Abstract
Boronate affinity systems have been recently used for the specific isolation of cis-diol group carrying biomolecules such as glycoproteins, nucleosides, carbohydrates. Nanosized materials have been extremely used for the biotechnological purposes due to their unique properties and their high surface areas. The objective of this presented work was to develop a new boronate affinity system for the nucleoside adsorption. For this purpose, poly(HEMA) nanoparticles were synthesized by using surfactant free emulsion polymerization technique and then functionalized with phenylboronic acid. Synthesized nanoparticles were characterized with FTIR, SEM, and Zeta size analysis. Nucleic acid adsorption experiments were repeated for different medium pH values, for various nucleosides concentrations, for different temperatures and ionic strengths, in order to determine the optimum adsorption conditions. In the light of these studies, it can be concluded that this boronate ligand carrying nanoparticles were very valuable for the separation of nucleosides.
Introduction
Nucleosides which construct the nucleic acids have very significant functions in biological systems (CitationBadruddoza et al. 2012). Nucleosides and their derivatives have been widely used for the production of medicines due to their significant biological and chemical effects. There are numerous methods for separation of nucleosides from the biological materials, such as, high performance liquid chromatography (CitationHelboe and Hansen 1999, CitationLiebich et al. 1997), mass spectrometry (CitationBullinger et al. 2008), electrochemical determination (CitationGoyal et al. 2007, CitationFortin et al. 2004), and capillary electrophoresis (CitationLin and Liu 2003). A recently used new separation technique for the nucleosides is boronate affinity chromatography.
The use of boronate affinity chromatography for separation of nucleic acid components and carbohydrates was first reported by CitationWeith et al. (1970). Since then, the specificity of the boronate ligand has been exploited for the separation of a wide variety of cis diol-containing compounds, including catechols, nucleosides, nucleotides, nucleic acids, carbohydrates, glycoproteins, and enzymes (CitationBergold and Scouten 1983). Although basic interaction in boronate affinity chromatography is the esterification between boronate ligands and cis-diols, there are some secondary interactions (hydrophobic interaction, ionic interaction, hydrogen bonding, and charge transfer interaction) that can play an important role. Application of boronate affinity chromatography using various supports has been investigated. For example, acrylamide-acrylamidephenylboronic acid copolymer membrane (CitationSallacan et al. 2002), thermosensitive N-isopropylacrylamide-4-vinylphenylboronic acid copolymer latex particles (CitationElmas et al. 2004), poly(4-vinylphenylboronic acid-co-N,N′-methylenebisacrylamide monolithic column (CitationRen et al. 2009), Au-Prussian blue nanocomposite (CitationLi et al. 2012), gold nanoparticles (CitationLiu et al. 2012), poly[(3-acrylamidophenyl)boronic acid-co-N,N′-methylenebisacrylamide]monolithic capillary (CitationWang et al. 2013), 3-aminophenylboronic acid functionalized-poly(3-chloro-2-hydroxypropylmethacrylate) microspheres (CitationÖzdemir et al. 2014) have been used for boronate affinity chromatography as a support materials.
Over the past decade, nanotechnology has become a vital area of research and development in many fields of science and technology. Specifically in the fields of biotechnology and bioanalytical chemistry, the combination of nanomaterials and biomolecules is of considerable recent interest (CitationHilliard et al. 2002). The nonporous nanoparticles have very small size and despite being nonporous, they permit to immobilize over 100 mg biomolecules (proteins, enzymes, peptides, nucleotides, nucleosides, and DNA) per gram wet particles (CitationBetancor et al. 2005).
In this study, poly(2-hydroxyethylmethacrylate) [poly (HEMA)] nanoparticles were prepared by surfactant-free emulsion polymerization technique. Then these nanoparticles were activated with 3-aminopropyltriethoxysilane (APTES) and were functionalized with phenylboronic acid (PBA). The PBA containing nanoparticles were characterized by Fourier transform infrared spectroscopy (FTIR), scanning electron microscopy (SEM), Zeta sizer technique and were used for the nucleoside adsorption. Adsorption studies were performed in a series of aqueous solution containing different amounts of nucleoside, pH values, temperature, and ionic strength. Additionally desorption of nucleosides and reusability of these nanoparticles was investigated.
