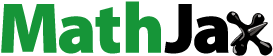
Abstract
In this paper, a novel amperometric L-glutamate (Glu) biosensor with immobilization of L-glutamate oxidase (L-GlOx) on polypyrrole-polyvinylsulphonate (PPy-PVS) film has been successfully developed. L-GlOx enzyme was immobilized on PPy-PVS film by cross-linking with glutaraldehyde (GA) and bovine serum albumin (BSA). Determination of Glu was carried out by oxidation of enzymatically produced H2O2 at 0.3 V versus Ag/AgCl. The optimum pH and temperature parameters were found to be 9.0 and 55°C, respectively. There were three linear parts in the regions between 1.0 × 10− 9 and 1.0 × 10− 8 M (R2 = 0.847), 5.0 × 10− 8 and 5.0 × 10− 7 M (R2 = 0.997), 5.0 × 10− 7 and 5.0 × 10− 5 M (R2 = 0.994). Storage stability, operation stability of the enzyme electrode were also studied.
Introduction
L-glutamate (Glu) is one of twenty amino acids forming proteins and found naturally in food that contain proteins. It can be synthesized in mammalian metabolism and can also be taken into metabolism from food. Glu has important functions because it is found in the central point of mammalian metabolism. First, it is a neurotransmitter in central nervous system and brain uses it as a transmitter during the transmission of nerve impulses (CitationGhobadi et al. 1996, CitationMaalouf et al. 2007). Furthermore, Glu also plays an essential role in many metabolic processes. It plays a major role in detoxification of nitrogen in nitrogen metabolism by performing the deamination reaction with water. It is used by brain when glucose storage is not enough. As a result of a transamination of α-ketoglutarate which locates in TCA cycle, Glu consists (CitationNelson and Cox 2000). Glu is very important clinically, because increased Glu level in cerebrospinal fluid is observed in neural disorders such as epilepsy, schizophrenia, Alzheimer's and Parkinson's diseases. Its quantification is found to be useful for diagnosis of these neurological disorders. Also, it is often used in clinical laboratories for the determination of aminotransferase activities (CitationCooper et al., 1991; CitationNelson and Cox 2000). Glu has an important place in food industry as well as clinically. It is widely used as a flavor enhancer in soups, sauces, and many processed foods (CitationMaalouf et al. 2007). Glutamate sodium salts are used as food additive (CitationJanarthanan and Mottola 1998). E-621 is classified as monosodium glutamate (MSG) that is used in foods as a flavor enhancer. There are different views about the effects of MSG on human body. Some researchers approach cautiously to this substance, some of them expressed that it triggers tension and headache; some other researchers explain that MSG is not harmful to human body (CitationJanarthanan and Mottola 1998).
A reliable, simple, and economic method for the determination of Glu which is important for both clinical sector and food industry would be useful. Many analytical methods have been improved until now for Glu determination such as spectrophotometry (CitationValero and Garcia-Carmona 1998), fluorescence (CitationChapman and Zhou 1999), chromatographic techniques (CitationSwanepoel et al. 1996), and capillary electrophoresis (CitationTucci et al. 1998). On the other hand, biosensors are better methods in some ways. Because of their good specifications such as high sensitivity and selectivity, rapid response, low costs, etc., biosensors have become an extremely broad field which impact on many sectors including clinical, pharmaceutical, food and environmental sectors (CitationAmine et al. 2006). So, biosensors for determination of Glu would be very useful.
In this study, we report a new amperometric biosensor for the determination of Glu. L-GlOx was immobilized onto a PPy-PVS film surface by cross-linking with glutaraldehyde (GA) and BSA. The determination of Glu was performed by oxidation of enzymatically generated H2O2. Optimum working conditions of biosensor with respect to the substrate concentration, pH, and temperature were investigated. Also the storage stability and operation stability of the biosensor were investigated.
