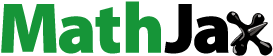
Abstract
Many studies of non-supported bimetallic nanoparticle (BMNP) dispersions, stabilized by ligands or polymers, and copolymers, were started only about 10 years ago. Several preparative procedures have been proposed, and full characterizations on BMNPs have been approved. Studies on BMNPs received huge attention from both scientific and technological communities because most of the NPs’ catalytic activity depends on their structural aspects.
In this study, we focus on the preparation, properties, and bio-application of BMNPs and introduction of the recent advance in these NPs.
Introduction
Bimetallic nanoparticles (BMNPs) involving Au–Pd, Au–Ag, and Au–Pt have been produced in a single step by a sol–gel process and stabilized in liquid and solid matrix. BMNPs have four types of integration patterns: core–shell NPs, subcluster NPs, alloy NPs, and multishell NPs (CitationTurkevich et al. 1951). Alloying of metals is a method of developing novel materials that have technological usefulness than their starting substances. Alloy NPs show various structural and physical properties than bulk samples (CitationCouchman and Jesser 1977, CitationCeylan et al. 2006).
Ongoing extensive studies on non-supported BMNP dispersions, stabilized by polymers or ligands, were started only 10 years ago. Studies on BMNPs received huge attention from both scientific and technological communities, because most of the NPs’ catalytic activity depends on their structural aspects (CitationToshima and Yonezawa 1998). In bulk metals, atoms are arranged in diverse geometries, each metal having its own atomic position. The follow-on crystal structure is typically simple and depends on the identity of the metal and other factors such as temperature. BMNPs can exist in another type of structure, in which the distribution of each metal element is not the same as that found in the bulk (CitationThulasiramaraju et al. 2014). Layered core− shell silver− gold BMNPs were arranged by coating Au layers over Ag seeds using a seed-growth technique. The arrangement of Ag100-xAux particles can vary from x = 0 to 30 (CitationCui et al. 2006). Nano-sized materials serve as an ideal candidate for diverse applications due to their extraordinarily small size and correspondingly large surface-to-volume ratio. Additionally, their properties may be modified by changing their size, shape, and composition using synthetic methods (CitationVan Hyning and Zukoski 1998, CitationAdair and Suvaci 2000, CitationJana et al. 2001, CitationVelikov et al. 2003, CitationD’Souza et al. 2004).
Synthesis
Several studies show that the stability and size of nanoscale colloidal particles effectively depend on the technique and the experimental conditions followed. The novel Ag/Ag–Au BMNPs were produced by the replacement reaction between Ag NPs and HAuCl4. Au–Ag NPs were synthesized by method of reduction of changeable mole fractions of HAuCl4 and AgNO3 using sodium borohydride in the presence of sodium citrate, in water .The exchange of Ag-NPs into Ag/Ag–Au BMNPs involved numerous sequential processes (CitationLu et al. 2007, CitationChen et al. 2006, CitationPark et al. 2009):
oxidative dissolution of Ag atoms,
reduction of AuCl− 4, and
deposition of Au atoms.
Metal NPs can be produced in two different ways, that is, by subdivision of bulk metals (a physical method) and by the growth of particles obtained from metal atoms, which are from molecular or ionic precursors (a chemical method) ().
Core–shell
Core–shell and multishell Au–Ag BMNPs have been produced by successive reduction of metal salts with ascorbic acid on pre-made seeds in the presence of a cationic surfactant, cetyltrimethylammonium bromide. In thickness-proscribed synthesis of core–shell structured Au/Ag or Ag/Au NPs, the external metal surface can be tuned as a performance of the internal metal surface, provided that the outer shell is thin enough (CitationRodriguez-Gonzalez et al. 2005, CitationCao et al. 2001, CitationRivas et al. 2000, CitationDaniel and Astruc 2004, CitationFerrer et al. 2007, CitationKim et al. 2005) ().
Figure 1. Schematic picture showing the shape and composition progress of Ag/Ag–Au metal core/alloy shell BMNPs. The green and cyan planes signify the {111} and {100} forms of silver, respectively; the yellow and blue planes denote the {111} and {100} forms of gold–silver alloy and pure gold, respectively (CitationZhang et al. 2008).
