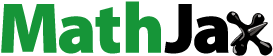
Abstract
Breast cancer is the most commonly diagnosed cancer and the leading cause of cancer death among women worldwide. Herbal medicines have tremendous potential as promising agents for the treatment of cancer. Curcumin is a natural polyphenol which has many anticancer effects. Because of its low aqueous solubility, low bioavailability, and quick degradation and metabolism, curcumin was released using PLGA-PEG nanoparticles. Herein, the efficiency of pure curcumin and curcumin-loaded PLGA-PEG in MCF-7 human breast cancer cell lines was studied. 1H NMR, FT-IR and SEM demonstrated PLGA-PEG structure and curcumin loaded on nanoparticles. Subsequently, the cytotoxic effects of free curcumin and curcumin-loaded PLGA-PEG were determined via an MTT assay. Our study confirmed that curcumin-loaded PLGA-PEG has more cytotoxic effects on the MCF-7 breast cancer cell line and could be exploited as a potential source for developing novel drugs against breast cancer.
Keywords::
Introduction
With one million new cases in the world annually, breast cancer is the most commonly diagnosed cancer and the leading cause of cancer death in women worldwide, accounting for 23% (1.38 million) of all new cancer cases and 14% (458,400) of the all cancer deaths in 2008. Calculations show that nearly half the breast cancer cases and 60% of the deaths appear in economically developing countries (CitationMcPherson et al. 2000, CitationJemal et al. 2011). Factors that have an effect on a woman's risk of breast cancer comprise geographical variation, age at menarche and menopause, age at first pregnancy, family history, radiation, previous benign breast disease, lifestyle (such as diet, weight, alcohol intake, and smoking), use of oral contraceptive and hormone replacement therapy (CitationMcPherson et al. 2000). When cancer is diagnosed, a variety of probable treatment options are considered. Three fundamental strategies are employed in the treatment of cancer: radiotherapy, surgery and chemotherapy, each of which could be used alone or in combination with the other/s. Systemic chemotherapy is generally used to treat the metastatic types of neoplastic disease. Chemotherapeutic agents are divided into two main groups: synthetic and natural products. In spite of immense progress in the field of organic chemistry, 25% of all prescription drugs are currently derived from natural sources. This is more important with regard to anti-cancer drugs, of which more than 80% are plant-derived compounds (CitationSadeghi-Aliabadi and Ahmadi 2000). Herbal medicines have been proven to be a significant source of novel agents with a pharmaceutical potential. Various anticancer drugs currently in use are either natural products or are derived from natural products. Herbal medicines have tremendous potential as promising agents for the treatment of cancer. Natural products have traditionally supplied a rich source of drugs for numerous diseases including cancer, and plants are a significant source of novel natural products (CitationRasul et al. 2012). A dynamic component of the perennial herb Curcuma longa (commonly known as turmeric), curcumin, is a yellow-colored polyphenol and a natural plant phenolic food additive. Curcumin demonstrates anti-cancer activities both in vitro and in vivo by diverse mechanisms. It hinders proliferation and induces apoptosis in a wide range of cancer cell types in vitro, including cells from cancers of the breast, bladder, lung and other tissues (CitationZhou et al. 2011). Owing to its meager aqueous solubility, low bioavailability, and quick degradation and metabolism, the use of curcumin for treatment is limited in animals and humans. To conquer these problems, different kinds of nano-preparations of this natural product have been developed (CitationLiu et al. 2013). Substantial research is being directed towards developing biodegradable polymeric nanoparticles intended for drug delivery (CitationSingh and Lillard 2009). Poly (lactic-co-glycolic acid) (PLGA) is one of the most effectively used biodegradable polymers. PLGA is approved by the US FDA and the European Medicines Agency (EMA) in diverse drug delivery systems in humans. However, the body distinguishes hydrophobic particles as foreign and the reticulo-endothelial system (RES) removes these from the blood stream and takes them up in the liver or the spleen. This procedure is one of the most significant biological obstacles to nanoparticle-based controlled drug delivery. To address these limitations, a number of methods of surface modifications have been developed to create nanoparticles that are not recognized by the RES. Nanoparticles can be coated with molecules that conceal the hydrophobicity by providing a hydrophilic layer at the surface. The most general method (CitationSadat Tabatabaei Mirakabad et al. 2014b) for surface modification is use of the hydrophilic and non-ionic polymer polyethylene glycol (PEG) (CitationDanhier et al. 2012, CitationSadat Tabatabaei Mirakabad et al. 2014b). The existence of PEG on the PLGA nanoparticles imparts extra functionality through the use of polymeric NPs (CitationMahapatro and Singh 2011).
Herein, we want to demonstrate the efficiency of pure curcumin and curcumin-loaded PLGA-PEG in MCF-7 human breast cancer cell lines. Treatment with curcumin results in growth inhibition and a decrease of growth in the cell culture, and these effects are further enhanced with the use of PLGA-PEG-loaded curcumin nanoparticles. In this study, we investigated the inhibitory effect of pure and PLGA-PEG-loaded curcumin on the MCF-7 human breast cancer cell line in an in vitro model of breast cancer, because we want to discover an efficient and safe approach to improve the current methods of treatment with chemotherapy to counteract this malignancy.
