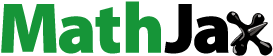
Abstract
Aim
In the present paper, paclitaxel (PTX)-loaded stealth liposomes were prepared with thin film hydration technique in order to prolong the time of circulation.
Method
The liposomes’ characterization, in vitro release, pharmacokinetics, and biodistribution profile of the drug were investigated.
Results
Microscope showed that the liposomes were spherical in shape with a smooth surface and the size was uniform and appropriate for administration via intravenous injection. The encapsulation efficiency was 91.1 ± 4.3%, and the mean diameter was 82.2 ± 7.6 nm from three batches. The results of in vivo studies indicated that PTX-loaded stealth liposomes show favorable pharmacokinetics and biodistribution compared to the free drug. In comparison with free PTX, the AUC0-t of PTX liposomes and stealth liposomes increased 1.91- and 3.39-fold, respectively. The stealth liposomes were long-circulating showing a half-life time of 34.2 h against 13.7 h for the conventional ones.
Conclusions
These results suggest that stealth liposomes would serve as a potent PTX delivery vehicle for the future cancer chemotherapy and represent a suitable platform for the development of targeted liposomal PTX systems.
Introduction
Paclitaxel (PTX) (), the prototype of taxane agents, emerges from a natural source (CitationZhang et al. 1971). PTX was found to be effective in treating a broad spectrum of advanced human cancer including non-small cell lung carcinoma (NSCLC) (CitationZhang et al. 2012, CitationOkamoto et al. 2014). The commercial PTX preparation (Taxol®) is formulated in the vehicle composed of Cremophor EL® (polyethoxylated castor oil used as a solubilizing surfactant) and dehydrated ethanol, which provides a homogeneous preparation. In the clinical application, PTX is usually administered as a 3-h and 24-h infusion representing a total dose of 135–175 mg/m2 of the body every 3 weeks (CitationKramer and Heuser 1995). However, the present-day chemotherapy employing Taxol® is accompanied by serious problems. One of the major problems associated with this formulation is the fact, that the diluted Cremophor EL®/ethanol vehicle is toxic (CitationDorr 1994). The negative side effects include serious hypersensitivity reactions, nephrotoxicity, and neurotoxicity (CitationWeiss et al. 1990).
Hence, the development of an improved delivery system for PTX is of high importance. Current approaches to the improvement are mainly focused on the development of formulations that are devoid of Cremophor EL®, investigation of the possibility of a large-scale preparation, and a request for a longer-term stability. Liposomes represent versatile and advanced nanodelivery systems for a wide range of biologically active compounds (CitationHofheinz et al. 2005). These relatively nontoxic systems have a considerable potential to entrap both hydrophobic and hydrophilic drugs. Recently, Lipusu® (liposomal PTX approved by State FDA of China) has entered the field of clinical applications (CitationYe et al. 2013, CitationXu et al. 2013, CitationWang et al. 2013). Generally, liposomes represent a promising approach to the optimization of PTX delivery.
Rapid clearance of the conventional liposomes by RES represents one of the major disadvantages in the drug delivery. This problem was solved by employing the long- circulating liposomes. The grafting of conventional liposomes with an inert and biocompatible polymer such as polyethylene glycol (PEG) leads to the formation of a protective and hydrophilic layer on the liposomal surface (CitationWoodle and Lasic 1992). The surface modification reduces a clearance of liposomes by the cells of reticuloendothelial system (RES) and apparently prolongs the half-life of liposomes during circulation (CitationUster et al. 1996). The long-circulating liposomes are also referred to as PEGylated, sterically stabilized, or stealth liposomes (CitationYang et al. 2014, CitationSur et al. 2014, CitationCai et al. 2014). So the present work was aimed at increasing the circulation time of PTX by preparing PTX-loaded stealth liposomes. The liposomes’ characterization, pharmacokinetics, and biodistribution profile of the drug were investigated.
Materials and methods
PTX was purchased from Zhejiang Haizheng Ltd, China. Hydrogenated soy phosphatidyl choline (SPC), distearoyl-L-a-phosphatidylethanolamine (DSPE) and high purity cholesterol (CHOL) were donated by Phospholipid Tech Ltd, Shanghai, China. PEG2000 and tocopherol were gifted from Sigma–Aldrich (Shanghai, China). All the chemicals and solvents used in the present study were of analytical grade. Deionized water was used throughout the experiment.