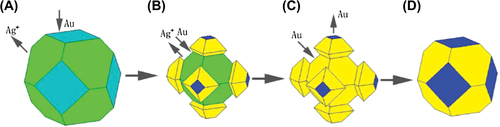
Co-reduction
The co-reduction of Au and Ag precursors is the simplest method of preparing Au–Ag alloy NPs. Since two metal precursors are involved in the reduction reactions, the influence of synthesis conditions on the rates of precursor reduction, and the nucleation and growth of the alloy NPs are more complex than in the case of monometallic NPs (CitationWang et al. 2009, CitationWilson et al. 2005, CitationKim et al. 2003, CitationMallin and Murphy 2002, CitationChen and Chen 2002, CitationLink et al. 1999, CitationHostetler et al. 1998, CitationHan et al. 1998).
Biogenic synthesis
One of the biological synthesis methods for Au–Ag NPs is by using the leaf extract of S. mahogani Jacq. The role of the leaf extract is reduction and stabilization of Au and Ag for the quick arrangement of stable metal NPs with different compositions, shapes, sizes, and also with high monodispersities. Au/Ag BMNPs produced by this technique have prospect for biomedical applications in the future (CitationMondal et al. 2011).
Laser-assisted synthesis of Au–Ag alloy NP
One of the simple and convenient methods for synthesizing Au–Ag BMNPs is “laser irradiation” method, which is a bottom-up approach comparable with the approach of laser ablation of bulk materials in solution for producing NPs. Spherical shapes and crystallized NPs can effortlessly be obtained in one-step procedures with no succeeding heat-treatments, due to high energetic state of irradiated species without production of by-products (CitationTakami et al. 1999) ().
Replacement reactions
Replacement reaction is a simple method to prepare Ag–Au BMNPs, which occurs between Ag-NPs and HAuCl4 at elevated temperatures (CitationSun et al. 2002, CitationLiang et al. 2005, CitationSun and Xia 2004).
This technique takes advantage of the rapid interdiffusion of Au and Ag atoms in the reduced dimension of NPs, high temperature of the process, and the plenty of interfacial vacancy defects created by the reaction.
The main advantage of this method is that the size and composition of the alloy NPs are separately tunable and that the particles can be formed in high concentrations (good process scalability) (CitationZhang et al. 2007).
Replacement Reaction:
The produced atomic gold would then alloy with the unreacted silver under suitable situation to form homogeneous alloy NPs (CitationLink et al. 1999) ().
Figure 3. Synthesis of core/alloy shell NPs by the replacement reaction between Ag NPs and HAuCl4. (CitationZhang et al. 2008).
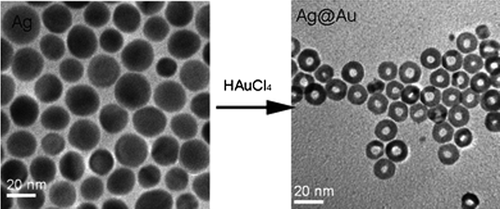
Properties
Properties of BMNPs are influenced by both the metals; they provide excessive ordinary metallic NPs, which is an advantage (CitationMohl et al. 2011, CitationHuang et al. 2006). The properties of alloy NPs can be extremely different from those of the elemental monometallic nanoparticles (CitationMohamed et al. 2000).
Optical properties of Au–Ag
Plasmonic coupling between NPs is one of the most interesting optical properties; the characteristic improvement of local optical field at particle–particle interface is extremely useful for numerous sensing applications (CitationLee and El-Sayed 2006, CitationWang et al. 2007). Monometallic Ag and Au NPs have relatively monotonous optical properties due to surface plasmon resonance (SPR); the SPR properties of Ag–Au alloy NPs are incessantly tunable because of the possibility of composition changes. SPR excitation within the gold and silver nanostructures greatly enhances the local electric field (CitationCheng et al. 2008, CitationWang et al. 2006). The absorption and dispersion of light in NPs rely on the characteristic of the metals, including their chemical composition, morphology, and size. NPs of noble metals, for instance gold and silver, with a size smaller than the wavelength of visible light powerfully scatter and absorb light because of SPR. Au–Ag BMNPs show diverse optical responses for alloy and core–shell configurations, even when they have the same Au and Ag contents (CitationKim et al. 2005, CitationKreibing and Vollmer 1995).
