sixth Hospital-1584)
References
- Barnes PJ. 2007. Using a combination inhaler (budesonide plus formoterol) as rescue therapy improves asthma control. BMJ. 335:513.
- Brightling CE, Desai D. 2013. Reply: role of primary necrosis/lysis of submucosal eosinophils in obese individuals with asthma. Am J Respir Crit Care Med. 188:1468–1469.
- Carr WW. 2013. New therapeutic options for allergic rhinitis: back to the future with intranasal corticosteroid aerosols. Am J Rhinol Allergy. 27:309–313.
- Chavhan SS, Petkar KC, Sawant KK. 2011. Nanosuspensions in drug delivery: recent advances, patent scenarios, and commercialization aspects. Crit Rev Ther Drug Carrier Syst. 28:447–488.
- Daheb K, Lecours JP, Lipman ML, Hildgen P, Roy JJ. 2013. Prediction of in vivo atenolol removal by high-permeability hemodialysis based on an in vitro model. J Pharm Pharm Sci. 16:657–664.
- Dyer MJ, Halpin DM, Stein K. 2006. Inhaled ciclesonide versus inhaled budesonide or inhaled beclomethasone or inhaled fluticasone for chronic asthma in adults: a systematic review. BMC Fam Pract. 7:34.
- Higuchi WI. 1962. The analysis of data on the medicament release from ointments. J Pharm Sci. 51:802–804.
- Kesisoglou F, Mitra A. 2012. Crystalline nanosuspensions as potential toxicology and clinical oral formulations for BCS II/IV compounds. AAPS J. 14:677–687.
- Kroegel C. 2009. Global Initiative for Asthma (GINA) guidelines: 15 years of application. Expert Rev Clin Immunol. 5:239–249.
- Liu Y, Xie P, Zhang D, Zhang Q. 2012. A mini review of nanosuspensions development. J Drug Target. 20:209–223.
- Lobo JM, Schiavone H, Palakodaty S, York P, Clark A, Tzannis ST. 2005. SCF-engineered powders for delivery of budesonide from passive DPI devices. J Pharm Sci. 94:2276–2288.
- Olaguibel JM, Quirce S, Juliá B, Fernández C, Fortuna AM, Molina J, et al. 2012. Measurement of asthma control according to Global Initiative for Asthma guidelines: a comparison with the Asthma Control Questionnaire. Respir Res. 13:50.
- Persson C.Primary lysis of eosinophils in severe desquamative asthma. Clin Exp Allergy. 2014; 44:173–183.
- Postma DS, Kerstjens HA, ten Hacken NH. 2008. Inhaled corticosteroids and long-acting beta-agonists in adult asthma: a winning combination in all? Naunyn Schmiedebergs Arch Pharmacol. 378:203–215.
- Rasenack N, Steckel H, Müller BW. 2003. Micronization ofanti- inflammatory drugs for pulmonary delivery by a controlled crystallization process. J Pharm Sci. 92:35–44.
- Spangler M, Hawley H, Barnes N, Saxena S. 2013. A review of guidelines and pharmacologic options for asthma treatment, with a focus on exercise-induced bronchoconstriction. Phys Sportsmed. 41:50–57.
- Xie M, Wenzel SE. 2013. A global perspective in asthma: from phenotype to endotype. Chin Med J (Engl). 126:166–174.
- Velaga SP, Berger R, Carlfors J. 2002. Supercritical fluids crystallization of budesonide and flunisolide. Pharm Res. 19:1564–1571.