Materials and methods
Materials
2-Hydroxyethylmethacrylate (HEMA), ethylene glycol dimethacrylate (EGDMA), potassium persulphate (KPS), and PBA were purchased from Sigma Chemical Co (St. Louis, MO). Poly(vinyl alcohol) (PVA) (molecular weight: 100.000, 98% hydrolyzed) was purchased from Aldrich (Munich, Germany). Nucleoside solution (100 mM) was purchased from Fermentas Inc. (Maryland, USA). All other chemicals were the guaranteed or of the highest purity commercially available and were used without further purification.
Preparation of poly(HEMA) nanoparticles
Poly(HEMA) nanoparticles were synthesized by using surfactant free emulsion polymerization according to the procedure in literature with minor modifications (CitationÖztürk et al. 2008). Briefly, a PVA stabilizer solution was prepared by dissolving 0.5 g of PVA in 100 mL of distilled water. Then, 0.6 mL of HEMA and 0.3 mL of EGDMA were mixed with the initial solution and incubated in an ultrasonic bath for 30 min. The initiator-KPS was added to the polymerization solution, and then this solution was incubated in a shaking water bath at 70°C for 7 h. After the polymerization, synthesized nanoparticles were washed with methanol and water, in order to remove unreacted monomers.
Activation of poly(HEMA) nanoparticles with 3-aminopropyltriethoxysilane
An activation agent, 3-aminopropyltriethoxysilane (APTES), was used for the activation of poly(HEMA) nanoparticles. For this purpose, synthesized nanoparticles were mixed with 200 μL of APTES solution (0.9%) for 1 h at room temperature. At the end of the activation process, silanized nanoparticles were washed several times with 10.0 mL of distilled water (CitationSteinberg et al. 2006).
Modification of activated poly(HEMA) nanoparticles with phenylboronic acid
Silanized poly(HEMA) nanoparticles were modified by using PBA. For this, 200 μL of poly(HEMA) solution was mixed with PBA solution (final concentration is 0.25 mg/mL) in MES buffer (25 mM, pH 6.0). This solution was stirred for 2 h by using an orbital shaker with the shaking rate of 200 rpm, and centrifuged at 10,000 rpm for 20 min. Supernatant solution was stored for the calculation of the binding amount of PBA.
Characterization of poly(HEMA) nanoparticles containing phenylboronic acid
FTIR spectrums of poly(HEMA) and PBA attached poly(HEMA) were obtained with a FTIR spectrophotometer (FTIR 8000 Series, Shimadzu, Japan). For this, dry polymers (about 0.1 g) were thoroughly mixed with KBr (0.1g, IR Grade, Merck, Germany), and pressed into a pellet and then the FTIR spectrum was recorded. Surface morphology and shape of nanoparticles were examined by SEM (Philips XL-30S- FEG- Almelo, The Netherlands) by using lyophilized polymers. A fragment of lyophilized polymer was mounted on SEM sample mount and was sputter coated with gold. Then the sample was placed into SEM and the surface morphology of particles was examined. The average size and the size distribution of synthesized nanoparticles were determined by using a Zeta sizer (Malvern Instruments, Model 3000, UK).
Adsorption of nucleoside onto nanoparticles containing phenylboronic acids
Adsorption of nucleoside onto nanoparticles containing PBA was performed in batch mode. The adsorption experiments were conducted for 2h at 25°C at a stirring rate of 100 rpm. Effects of pH of the medium, initial nucleoside concentration, temperature, and ionic strength on the adsorption capacity were studied. The pH of the adsorption medium was changed between pH 5.0 and 9.0 by using different buffer systems (25 mM MES for pH 5.0–6.0, 25 mM phosphate for 7.0–8.0, 25 mM carbonate for pH 9.0). To investigate the effects of the initial concentration of nucleoside on adsorption, nucleoside concentration was changed between 0.05 and 0.2 nM. To show the effects of the temperatures on the adsorption, adsorption studies were carried out between 4 and 50°C. To determine the effect of ionic strength at adsorption, different salts (NaCl, MgCl2, CaCl2) were used between 0.01 and 1 M. The amount of adsorbed nucleoside onto nanoparticles containing PBA was determined by measuring the initial and final concentrations of nucleoside by UV absorbance at 260 nm.