Antibacterial properties
Au–Ag BMNPs show high-quality antibacterial activity against Gram-positive bacteria Staphylococcus aureus and Gram-negative bacteria Klebsiella pneumoniae. BMNPs at the concentration of 1:3 exhibit areas of inhibition against the pathogenic bacteria, for example, Staphylococcus aureus and Klebsiella pneumoniae (CitationRamakritinan et al. 2013).
Catalytic properties
A novel group of materials for catalysis have been densely studied, that is, “bimetallic nanoparticles”. Metal NP catalysts comprising two (or more) different metal components have received attention from both technological and scientific points of view for improving the quality or properties of catalyst (CitationMenezes et al. 2013).
Although the size of Au-NPs is smaller than that of Ag-NPs and AuAg-NPs, equal atomic concentrations of Au-NPs, Ag-NPs, and AuAg-NPs are applied for the catalytic reaction due to their enhanced surface areas and decreased densities (CitationShin et al. 2012).
Applications
Photothermal cancer therapy
Au–Ag BMNPs can be used as nanomaterials in several in vitro and in vivo cancer therapies, Raman scattering development, and catalytic reactions using extremely well-organized photothermal ablation, large resistive heat generation, and tunable near-IR absorption (CitationLiu et al. 2014). Ru-Shi Liu et al. found that oral cancer cells without NPs were not hurt after exposure to laser irradiation for 5 min (see ). On the contrary, cells with added NPs experienced significant cell death after laser exposure for 1 min (see and ).
Catalytic application
The application of BMNPs as catalysts is one of the most active areas of nanoscience (CitationDiscuss and Jellinek 2008) . BMNPs act as catalysts in chemical reactions and in environmental remediation. Differential metal components of Au with other metals, such as Ag, Cu, Pt, and Pd, were found to have reduced put on compared with that of net Au associates at the expense of higher cost (CitationXiong and Manthiram 2005, CitationShah et al. 2012) ().
Electroplating and fabrication
BMNPs allow more than one metal to be used for establishing contact, with or without the expense and complexity of integrating Au alloy into the microelectromechanical systems) switch fabrication process (CitationMallin and Murphy 2002, CitationWang et al. 2006).
Potential applications
BMNPs surrounding the surface regions of glass are of great interest because of their potential application (CitationKim et al. 2007, CitationWiederrecht 2004). Ag–Au NPs in silica glasses with linear and nonlinear optical properties have been achieved (CitationDitlbacher et al. 2000).Later, nonlinear field enrichment relying on the laser polarization of single Ag nanobars and nanorice was also reported (CitationWiley et al. 2007).
Thermal conductivity
It has been demonstrated that nanofluids, consisting of Ag–Au in water, enhances the thermal conductivity of the fluids (CitationZhong et al. 2006, Citationvan Dijk 2007).
Conclusion
The BMNPs with core–shell structures can offer unique physical and optical properties inaccessible to monometallic systems. These nanoparticles have been utilized in many areas of research including chemical catalysis, surface-enhanced Raman spectroscopy, and photothermal therapy. This review article provides a comprehensive overview of BMNP systems consisting of gold and silver; it is based on the recent advances in wet-chemical synthetic methodologies, the characterization of size- and shape-dependent optical properties, and various optically driven applications including catalysis, signal-enhancing devices, and biomedical purposes.
Authors’ Contributions
EA and HTN conceived the study and participated in its design and coordination. AA, SD, and MK participated in the sequence alignment and drafted the manuscript. All authors read and approved the final manuscript.
Acknowledgments
The authors thank the Department of Medical Nanotechnology and the Faculty of Advanced Medical Science of Tabriz University for all their supports.
Declaration of interest
The authors report no declarations of interest. The authors alone are responsible for the content and writing of the paper.
This work is funded by 2014 Drug Applied Research Center Tabriz university of Medical Sciences Grant.
References
- Adair JH, Suvaci E. 2000. Morphological control of particles. Curr Opin Colloid Interface Sci. 5:160–167.