Preparation of liposomes
Liposomes were prepared with SPC:CHOL:PEG2000-DSPE:tocopherol:PTX = 16.2:3.8:1.3:0.2:1 molar compositions by thin film hydration technique (CitationUmrethia et al. 2007). Briefly, SPC, CHOL, and PTX were weighed accurately and then dissolved in organic phase, which is, chloroform (5 mL) in a 100-mL round bottom flask. This was attached to a rotary evaporator and the organic phase was removed by evaporating at 45 ± 2°C, which led to the formation of the film on the wall of the flask. The other processing conditions, such as rotational speed of evaporating flask (100 rpm) and vacuum (250 mmHg) were maintained constant. The round bottom flask containing thin lipid film was left in vacuum desiccator overnight to remove the solvent residuals if any. Then it was hydrated with phosphate-buffered saline (PBS), pH 7.4, using vortex mixture for about 2 min to form conventional liposomes. This liposomal suspension was allowed to stand at room temperature for about 2 h to achieve complete swelling. The resultant suspension was sonicated for 12 min in probe sonicator (220 W) to obtain small and homogenous vesicles and extruded through polycarbonate membrane of 0.2 mm pore size.
Size, zeta potential, and morphological observation
The particle sizes and zeta potentials of PTX-loaded stealth liposomes were evaluated in triplicate by dynamic light scattering (DLS), using a Zeta Potential Analyzer (Santa Barbara, CA, USA). Transmission electron microscope (TEM) (JEM-100CX, JEOL, Japan) was used for the morphological examination.
Entrapment efficiency
The entrapment efficiency (EE) was calculated by the percentage of PTX encapsulated in stealth liposomes relative to the total amount of PTX in stealth liposome suspension. To determine the total amount of PTX in stealth liposome suspension, 0.5 mL PTX stealth liposome was disrupted by the addition of 2 mL chloroform-methanol (2:1, v/v) to form a clear solution. The concentration of PTX was assayed by HPLC method at 230 nm compared to a standard curve.
To completely remove non-entrapped drug, liposomes were passed through a Sephadex G50 column (DEAE) equilibrated with PBS (pH 7.4). The resultant PBS fraction containing the stealth liposomes free of non-entrapped PTX collected and the entrapped PTX concentration was determined as described below.
EE was calculated using the following equation:
where Wincorporated is the weight of drug incorporated in liposomes, and Winitial is the weight of initial feeding drug.
Drug release study
The in vitro release of liposomes was analyzed according to the published method (CitationChen et al. 2010). Non-entrapped PTX was removed from liposomes over a Sephadex G-50 column as described above before an in vitro release test. The liposomes suspension (drug content: 10 mg) was placed in a dialysis bag with a molecular weight cut-off of 12,000. The dialysis bag was suspended in 100 mL isotonic PBS (pH 7.4, containing 0.1% Tween 80) which was incubated at 37°C under constant rotation at 75 rpm for 24 h. Samples of 2 mL were withdrawn at different time intervals (1, 2, 3, 4, 6, 8, 10, 12, 16, 20, and 24 h) and replaced with an equal volume of fresh release medium. The concentration of PTX in the samples was determined by the HPLC method described below.
Stability investigation
The stability assessment of liposomes was monitored for over 6 months at refrigerated temperature. At predetermined time intervals, samples were removed to room temperature and their physical and chemical stability were evaluated by drug content, physical appearance, particle size, and EE.
Pharmacokinetic studies
Eighteen Sprague–Dawley rats (purchased from Shanghai experimental animal center) were used to investigate the effect of liposomes formulation on the pharmacokinetics of PTX after intravenous (i.v.) administration. Rats were divided into three groups at random and given a single 5 mg/kg dose of the PTX-loaded stealth liposomes, PTX injection, and PTX conventional liposomes (made by phospholipid and CHOL) by tail-vein injection. As a control, PTX injection was diluted to 1.5 mg/mL with 5% glucose solution shortly before administration. Blood samples (0.5 mL) were collected into heparinized tubes from the orbital vein at 0, 0.5, 1, 2, 3, 4, 6, 8, 10, 12, 16, 20, 24, and 48 h after i.v. administration. Blood was immediately processed for plasma by centrifugation at 3000 × g for 10 min. Plasma samples were frozen and maintained at −70°C until analysis.