Desorption of nucleosides and reusability of nanoparticles
Nucleoside desorption from nanoparticles containing PBA was performed with 0.1 M Tris-HCl buffer (pH 9.0) at room temperature. The final nucleoside concentration in desorption medium was determined by absorbance at 260 nm. To determine the reusability of the nanoparticles containing PBA, nucleoside adsorption and desorption cycle was repeated five times.
Results and discussion
Characterization of nanoparticles containing phenylboronic acid
Synthesis and application of nanosized materials have been taking great attention due to their unique chemical and physical properties and large specific surface areas. In this study poly(HEMA) nanoparticles were prepared by surfactant free emulsion technique. Then PBA was attached to these nanoparticles by activated APTES. Schematic presentation of preparation of PBA attached nanoparticles was demonstrated in . The FTIR spectra of poly(HEMA) and PBA attached poly(HEMA) are shown in . The FTIR spectrum of poly(HEMA) have the characteristic stretching vibration band of hydrogen bonded alcohol, -OH, around 3500 cm− 1, vibration band of carbonyl groups, -C = O, around 1700 cm− 1, and stretching vibration band of C-H, around 3000 cm− 1. As seen , attachment of PBA onto poly(HEMA) nanoparticles was realized by same characteristic peaks. The sharp peak around 1725 cm− 1 was due to vibration of aryl C = C bands from PBA. The surface morphology and structure of these nanoparticles was investigated by SEM. As seen in , the particles were mono sized, spherical, nonporous, and about 200 nm in diameter. Nanoparticles can produce larger specific surface area and therefore may result in high ligand loading and high molecules (protein, nucleic acid) adsorption. Average particle size and size distribution of nanoparticles were evaluated by Zeta sizer and was found to be about 206.5 nm with 1.043 polydispersity ().
Adsorption of nucleoside onto nanoparticles containing phenylboronic acid
Boronate affinity chromatography has been widely used for the separation and recognition of the cis-diol group carrying compounds. Because of the high specificity of the boronate affinity systems, this chromatographic technique has been deeply used for the separation of the glycoproteins, enzymes, nucleosides, and carbohydrates. These cis-diol group carrying compounds are very important class of biomolecules which are used for the biotechnological purposes, and their separation, purification, and recognition have been attached with great importance. In this study poly(HEMA) nanoparticles were modified by APTES and used for PBA attachment. The binding of nucleosides onto the boronic acid functionalized nanoparticles occurred via the formation of cyclic borate ester by reaction between the boronic acid groups in the tetrahedral anionic form and diol groups of nucleosides (CitationElmas et al. 2004). The effect of pH on nucleoside adsorption behavior of nanoparticles containing PBA was evaluated. The effect of pH on specific binding capacities of the nucleosides is shown in . As seen from , maximum nucleoside adsorption was observed at pH 6.0, MES buffer. Above and below that pH value, adsorbed amount of nucleoside was decreased. The nucleoside binding at acidic pH may greatly facilitate the application of boronate affinity techniques to biological samples of acidic pH (e.g., urine, tears, and saliva) (CitationLi and Liu 2012). shows the effect of initial nucleoside concentration on nucleoside adsorption onto PBA modified nanoparticles. As presented in this figure, the nucleoside concentration were changed between 0.05 and 0.2 nM and the specific nucleoside binding capacity of the PBA modified nanoparticles was increased with the increase in the initial concentration of incubation solution. The increase in adsorption capacities with the increase in the initial nucleoside concentration is due to the increase in the driving force of the concentration gradient as expected (CitationÖzdemir et al. 2014). But relatively lower nucleoside adsorption was observed at the nucleoside concentration higher than 0.1 nM. This situation can be explained with steric hindrance. Maximum nucleoside capacity was found to be 35.6 mg/g nanoparticles at 0.1 nM nucleoside concentration. Reduction in the size of support materials can generally improve the efficiency of nucleoside adsorption. In the case of surface attachment, smaller particles can provide a larger surface area for higher nucleoside loading per unit mass of particles (CitationKim et al. 2006). shows the effect of temperature on adsorption of nucleoside. The adsorption of nucleoside on the PBA modified nanoparticles increased with increasing temperature. In previous studies it was reported that adsorption affinity and capacity were increased markedly at lower temperatures, and complex formation between diol groups and boronic acid groups occur more easily in the low temperature range (CitationElmas et al. 2004, CitationSingh and Willson 1999). In order to examine the effect of ionic strength of medium on the nucleoside adsorption onto the PBA modified nanoparticles different kinds of salts were studied (NaCl, MgCl2, and CaCl2). It was observed from this study that, while NaCl didn't affect the adsorption capacity of the nanoparticle, MgCl2 and CaCl2 increased the adsorbed amount of nucleoside. This also contributed the temperature study's findings that one of the main driving forces for the adsorption of the nucleoside onto the boronate affinity nanoparticles was hydrophobic interactions. It should be kept in mind that secondary interactions are important in the interaction of the nucleic acid components with boronates. These interactions might be hydrogen bonds between the hydroxyl groups, and/or hydrophobic interactions due to the aromatic boronate ligand, and/or ionic interactions. The nature of the base is also important, and purines, adenines, and guanines have stronger binding bounds than other bases, probably due to hydrophobic and/hydrogen bonding effects (CitationRosenberg et al. 1972).