- Cao YW, Jin R, Mirkin CA. 2001. DNA-modified core–shell Ag/Au nanoparticles. J Am Chem Soc. 123:7961–7962.
- Ceylan A, Jastrzembski K, Shah SI. 2006. Enhanced solubility Ag-Cu nanoparticles and their thermal transport properties. Metall Mater Trans A. 37:2033
- Chen DH, Chen CJ. 2002. Formation and characterization of Au–Ag bimetallic nanoparticles in water-in-oil microemulsions. J Mater Chem. 12:1557–1562
- Chen JY, McLellan JM, Siekkinen A, Xiong YJ, Li ZY, Xia YN. 2006. Synthesis of Ag@ AgAu metal core/alloy shell bimetallic nanoparticles with tunable shell compositions by a galvanic replacement reaction. J Am Chem Soc. 128:14776–14777.
- Cheng MT, Liu SD, Wang QQ. 2008. Modulating emission polarization of semiconductor quantum dots through surface plasmon of metal nanorod. Appl Phys Lett. 92:162–107.
- Couchman, P. R., Jesser, W. A. 1977. Thermodynamic theory of size dependence of melting temperature in metals. J Nat Products. 269:481.
- Cui Y, Ren B, Yao JL, Gu RA, Tian ZQ. 2006. Synthesis of Ag core Au shell bimetallic nanoparticles for immunoassay based on surface-enhanced Raman spectroscopy. J Phys Chem B. 110:4002–4006.
- D’Souza L, Suchopar A, Richards RM. 2004. In situ approaches to establish colloidal growth kinetics. J Colloid Interface Sci. 279:458–463
- Daniel MC, Astruc D. 2004. Gold nanoparticles: assembly, supramolecular chemistry, quantum-size-related properties, and applications toward biology,catalysis, and nanotechnology. Chem Rev. 104:293–346.
- Discuss F, Jellinek J. 2008. Nanoalloys: tuning properties and characteristics through size and composition138:11–35.
- Ditlbacher H, Krenn JR, Lamprecht B, Leitner A, Aussenegg FR. 2000. Optical properties of Ag and Au nanowire gratings. Opt Lett. 25:560
- Ferrer D, Torres-Castro A, Gao X, Sepulveda-Guzman S, Ortiz- Mendez U, Jose-Yacaman M. 2007. Three-layer core/shell structure in Au–Pd bimetallic nanoparticles. Nano Lett. 7:1701–1705.
- Han SW, Kim Y, Kim K. 1998. Dodecanethiol-derivatized Au/Ag bimetallic nanoparticles: TEM, UV/VIS, XPS, and FTIR analysis. J. Colloid Interface Sci. 208:272–278
- Hostetler MJ, Zhong CJ, Yen BKH, Anderegg J, Gross SM, Evans ND, et al. 1998. Stable, monolayer-protected metal alloy clusters. J. Am. Chem. Soc. 120:9396–9397
- Huang Q, Yang H, Tang Y, Lu T, Akins DL. 2006. Carbon-supported Pt–Co alloy nanoparticles for oxygen reduction reaction. Electrochem Commun. 8:1220.
- Jana NR, Gearheart L, Murphy CJ. 2001. Evidence for seedmediated nucleation in the chemical reduction of gold salts to gold nanoparticles. Chem Mater. 13:2313–2322.
- Kim JH, Ishihara A, Mitsushima S, Kamiya N, Ota KI. 2007. Catalytic activity of titanium oxide for oxygen reduction reaction as a non-platinum catalyst for PEFC. Electrochim Acta. 52:2492.
- Kim K, Kim KL, Lee SJ. 2005. Surface enrichment of Ag atoms in Au/Ag alloy nanoparticles revealed by surface enhanced Raman scattering spectroscopy. Chem Phys Lett. 403:77–82.
- Kim MJ, Na HJ, Lee KC, Yoo EA, Lee MY. 2003. Preparation and characterization of Au–Ag and Au–Cu alloy nanoparticles in chloroform. J Mater Chem. 13:1789–1792
- Kreibing U, Vollmer M. 1995. Optical Properties of Metal Clusters, vol. 25. Berlin, Germany: Springer .