Material and methods
Equipment and reagents
The electrochemical studies were carried out by using Epsilon EC electrochemical analyzer with a three-electrode cell. The working electrode was a Pt plate (0.5 cm2). Auxiliary and reference electrodes were Pt wire and Ag/AgCl electrode (3.0 M KCl), respectively. The pH values of the buffer solutions were measured with an ORION Model 720A pH/ion meter. Temperature control was achieved with Grant GD 120 thermostat. Glu oxidase (EC 1.4.3.11, purified from the Streptomyces sp., activity of 1.0 unit/mL) was purchased from Sigma. L-glutamic acid monosodium salt monohydrate was also purchased from Aldrich. Pyrrole and sodium polyvinylsulphonate were supplied from Fluka and Aldrich, respectively. All other chemicals were obtained from Sigma. All of the solutions were prepared by using distilled water. Scanning electron microscopy was done by using a JEOL JEM 100 CX II scanning electron microscope (JEOL, Peabody, MA) equipped with a Link analytical system.
Preparation of Pt/PPy-PVS film electrode
The surface of the Pt plate electrode was cleaned (CitationGros et al. 2000) and covered with PPy-PVS film by electropolymerization of pyrrole in a medium containing sodium polyvinylsulphonate (CitationChaubey et al. 2000) The Pt plate electrode was immersed in 10.0 mL solution containing 0.1 M pyrrole and 2.5 mL (25.0%) of sodium polyvinylsulphonate. The solution was purged with argon in order to remove the oxygen. Pyrrole electropolymerization was carried out on the Pt electrode surface by cyclic voltammetric scans between −1.0 and 2.0 V at a scan rate of 50 mV/s [vs. Ag/AgCl electrode (3.0 M KCl)]. PPy-PVS film was obtained after 1 cycle ( and ). After electropolymerization, PPy-PVS film was rinsed with deionized water to remove the unreacted pyrrole monomer. In , the growth of PPy-PVS film is seen. In , it can be seen that the surface morphology of the PPy-PVS film is a cauliflower-like structure.
Immobilization of L-GlOx on Pt/PPy-PVS film electrode
After electropolymerization, the mixture solution of 50.0 μL of L-GlOx (1.0 unit/mL), 1.0 mg of BSA, 81.25 μL of 0.1 M glycine buffer at pH of 9.0, and 7.5 μL of 2.5% GA was dropped on Pt/PPy-PVS film. Electrode was dried at room temperature and washed with buffer solution (0.1 M glycine buffer of pH 9.0) several times in order to remove the excess non-immobilized enzyme and GA. Immobilized enzyme electrode was kept in a refrigerator at 4°C in glycine buffer, when not in use.
Amperometric measurements
Quantification of Glu was achieved via electrochemical detection of enzymatically released H2O2.
Enzyme electrode was immersed into glycine buffer (0.1 M) at pH 9.0. The solution contained 0.1 M sodium chloride as supporting electrolyte. Electrode was brought to equilibrium by keeping it at 0.3 V [vs. Ag/AgCl electrode (3.0 M KCl)]. Steady-state current (ia) was recorded. Glu solution was added to the cell from stock solution and the system was stirred. Current (ib) values obtained at 0.3 V were recorded and current values (Δi = ib-ia) were plotted against the Glu concentration.
Results and discussion
In this study, we reported a new L-GlOx-based amperometric biosensor for the determination of Glu. L-GlOx was immobilized onto a PPy-PVS film surface by cross-linking with GA and BSA. The determination of Glu was performed by oxidation of H2O2, which occurs during enzymatic reactions at 0.3 V versus Ag/AgCl. Reaction in , which shows the Glu determination, is shown below.
According to this scheme, a biochemical reaction occurs between Glu in solution and L-GlOx enzyme which is immobilized onto PPy-PVS electrode. First, Glu is oxidized to α-ketoglutarate by L-GlOx. And flavin adenine dinucleotide (FAD), which is a prosthetic group in enzyme structure, is reduced to FADH2 by taking electron. FADH2 in enzyme is oxidized by giving its electrons to oxygen in solution to make the reaction reversible. Thus, enzyme comes to its previous form. By taking the electron, oxygen is reduced to H2O2. Glu determination was made by measuring the anodic current of hydrogen peroxide on electrode surface.
The parameters affecting the performance of biosensor and optimum working conditions were investigated.
Determination of working potential
After preparing Pt/PPy-PVS electrodes, hydrogen peroxide oxidation was carried out at different potentials (0.2–0.5 V) (). When was examined, it was seen that the variation in current in high potentials was higher than in low potentials. Compared with other potentials, the oxidation currents of H2O2 at 0.2 V were too low. Interference effects of substances presented in body fluids (e.g., ascorbic acid, uric acid) could be more in high potentials (CitationZhang et al. 2007). Therefore 0.3 V was used as working potential.