Biodistribution studies
Sixty mice bearing tumor xenograft (A549 cell) were used in the experiment to assess the effect of stealth liposomes formulation on the biodistribution of PTX after i.v. administration. The mice were divided into three groups at random and given a single 5 mg/kg dose of either PTX-loaded stealth liposomes, PTX injection, or PTX conventional liposomes by tail-vein injection. At 6, 12, 24, and 48 h after drug injection, each animal (n = 5 for each time point) was euthanized, then tumor, liver, spleen as well as plasma were collected. Tissue samples were washed in ice-cold saline, blotted with paper towel to remove excess fluid, weighed, and stored at −70°C until assessed for drug concentration by HPLC.
Systemic toxicity evaluation
At the 48-h of biodistribution studies, the rats of three groups (PTX injection, PTX conventional liposomes, and PTX-loaded stealth liposomes) were euthanized; the liver and kidney tissue were removed. The weight difference and clinical signs of toxicity were observed and the histopathological changes were recorded to evaluate their tissue compatibility.
HPLC analysis
The analysis of PTX levels in vitro and in vivo were carried out using an RP-HPLC method on a system equipped with a LC-10ATVP pump, a SPD-10AVP UV-Vis detector (Shimadzu, Kyoto, Japan), and a HS2000 interface (Empire Science & Tech, Hangzhou, China) operated at 230 nm. A reversed-phase column (Gemini 5 μm C18, 150 × 4.6 mm, Phenomenex, California, USA) was used at room temperature. The mobile phase consisted of acetonitrile and ammonium acetate buffer solution (10 mmol/L, pH 5.0) (50:45, v/v) was freshly prepared for each run and degassed before use. Samples of 20 μL were injected into the HPLC column for all the analysis. The flow rate for mobile phase was 1.0 mL/min, and the retention time of PTX was about 8.1 min.
Tissue samples were homogenized in a mixed solution of acetonitrile and water (50:50, v/v). Diazepam (1 μg/mL, 50 μL) as an internal standard was added into 200 μL of plasma or tissue samples, and vortexed for 1 min. The drug and internal standard were then extracted into 3 mL of anhydrous diethyl ether by vortex mixing for 2 min. After centrifugation at 3500 × g for 10 min, the clear supernatant was removed and evaporated under a gentle stream of nitrogen. The residue was then dissolved by 100 μL of acetonitrile and centrifuged at 1500 × g for 5 min before HPLC analysis.
Statistical analysis
Results are expressed as the mean ± S.D. of more than three experiments. Analysis of variance (ANOVA) was used to test the statistical significance of differences among groups (P < 0.05). Statistical significance in the differences of the means was determined using Student's t-test or Dunnett's test for the single or multiple comparisons of experimental groups, respectively.
Results and discussions
Preparation and characterization
In this study, PTX-loaded stealth liposomes were prepared by a composition of SPC:CHOL:PEG2000-DSPE: tocopherol:PTX = 16.2:3.8:1.3:0.2:1. As shown in , the surface morphology of PTX-loaded stealth liposomes was observed by TEM. The liposomes were spherical in shape with a smooth surface and the size was uniform and appropriate for administration via i.v. injection. The encapsulation efficiency of preparation were 91.1 ± 4.3% (n = 3), respectively, and the mean diameter was 82.2 ± 7.6 nm from three batches. The zeta potential was −3.6 ± 0.7 mV. According to former experience, the inclusion of CHOL at more than 20% caused PTX precipitation and liposome destabilization. The conventional PTX liposomes were found to be more stable than corresponding PEGylated ones (CitationCrosasso et al. 2000). So the composition of lipids comprising SPC, CHOL, and DSPE-PEG was optimized to improve the encapsulation capacity of PTX and prepare stable PEGylated liposomes. The addition of cholesterol allowed a preparation of small-sized liposomes with high drug incorporation.