- Lee K-S, El-Sayed MA. 2006. Gold and silver nanoparticles in sensing and imaging: sensitivity of Plasmon response to size, shape, and metal composition. J Phys Chem B. 110:19220–19225.
- Liang HP, Wan LJ, Bai CL, Jiang L. 2005. Gold hollow nanospheres: tunable surface plasmon resonance controlled by interior-cavity sizes. J Phys Chem B. 109:7795.
- Link S, Wang ZL, El-Sayed MA. 1999. Alloy formation of gold-silver nanoparticles and the dependence of the plasmon absorption on their composition. J Phys Chem B. 103:3529–3533.
- Liu R-S, Cheng L-C, Huang J-H, Chen HM, Lai T-C, et al. 2014. Highly efficient urchin-like bimetallic nanoparticles forphotothermal cancer therapy. NEWS ROOM. doi:https://doi.org/10.1117/2.1201301.004676. Page 2/3.
- Lu XM, Tuan HY, Chen JY, Li ZY, Korgel BA, Xia YN. 2007. Mechanistic studies on the galvanic replacement reaction between multiply twinned particles of Ag and HAuCl4 in an organic medium. J Am Chem Soc. 129:1733–1742.
- Mallin MP, Murphy CJ. 2002. Solution-phase synthesis of sub-10 nm Au-Ag alloy nanoparticles. Nano Lett. 2:1235–1237.
- Menezes WG, Neumann B, Zielasek V, Thiel K, äumer MB. 2013. Bimetallic AuAg nanoparticles: Enhancing the catalytic activity of Au for reduction reactions in the liquid phase by addition of Ag. Chem. Phys. Chem. 14(8):1577–1581.
- Mohamed MB, Volkov V, Link S, El-Sayed MA. 2000. The lightning gold nanorods: fluorescence enhancement of over a million compared to the gold metal. Chem Phys Lett. 17:517–523.
- Mohl M, Dobo D, Kukovecz A, Konya Z, Kordas K, Wei J, et al. 2011. Formation of CuPd and CuPt bimetallic nanotubes by galvanic replacement reaction. J Phys Chem C. 115:9403.
- Mondal S, Roy N, Laskara RA, Ska I, Basu S, Mandal D, Begum NA. 2011. Biogenic synthesis of Ag, Au and bimetallic Au/Ag alloy nanoparticles using aqueous extract of mahogany (Swietenia mahogani JACQ.), leaves. Colloids Surf B Biointerfaces. 82:497–504.
- Park J, Zheng H, Jun YW, Alivisatos AP. 2009. Hetero-epitaxial anion exchange yields single-crystalline hollow nanoparticles. J Am Chem Soc. 131:13943–13945.
- Ramakritinan CM, Kaarunya E, Shankar S, Kumaraguru AK. 2013. Antibacterial effects of Ag, Au and bimetallic (Ag-Au) nanoparticles synthesized from red Algae. Solid State Phenomena. 201:211–230.
- Rivas L, Sanchez-Cortes S, Garcia-Ramos JV, Morcillo G. 2000. Mixed silver/gold colloids: a study of their formation, morphology, and surface-enhanced Raman activity. Langmuir. 16:9722–9728.
- Rodriguez-Gonzalez B, Burrows A, Watanabe M, Kiely CJ, Liz-Marzán LM. 2005. Multishell bimetallic AuAg nanoparticles: synthesis, structure and optical properties. J Mater Chem. 15: 1755–1759.
- Shah A, Latif-ur-Rahman, Qureshi R, Zia-ur-Rehman. 2012. Synthesis, characterization and applications of bimetallic (Au-Ag, Au-Pt, Au-Ru) alloy nanoparticles. Rev Adv Mater Sci. 30:133–149.
- Shin KS, Kim JH, Kim IH, Kim K. 2012. Poly(ethylenimine)-stabilized hollow gold-silver bimetallic nanoparticles: fabrication and catalytic application. Bull Korean Chem Soc. 33.
- Sun YG, Mayers BT, Xia YN. 2002. Template-engaged replacement reaction: a one-step approach to the large-scale synthesis of metal nanostructures with hollow interiors. Nano Lett. 2:481.