Effect of glutaraldehyde amount on the response of biosensor
Cross-linking is one of the suitable enzyme immobilization methods in the construction of a biosensor. GA (CHO-CH2CH2CH2-CHO) is a commonly used cross-linking reagent. It reacts with amine groups of enzyme molecules to make the conjugation. Because of its small size, GA can not only react with amine groups on the enzyme's surface but can diffuse into the enzyme and bind to interior amine groups (CitationXin and Wightman 1997). This case can affect the three-dimensional structure of an enzyme and also its catalytic function, because enzyme's active region can be destroyed by over-cross-linking (CitationXin and Wightman 1997). So, to improve the performance of the biosensor, amount of GA has to be investigated.
Different percentages of GA solutions (0.08, 0.13, and 0.67%) were used for immobilization of L-GlOx. The results demonstrated that the activity of the cross- linking system increased when the GA percentage was increased from 0.08% to 0.13% (). But when the GA percentage was 0.67%, activity of the cross-linking system decreased. 0.13% was decided as suitable GA amount. Because, 0.08% GA concentration was not enough, but 0.67% was much and caused enzyme activity loss.
Figure 5. The effect of glutaraldehyde amounts on the response of the biosensor (at 25°C, 1.0 × 10− 7–1.0 × 10− 3 M Glu at 0.3 V operating potential). (


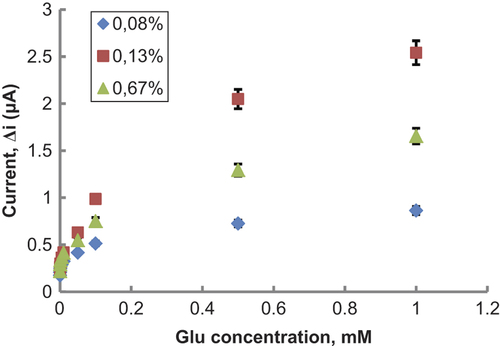
In this study, GA percentage was kept constant at 0.13% which was observed to give maximum activity with reasonable mechanical stability. It was also selected in the further characterization experiments.
Effect of pH on amperometric response of biosensor
pH plays an important role in maintaining the proper conformation of an enzyme, because enzyme activity is dependent on the ionization state of the amino acids in the active site. The effect of pH on the response of the Glu biosensor was tested varying between pH 6.0 and 10.0. The measurements were performed at a constant Glu concentration 1.0 × 10− 3 M with 0.1 M phosphate and glycine buffers varied between pH 6.0 and 10.0. shows that the maximum response was obtained at pH 9.0.
Figure 6. The effect of pH on the response of the biosensor (at 25°C, 1.0 × 10− 3 M Glu, at 0.3 V operating potential).). (

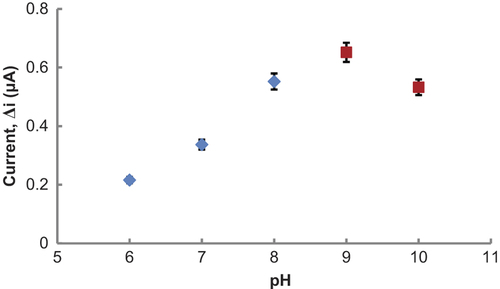
pH values, greater than physiologic conditions, were also seen in biosensors which were prepared from PPy-PVS polymers (CitationArslan and Arslan 2011, CitationÖzdemir et al. 2012). L-GlOx enzyme kept its activity even at high pH, when they were immobilized to PPy-PVS.
The difference in pH values was attributed to the fact that the used polymer and the type of immobilization were different. Because, change in the environment of enzyme's active site can cause different interactions between enzyme and immobilization materials (CitationBasu et al. 2006, CitationChang et al. 2007). Furthermore, enzymes become stronger after immobilization. Immobilized enzymes can show high activities under different pH conditions when compared with free enzyme, as the stable substrate-enzyme complex may form.