In vitro release study
PTX release properties were evaluated in PBS containing 0.1% Tween 80. As shown in , free PTX exhibited a rapid release, with over 90% of the drug released into the media within 3 h incubation. On the other hand, PTX-loaded stealth liposomes achieved sustained release behaviors showing that the cumulative PTX release of drug loaded liposomes was less than 40% after 24 h incubation in PBS. With the presence of rat plasma, the release of PTX was accelerated dramatically and a large extent of drug release was observed. 65% of the drug released into the media within 24 h incubation. But both of these groups did not display burst initial release patterns.
Stability investigation
The 6-month comparative stability studies of lyophilized liposomes at refrigerated temperature showed that there were negligible drug loss and changes in vesicle size without any change in color in both the forms of liposomes at the end of first month. At the end of the third month, percent drug loss was increased (2.1%) for liposomes and with a little increase in particle size (1.1 nm). After the sixth month, the lyophilized liposomes showed only 3.6% of drug loss with particle size increase of 2.1 nm. Thus the freeze dried form of liposomes was found to be stable for nearly 6 months. There were no noticeable physical appearance and EE changes observed for liposomes at the end of the sixth month.
Pharmacokinetic studies
The plasma concentration time profiles of PTX following i.v. administration of 5 mg/kg PTX-loaded stealth liposomes, PTX injection, and PTX conventional liposomes are shown in . The pharmacokinetic parameters are summarized in . As seen in and , after encapsulation into stealth liposomes, the pharmacokinetics of PTX were significantly improved. In comparison with free PTX, the AUC0-t of PTX liposomes and stealth liposomes increased 1.91- and 3.39-fold, respectively. Moreover, the stealth liposomes were long-circulating showing a half-life time of 34.2 h against 13.7 h for the conventional ones. It is a result of a reduced clearance of the PTX stealth liposomes. Rapid clearance of the conventional liposomes by RES represented one of the major disadvantages in the drug delivery. This problem was solved by employing the long-circulating liposomes. The grafting of conventional liposomes with an inert and biocompatible polymer such as PEG led to the formation of a protective and hydrophilic layer on the liposomal surface. The surface modification reduced a clearance of liposomes by the cells of RES and apparently prolonged the half-life of liposomes during circulation.
Figure 4. Mean plasma concentration-time profiles of PTX-loaded stealth liposomes (blue line), PTX injection (green line), and PTX conventional liposomes (red line). (Each group represents mean ± SD of six rats).
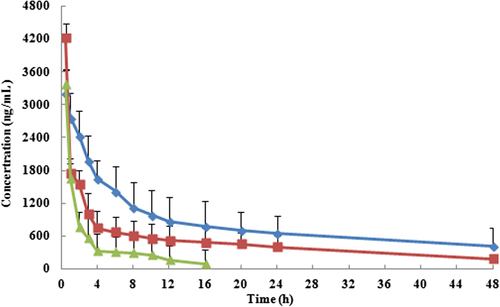
Table I. Pharmacokinetic parameters of 5 mg/kg PTX-loaded stealth liposomes, PTX injection and PTX conventional liposomes (n = 6).
Biodistribution studies
The biodistribution effect of PTX was evaluated after i.v. administration of 5 mg/kg (single dose) of PTX injection, conventional and stealth liposomes in mice model bearing tumor xenograft which was shown in . The Plasma PTX concentration-time profiles observed in mice were similar to the pharmacokinetics study in rats. The PTX AUC0–t of stealth liposomes was lower in liver and spleen, and higher in plasma and tumor tissue compared to PTX conventional liposomes. There were significant differences in plasma, liver, spleen, and tumor tissue between both formulations (p < 0.05). The studies demonstrated stealth liposomes decrease in PTX uptake in RES-containing organs (liver and spleen) after 48 h in comparison with their conventional liposomes. Long circulation time and slow release of PTX from stealth liposomes offered a chance for PTX to be attained at tumor through enhanced permeability and retention (EPR) effect and maintain the effective therapeutic level for a long time-period via a depot effect. After 48 h, mice's liver and spleen tissue sections of the experimental groups were normal, with no structural damage and toxic substances deposited which suggested that the drug in the body had no toxic effects ().