- Sun YG, Xia YN. 2004. Mechanistic study on the replacement reaction between silver nanostructures and chloroauric acid in aqueous medium. J Am Chem Soc. 126:3892–3901.
- Takami A, Kurita H, Koda S. 1999. Laser-induced size reduction of noble metal particles. J Phys Chem B. 103:1226–1232.
- Thulasiramaraju TV, Arunachalam A, SurendraBabu GV. 2014. Bimetallic nanoparticles-a review on their preparation and characterization.
- Toshima N, Yonezawa T. 1998. Bimetallic nanoparticles novel materials for chemical and physical applications. New J Chem. 22:1179.
- Turkevich J, Stevenson PC, Hillier J. 1951. Direct electrochemistry of horseradish peroxidase immobilized on a colloid cysteamine-modified gold electrode. Disc Faraday Soc. 11:55.
- van Dijk M. 2007. Nonlinear optical studies of single gold nanoparticles. Ph.D. dissertation (Universiteit Leiden).
- Van Hyning DL, Zukoski CF. 1998. Formation mechanisms andaggregation behavior of borohydride reduced silver particles. Langmuir. 14:7034–7046.
- Velikov KP, Zegers GE, van Blaaderen A. 2003. Synthesis and characterization of large colloidal silver particles. Langmuir. 19:1384–1389
- Wang A, Hsieh YP, Chen YF, Mou CY. 2006. Au–Ag alloy nanoparticle as catalyst for CO oxidation: Effect of Si/Al ratio of mesoporous support. J Catal. 237:197.
- Wang C, Yin H, Chan R, Peng S, Dai S, Sun S. 2009. One-pot synthesis of Oleylamine coated AuAg alloy NPs and their catalysis for CO oxidation. Chem Mater. 21:433–435.
- Wang H, Brandl DW, Le F, Nordlander P, Halas NJ. 2006. Nanorice: a hybrid plasmonic nanostructure. Nano Lett. 6:827–832.
- Wang L, Shi X, Kariuki NN, Schadt M, Wang GR, Rendeng Q, et al. 2007. Array of molecularly mediated thin film assemblies of nanoparticles: correlation of vapor sensing with interparticle spatial properties. J Am Chem Soc. 129:2161–2170.
- Wiederrecht GP. 2004. Near-field optical imaging of noble metal nanoparticles. Eur Phys J Appl Phys. 28:3.
- Wiley BJ, Chen Y, McLellan JM, Xiong Y, Li Z-Y, Ginger D, Xia Y. 2007. Synthesis and optical properties of silver nanobars and nanorice. Nano Lett. 7:1032–1036.
- Wilson OM, Scott RWJ, Garcia-Martinez JC, Crooks RM. 2005. Synthesis, characterization, and structure-selective extraction of 1-3-nm diameter AuAg dendrimer-encapsulated bimetallic nanoparticles. J Am Chem Soc. 127:1015–1024.
- Xiong L, Manthiram A. 2005. Nanostructured Pt–M/C (M = Fe and Co) catalysts prepared by a microemulsion method for oxygen reduction in proton exchange membrane fuel cells. Electrochim Acta. 50:2323.
- Zhang Q, Lee JY, Yang J, Boothroyd C, Zhang J. 2007. Size and composition tunable Ag–Au alloy nanoparticles by replacement reactions. Nanotechnology. 18:8.
- Zhang Q, Xie J, Lee JY, Zhang J, Boothroyd C. 2008. Synthesis of Ag@AgAu metal core/alloy shell bimetallic nanoparticles with tunable shell compositions by a galvanic replacement reaction. Small. 4:1067–1071.
- Zhang QB, Xie JP, Lee JY, Zhang, JX, Boothroyd C. 2008. Monodisperse icosahedral Ag, Au, and Pd nanoparticles: size control strategy and superlattice formation. Small. 4:1067–1071.
- Zhong H, Zhang H, Liu G, Liang Y, Hu B. 2006. A novel non-noble electrocatalyst for PEM fuel cell based on molybdenum nitride. J Electrochem Commun. 8:707.