Effect of temperature
Temperature is an important factor which has a significant effect on enzyme activity. It is important to investigate the dependence of the response of the enzyme electrode to temperature. The relationship between reaction rate of an enzyme and temperature is exponential. The amperometric response of the prepared biosensor was investigated at different temperatures by using constant Glu concentration of 1.0 × 10− 3 M (). As seen from the , the current difference increases with temperature up to 55°C and decreases afterwards. The highest current difference was obtained at 55°C. The enzyme was thought to be denaturized after this temperature. The study was carried out at 25°C due to the difficulties involved in working at 55°C. For Glu biosensor, temperature values different than 55°C were employed as found in the literature (48; 65°C) (CitationPan and Arnold 1996, CitationChang et al. 2007). This was attributed to the fact that the used polymer and the type of the immobilization were different. Additionally, immobilized enzymes can show high activity under temperatures different than physiological conditions compared to that of free enzymes. High optimum temperature was also seen in biosensors which were prepared from PPy-PVS polymers (CitationÇolak et al. 2012, CitationÖzdemir et al. 2012, CitationDolmacı et al. 2012).
Substrate concentration and calibration curves
The effect of the substrate concentration on the reaction rate, catalyzed by immobilized enzyme, was studied by using varying concentrations 1.0 × 10− 9 × 1.0 × 10− 3 M Glu (). There were three linear parts in the region between 1.0 × 10− 9 and 1.0 × 10− 8 M (R2 = 0.847) (), 5.0 × 10− 8 and 5.0 × 10− 7 M (R2 = 0.997) (), and 5.0 × 10− 7 and 5.0 × 10− 5 M (R2 = 0.994) (). When compared with the literature (1.0 × 10− 7 × 1.0 × 10− 4 M and 8.0 × 10− 6 × 8.0 × 10− 4 M, respectively, for CitationKaryakin et al. 2000 and CitationGhobadi et al. 1996), linear working range of biosensor is wider and biosensor is sensitive to low Glu concentration. It has been shown that the linearity of these graphs is highly satisfactory and can be used for the quantitative determination of Glu.
Figure 8. (A) The effect of Glu concentration on the response of the biosensor (at 0.1 M pH 9.0 glycine buffer, 25°C, 0.3 V operating potential). (B) The calibration curve of Glu biosensor (at 0.1 M, pH 9.0 glycine buffer, 25°C). (C) The calibration curve of Glu biosensor (at 0.1 M, pH 9.0 glycine buffer, 25°C). (D) The calibration curve of Glu biosensor (at 0.1 M, pH 9.0 glycine buffer, 25°C).
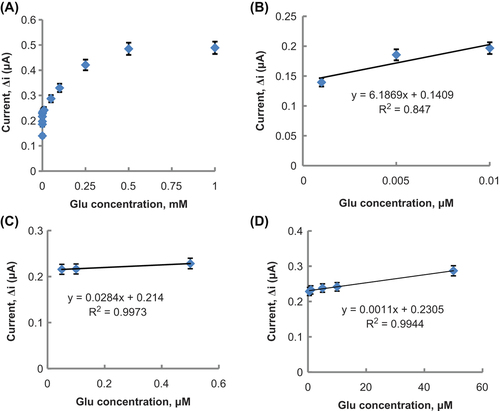
The low detection limit of the biosensor was found to be 5.0 × 10− 9 M and response time for the biosensor was 200 s. Detection limit of biosensor is lower than detection limit in literature (2.0 × 10− 5 M and 1.0 × 10− 7 M, respectively, for CitationMaalouf et al. 2007 and CitationKaryakin et al. 2000). Because of the reasons that prepared Glu biosensor has a wide linear working range which includes low concentrations and determination limit, Glu determination can be made with a variety of different samples with our biosensor. Km(app) and Imax(app) values were found to be 1.1 × 10− 6 mM and 0.268 μA, respectively. The Km(app) value that shows the affinity of the biosensor was 1.1 × 10− 6 mM and the values, cited in the literature, were 1.92 mM (CitationBasu et al. 2006) and 2.84 mM (CitationMaalouf et al. 2007). PPy-PVS film, used in this study, increased the affinity of enzymes to Glu. It can be understood from the Km(app) value which is lower than the Km(app) values in literature.
Operational stability of the Glu biosensor
In order to test the operational stability of the prepared enzyme electrode, the current changes, obtained after subsequent usage, were plotted against the number of measurements. At the end of the 20 measurements, the biosensor had lost 4.0% of its initial activity. The relative standard deviation, obtained after 20 measurements at a constant Glu concentration of 5.0 × 10− 6 M, was found to be 4.4% ().