Figure 5. The effect of pH on the nucleosides binding onto phenylboronic acid modified poly(HEMA) nanoparticles.
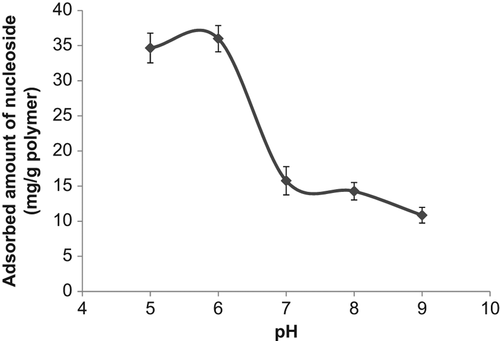
Nucleoside desorption from nanoparticles containing phenylboronic acid
In affinity separation processes, desorption of adsorbed molecule is very important. The nucleoside adsorbed on nanoparticles containing PBA was desorbed with 0.1 M Tris-HCl buffer at pH 9.0 and the desorption ratio was calculated as 90% at the end of 1 h. The adsorption-desorption cycle was repeated five times using the same nanoparticles in order to examine reusability of the nanoparticles. It was observed that the nanoparticles containing PBA can be used many times without decreasing their adsorption capacities significantly.
Conclusion
Boronate affinity chromatography uses the interaction of boronate with various cis-diol containing compounds. Boronate affinity chromatography has been successfully employed in the separation of nucleosides and nucleotides because it possesses 2′,3′-diol interacting group. In the presented work, PBA was used as a ligand for the affinity adsorption of nucleosides. Poly(HEMA) nanoparticles were synthesized as a support material and functionalized with PBA. This work reports on the adsorption of nucleosides by boronate affinity chromatography with nanoparticles. Nucleosides were successfully adsorbed on nanoparticles containing PBA and then adsorbed nucleosides were desorbed up to 90%. The positive results show the usability of the PBA attached poly(HEMA) nanoparticles as an affinity adsorbent.
Declaration of interest
The authors report no declarations of interest. The authors alone are responsible for the content and writing of the paper.
References
- Badruddoza AZM, Junwen L, Hidayat K, Uddin MS. 2012. Selective recognition and separation of nucleosides using carboxymethyl-β-cyclodextrin functionalized hybrid magnetic nanoparticles. Colloids Surf B Biointerfaces. 92:223–231.
- Bergold MJ, Scouten WH. 1983. Borate chromatography. In: Scouten WH, Ed. Solid Phase Biochemistry. New York: Wiley, pp. 149–187.
- Betancor L, Fuentes M, Dellamora OG, Lopez GF, Hidalgo A, Alonso MN, et al. 2005. Dextran aldehyde coating of glucose oxidase immobilized on magnetic nanoparticles prevents its inactivation by gas bubbles. J Mol Catal B Enzym. 32:97–101.
- Bullinger D. Fux R, Nicholson G, Plontke S, Belka C, Laufer S, Gleiter CH, Kammerer B. 2008. Identification of urinary modified nucleosides and ribosylated metabolites in humans via combined ESI-FTICR MS and ESI-IT MS analysis. J Am Soc Mass Spectrom. 19:1500–1513.