Figure 5. Tissue sections isolated on 48 h after treatment and stained with hematoxylin and eosin for histopathological evaluation. (A) Liver. (B) Spleen (× 200). (a) PTX injection. (b) PTX conventional liposomes and (c) PTX-loaded stealth liposomes.
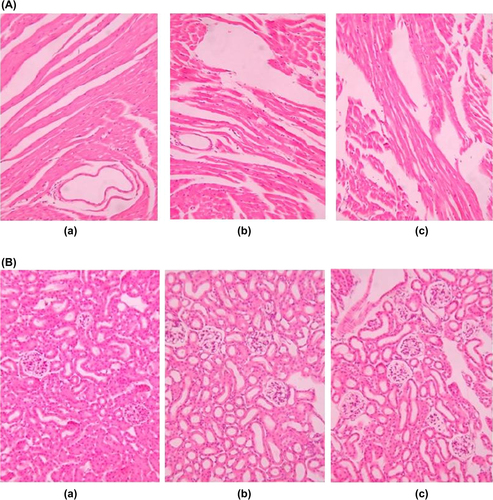
Table II. AUC0-t of different tissue of PTX injection, PTX conventional liposomes, and PTX-loaded stealth liposomes (n = 5).
It was demonstrated that the permeability of the capillary endothelium in the tumors was increased in comparison with normal tissues (CitationJain 1987). The macromolecules are passively accumulated to greater extent for longer period in the tumor than in the nonmalignant capillary endothelium. This phenomenon is referred to as an EPR effect (CitationMaeda and Matsumura 1989). Small long-circulating liposomes (100 nm) demonstrated a higher frequency of encountering permeable capillaries of the tumor and extravasating into the fenestrated tumor tissue. This accumulation of long-circulating liposomes with encapsulated drugs by EPR effect represents a passive targeting mechanism enhancing the drug delivery and drug therapeutic potential (CitationYuan et al. 1995).
Conclusion
In the present paper, PTX-loaded stealth liposomes were prepared with SPC:CHOL:PEG2000-DSPE:tocopherol: PTX = 16.2:3.8:1.3:0.2:1 molar compositions by thin film hydration technique. The liposomes were spherical in shape with a smooth surface and the size was uniform and appropriate for administration via i.v. injection. The encapsulation efficiency of preparation were 91.1 ± 4.3% (n = 3), respectively, and the mean diameter was 82.2 ± 7.6 nm from three batches. The zeta potential was −3.6 ± 0.7 mV. In addition, our study indicates that PTX-loaded stealth liposomes showed favorable pharmacokinetics and biodistribution from the free drug. In comparison with free PTX, the AUC0-t of PTX liposomes and stealth liposomes increased 1.91- and 3.39-fold, respectively. The stealth liposomes were long-circulating showing a half-life time of 34.2 h against 13.7 h for the conventional ones. These results suggested that stealth liposomes would serve as a potent PTX delivery vehicle for the future cancer chemotherapy and represent a suitable platform for the development of targeted liposomal PTX systems.
Declaration of interest
The authors report no declarations of interest. The authors alone are responsible for the content and writing of the paper.
This work was supported by Dr. Wu Jingyun of Merck for the statistic service in this study.
References
- Cai D, Gao W, He B, Dai W, Zhang H, Wang X, et al. 2014. Hydrophobic penetrating peptide PFVYLI-modified stealth liposomes for doxorubicin delivery in breast cancer therapy. Biomaterials. 35:2283–2294.
- Chen J, Lin A, Chen Z, Wang W, Zhang T, Cai H, Cai B. 2010. Ammonium sulfate gradient loading of brucine into liposomes: effect of phospholipid composition on entrapment efficiency and physicochemical properties in vitro. Drug Dev Ind Pharm. 36:245–253.
- Crosasso P, Ceruti M, Brusa P, Arpicco S, Dosio F, Cattel L. 2000. Preparation, characterization and properties of sterically stabilized paclitaxel-containing liposomes. J Control Release. 63:19–30.