Storage stabilization of the enzyme electrode
Response of the enzyme electrode, prepared under optimum conditions, was measured for a period of 42 days at constant Glu concentration (1.0 × 10− 3 M). Results of 13 measurements during this period are plotted in . There was no noticeable decrease in the response during the first 3 days. There was a rapid decrease in current values between the third and twenty-fourth days and there was not a significant change after the twenty-fourth day. The electrode showed 25.5% of the initial amperometric response at the end of the 42 days.
Interference effects
A few common substances, found in serum, were investigated with Pt/PPy-PVS film electrode (without immobilizing enzyme) for any interfering effect. The response current for a constant concentration of H2O2 (1.9 × 10− 4 M) was compared with the current value, obtained in the presence of the variable concentrations of the interfering species. These interferants and their concentrations were ascorbic acid (1.0 × 10− 4 M), uric acid (3.0 × 10− 4 M), paracetamol (1.0 × 10− 4 M), and fructose (8.0 × 10− 5 M). The results indicated that in the presence of ascorbic acid, uric acid, paracetamol, and fructose, the response current was increased by 40.0, 70.0, 10.0, and 3.0%, respectively. These interferences were almost removed by dilution of solution in cell. There was not any interference effect of these compounds with 6-fold dilution. The aim of diluting was to eliminate interference (CitationPerez et al. 2003, CitationÇete et al. 2006).
Glu determination in synthetic blood sample
A synthetic blood sample that contains body concentrations of some interferants was prepared. These interferants (and their concentrations) were ascorbic acid (1.0 × 10− 4 M), uric acid (3.0 × 10− 4 M), paracetamol (1.0 × 10− 4 M), and fructose (8.0 × 10− 5 M). Glu concentration was kept as 1.9 × 10− 4 M. The synthetic blood sample was added into a cell and diluted 6-fold. By using the calibration curve, Glu amount determined after three experiments was (1.90 ± 0.024) × 10 − 4 M.
Conclusions
In this study, we report a new amperometric biosensor for the determination of Glu. L-GlOx was immobilized onto a PPy-PVS film surface by cross-linking with GA and BSA. In this study, prepared Glu biosensor can be used in a wide concentration range. It has a very low detection limit and an acceptable response time for a biosensor. The interference effects of different interferants were not observed after dilution. Therefore, Glu determination can be made in a variety of different samples with our biosensor. It was seen that the Glu biosensor was highly sensitive, selective and its operational stability were found to be very good. Thus, Glu determination can be made with our biosensor for many times. In this study, it is found that Glu biosensor is easy to prepare and is highly cost effective. This composite (PPy-PVS) can supply a biocompatible and electrochemical microenvironment for immobilization of the enzyme, making this material a good candidate for the fabrication of highly sensitive and selective Glu biosensors.
Declaration of interest
The authors report no conflicts of interest. The authors alone are responsible for the content and writing of the paper.
The authors are very grateful for the financial support of Gazi University Research Fund (Project no: 05/2013-05).
References
- Amine A, Mohammadi H, Bourais I, Palleschi G. 2006. Enzyme inhibition-based biosensors for food safety and environmental monitoring. Biosens Bioelectron. 21:1405–1423.
- Arslan H, Arslan F. 2011. Preparation of a polypyrrole- polyvinylsulphonate composite film biosensor for determination of phenol based on entrapment of polyphenol oxidase. Artif Cells Nanomed Biotechnol. 39:341–345.
- Basu A.K, Chattopadhyay P, Roychudhuri U, Chakraborty R. 2006. A biosensor based on co-immobilized l-glutamate oxidase and l-glutamate dehydrogenase for analysis of monosodium glutamate in food. Biosens Bioelectron. 21:1968–1972.
- Chang K-S, Chang C-K, Chou S-F, Han H-C, Chen C-Y. 2007. Characterization of a planar l-glutamate amperometric biosensor immobilized with a photo-crosslinkable polymer membrane. Sens Actuators B Chem. 122:195–203.
- Chapman J, Zhou M. 1999. Microplate-based fluorometric methods for the enzymatic determination of l-glutamate: application in measuring l-glutamate in food samples. Anal Chim Acta. 402:47–52.