- Elmas B, Onur MA, Şenel S, Tuncel A. 2004. Thermosensitive N-isopropylacrylamide-vinylphenyl boronic acid copolymer latex particles for nucleotide isolation. Colloids Surf A. 232:253–259.
- Fortin E, Chane-Tune J, Mailley P, Szunerits S, Marcus B, Petit JP, et al. 2004. Nucleosides and ODN electrochemical detection onto boron doped diamond electrodes. Bioelectrochemistry. 63:303–306.
- Goyal RN, Gupta VK, Oyama M, Bachheti N. 2007. Voltammetric determination of adenosine and guanosine using fullerene- C60-modified glassy carbon electrode. Talanta. 71:1110–1117.
- Helboe T, Hansen SH. 1999. Separation of nucleosides using capillary electrochromatography. J Chromatogr A. 836:315–324.
- Hilliard LR, Zhao X, Tan W. 2002. Immobilization of oligonucleotides onto silica nanoparticles for DNA hybridization studies. Anal Chim Acta. 470:51–56.
- Kim J, Grate JW, Wang P. 2006. Nanostructures for enzyme stabilization. Chem Eng Sci. 61:1017–1026.
- Li H, Liu Z. 2012. Recent advances in monolithic column-based boronate-affinity chromatography. Trends Analyt Chem. 37:148–161.
- Li J, Wang Z, Li P, Zong N, Li F. 2012. A sensitive non-enzyme sensing platform for glucose based on boronic acid-diol binding. Sens Actuators B Chem. 161:832–837.
- Liebich HM, Di Stefano C, Wixforth A, Schmid HR. 1997. Quantitation of urinary nucleosides by high-performance liquid chromatography. J Chromatogr A. 763:193–197.
- Lin SY, Liu CY. 2003. An insight into the phenomena involved in a multiple-function stationary phase for the capillary electrochromatographic separation of 2′-, 3′-, and 5′-monophosphorylated nucleoside isomers. Electrophoresis. 24:2973–2982.
- Liu S, Du Z, Li P, Li F. 2012. Sensitive colorimetric visualization of dihydronicotinamide adenine dinucleotide based anti-aggregation of gold nanoparticles via boronic acid-diol binding. Biosens Bioelectron. 35:443–446.
- Özdemir N, Çakır A, Somtürk B. 2014. Boronic acid functionalized polymeric microspheres for catecholamine isolation. Colloids Surf A. 445:40–47.
- Öztürk N, Bereli N, Akgöl S, Denizli A. 2008. High capacity binding of antibodies by poly(hydroxyethyl methacrylaye) nanoparticles. Colloids Surf B Biointerfaces. 67:14–19.
- Ren L, Liu Y, Dong M, Liu Z. 2009. Synthesis of hydrophilic boronate affinity monolithic capillary for specific capture of glycoproteins by capillary liquid chromatography. J Chromatogr A. 1216:8421–8425.
- Rosenberg M, Wiebers J, Gilham PT. 1972. Studies on the interaction of nucleotides, polynucleotides, and nucleic acids with dihydroxylboryl-substituted cellulose. Biochemistry. 11:3623–3628.
- Sallacan N, Zayats M, Bourenko T, Kharitonov AB, Willner I. 2002. Imprinting of nucleotide and monosaccharide recognition sites in acrylamidephenylboronic acid- acrylamide copolymer membranes associated with electronic transducers. Anal Chem. 74:702–712.
- Singh N, Willson RC. 1999. Boronate affinity adsorption of RNA: possible role of conformational changes. J Chromatogr A. 840:205–213.
- Steinberg TG, Huynh M, Barker D, Zhao C. 2006. Synthetic modification of silica beads that allows for sequential attachment of two different oligonucleotides. Bioconjug Chem. 17:841–848.
- Wang X, Liu Y, Ren L, Li H, Liu Z. 2013. Development of poly((3-acrylamidephenyl)boronic acid-co-N,N-methylenebisacrylamide) monolithic capillary for the selective capture of cis-diol biomolecules. Anal Methods. 5:5444–5449.
- Weith HL, Wiebers JL, Gilham PT. 1970. Synthesis of cellulose derivatives containing the dihydroxyboryl group and a study of their capacity to form specific complexes with sugars and nucleic acid components. Biochemistry. 9:4396–4401.