- Dorr RT. 1994. Pharmacology and toxicology of Cremophor EL diluents. Ann Pharmacother. 28:511–514.
- Hofheinz RD, Gnad-Vogt SU, Beyer U, Hochhaus A. 2005. Liposomal encapsulated anticancer drugs. Anticancer Drugs. 16:691–707.
- Jain RK. 1987. Transport of molecules across tumor vasculature. Cancer Metastasis Rev. 6:559–593.
- Kramer I, Heuser A. 1995. Paclitaxel pharmaceutical and pharmacological issues. Eur Hosp Pharm. 1:37–41.
- Maeda H, Matsumura Y. 1989. Tumoritropic and lymphotropic principles of macromolecular drugs. Crit Rev Ther Drug Carrier Syst. 6:193–210.
- Okamoto I, Sakai K, Morita S, Yoshioka H, Kaneda H, Takeda K, et al. 2014. Multiplex genomic profiling of non-small cell lung cancers from the LETS phase III trial of first-line S-1/carboplatin versus paclitaxel/carboplatin: results of a West Japan Oncology Group study. Oncotarget. 5:2293–2304.
- Sur S, Fries AC, Kinzler KW, Zhou S, Vogelstein B. 2014. Remote loading of preencapsulated drugs into stealth liposomes. Proc Natl Acad Sci USA. 111:2283–2288.
- Umrethia M, Ghosh PK, Majithya R, Murthy RS. 2007. 6-Mercaptopurine (6-MP) entrapped stealth liposomes for improvement of leukemic treatment without hepatotoxicity and nephrotoxicity. Cancer Invest. 25:117–123.
- Uster PS, Allen TM, Daniel BE, Mendez CJ, Newman MS, Zhu GZ. 1996. Insertion of poly (ethylene glycol) derivatized phospholipid into pre-formed liposomes results in prolonged in vivo circulation time. FEBS Lett. 386:243–246.
- Wang H, Cheng G, Du Y, Ye L, Chen W, Zhang L, et al. 2013. Hypersensitivity reaction studies of a polyethoxylated castor oil-free, liposome-based alternative paclitaxel formulation. Mol Med Rep. 7:947–952.
- Zhang MC, Taylor HL, Wall ME, Coqqon P, McPhail AT. 1971. Plant antitumor agents. VI. The isolation and structure of taxol, a novel antileukemic and antitumor agent from Taxus brevifolia. J Am Chem Soc. 93:2325–2327.
- Weiss RB, Donehower RC, Wiernik PH, Ohnuma T, Gralla RJ, Trump DL, et al. 1990. Hypersensitivity reactions from taxol. J Clin Oncol. 8:1263–1268.
- Woodle MC, Lasic DD. 1992. Sterically stabilized liposomes. BBA-Biomembr. 1113:171–199.
- Xu X, Wang L, Xu HQ, Huang XE, Qian YD, Xiang J. 2013. Clinical comparison between paclitaxel liposome (Lipusu®) and paclitaxel for treatment of patients with metastatic gastric cancer. Asian Pac J Cancer Prev. 14:2591–2594.
- Yang G, Yang T, Zhang W, Lu M, Ma X, Xiang G. 2014. In vitro and in vivo antitumor effects of folate-targeted ursolic acid stealth liposome. J Agric Food Chem.; 62:2207–2215.
- Ye L, He J, Hu Z, Dong Q, Wang H, Fu F, Tian J. 2013. Antitumor effect and toxicity of Lipusu in rat ovarian cancer xenografts. Food Chem Toxicol. 52:200–206.
- Yuan F, Dellian M, Fukumura D, Leunig M, Berk DA, Torchilin VP, Jain RK. 1995. Vascular permeability in a human tumor xenograft — molecular-size dependence and cutoff size. Cancer Res. 55: 3752–3756.
- Zhang HL, Ruan L, Zheng LM, Whyte D, Tzeng CM, Zhou XW. 2012. Association between class III β-tubulin expression and response to paclitaxel/vinorebine-based chemotherapy for non-small cell lung cancer: a meta-analysis. Lung Cancer. 77: 9–15.