- Chaubey A, Gerard M, Singhal M, Singh VS, Malhotra BD. 2000. Immobilization of lactate dehydrogenase on electrochemically prepared polypyrrole-polyvinylsulphonate composite films for application to lactate biosensors. Electrochim Acta. 46:723–729.
- Cooper JM, McNeil CJ, Spoors JA. 1991. Amperometric enzyme electrode for the determination of aspartate aminotransferase and alanine aminotransferase in serum. Anal Chim Acta. 245:57–62.
- Çete S, Yaşar A, Arslan F. 2006. An amperometric biosensor for uric acid determination prepared from uricase immobilized in polypyrrole film. Artif Cell Blood Sub. 34:367–380.
- Çolak Ö, Yaşar A, Çete S, Arslan F. 2012. Glucose biosensor based on the immobilization of glucose oxidase on electrochemically synthesized polypyrrole-poly(vinyl sulphonate) composite film by cross-linking with glutaraldehyde. Artif Cells Nanomed Biotechnol. 40:354–361.
- Dolmacı N, Çete S, Arslan F, Yaşar A. 2012. An amperometric biosensor for fish freshness detection from xanthine oxidase immobilized in polypyrrole-polyvinylsulphonate film Artif Cell Blood Sub. 40:275–279.
- Ghobadi S, Csöregi E, Marko-Varga G, Gorton L. 1996. Bienzyme carbon paste electrodes for L-glutamate determination. Curr Sep. 14:94–102.
- Gros P, Durliat H, Comtat M. 2000. Use of polypyrrole film containing Fe(CN)63− as pseudo-reference electrode: application for amperometric biosensors. Electrochim Acta. 46:643–650.
- Janarthanan C, Mottola HA. 1998. Enzymatic determinations with rotating bioreactors: Determination of glutamate in food products. Anal Chim Acta. 369:147–155.
- Karyakin AA, Karyakina EE, Gorton L. 2000. Amperometric biosensor for glutamate using prussian blue-based “Artificial Peroxidase” as a transducer for hydrogen peroxide. Anal Chem.72:1720–1723.
- Maalouf R, Chebib H, Saϊkali Y, Vittori O, Sigaud M, Jaffrezic-Renault N. 2007. Amperometric and impedimetric characterization of a glutamate biosensor based on Nafion® and a methyl viologen modified glassy carbon electrode. Biosens Bioelectron. 22:2682–2688.
- Nelson DL, Cox MM. 2000. Lehninger Principles of Biochemistry. New York: Worth Publishers.
- Özdemir M, Arslan F, Arslan H. 2012. An amperometric biosensor for choline determination prepared from choline oxidase immobilized in polypyrrole-polyvinylsulfonate film. Artif Cell Blood Sub. 40:280–284.
- Pan S, Arnold MA. 1996. Selectivity enhancement for glutamate with a Nafion/glutamate oxidase biosensor Talanta. 43:1157–1162.
- Perez DM, Ferrer ML, Mateo CR. 2003. A reagent less fluorescent sol-gel biosensor for uric acid detection in biological fluids. Anal Biochem. 322:238–242.
- Swanepoel E, de Villiers MM, du Preez JL. 1996. Fluorimetric method of analysis for d-norpseudoephedrine hydrochloride, glycine and l-glutamic acid by reversed-phase high-performance liquid chromatography. J Chromatogr A. 729:287–291.
- Tucci S, Pinto C, Goyo J, Rada P, Hernandez L. 1998. Measurement of glutamine and glutamate by capillary electrophoresis and laser induced fluorescence detection in cerebrospinal fluid of meningitis sick children. Clin Biochem. 31:143–150.
- Valero E, Garcia-Carmona F. 1998. A continuous spectrophotometric method based on enzymatic cycling for determining l-vglutamate. Anal Biochem. 259:265–271.
- Xin Q, Wightman RM. 1997. Enzyme modified amperometric sensors for choline and acetylcholine with tetrathiafulvalene tetracyanoquinodimethane as the electron-transfer mediator. Anal Chim Acta. 341:43–51.
- Zhang Y, Wen G, Zhou Y, Shuang S, Dong C, Choi MM. 2007. Development and analytical application of an uric acid biosensor using an uricase-immobilized eggshell membrane. Biosens Bioelectron. 22:1791–1797.