Materials and methods
Material
D,L-lactide and glycolide, stannous octoate (Sn (Oct)2: stannous 2-ethylhexanoate), PEG (molecular weight 6000), dichloromethane (DCM), and polyvinyl alcohol (PVA) were purchased from Sigma-Aldrich (USA). Curcumin powder was also supplied by Sigma-Aldrich. Scanning electron microscopy (SEM) was conducted using the KYKY model EM3200. The drug-loading capacity and release behavior were determined using an ultraviolet visible 2550 spectrometer (Shimadzu, Tokyo, Japan). The infrared spectra were recorded in real-time with a Perkin Elmer series FTIR. The 1H NMR spectra were recorded in real-time with a Brucker DRX 300 spectrometer operating at 300.13 MHz. The samples were homogenized using a homogenizer (SilentCrusher M, Heidolph Instruments GmbH, Schwabach, Germany). The organic phase was evaporated by rotary (Rotary Evaporators, Heidolph Instruments, Hei-VAP series). The MCF-7 cell line (human breast cancer epithelial-like cell line) was prepared from the cell bank of the Pasteur Institute in Iran. This cell line was cultured in RPMI1640 (Gibco, Invitrogen, UK) supplemented with 10% heat-inactivated fetal bovine serum (FBS) (Gibco, Invitrogen, UK), 0.05 mg/mL penicillin G, 2 mg/mL bicarbonate sodium (Serva Co., Germany), 0.08 mg/mL streptomycin (Merck co, Germany), and incubated in 37°C with humidified air containing 5% CO2. Trypsin-EDTCA was obtained from Gibco, Invitrogen (UK), and 3(4,5-dimethylthiazol-2-yl)2,5-diphenyl-tetrazolium bromide (MTT) and dimethyl sulfoxide (DMSO) were all obtained from Sigma-Aldrich (USA).
Synthesis of PLGA-PEG triblock copolymer
PLGA-PEG copolymers (PEG6000) were prepared by a melt polymerization process under vacuum using stannous octoate [Sn(Oct)2: stannous 2-ethylhexanoate] as a catalyst. DL-lactide (1.441 g), glycolide (0.285 g), and PEG6000 (0.77 g) (45%/w) were heated to 140°C in a bottleneck flask, in a nitrogen atmosphere, for complete melting. The molar proportion of DL-lactide and glycolide was 3:1. Then 0.05% (w/w) stannous octoate was added and the temperature of the reaction mixture was increased to 180°C. The temperature was maintained for 4 h. The polymerization was done under vacuum. The copolymer was recovered by dissolving in methylene chloride followed by precipitation in ice-cold diethyl ether. The procedure for synthesis of the PLGA-PEG copolymer is shown in .
Figure 1. (A) Preparation of a triblock copolymer of PLGA-PEG, and (B) mechanism of PLGA-PEG prepared by Sn (Oct)2 as catalyst. PEG, poly (ethylene glycol); PLGA, poly (D, L-lactic-co-glycolic acid).
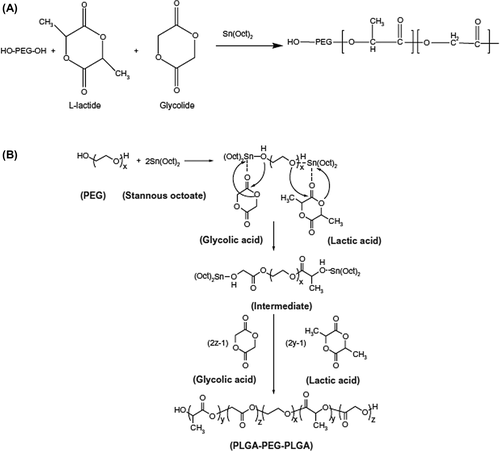
A triblock copolymer of PLGA-PEG was organized by ring opening polymerization of DL-lactide and glycolide in the presence of PEG 6000 (CitationAkbarzadeh et al. 2012d, Citation2012c, CitationEbrahimnezhad et al. 2013, CitationAlimirzalu et al. 2014).
Measurement of copolymer
The 1H NMR spectra were recorded in CDCl3 on a Bruker AM 300.13 MHz spectrometer. The FTIR (Perkin Elmer series) spectrum was obtained from a neat film cast of the chloroform copolymer solution between KBr tablets. SEM was used to determine size and shape of the PLGA-PEG nanoparticles.
Curcumin loading and determination of curcumin encapsulation efficiency
Curcumin, an anticancer drug, was used for the drug loading and release studies. In brief, 120 mg of nanoparticles were dissolved in 3 mL dichloromethane solution. In another test, 20 mg of curcumin was dispersed in 3 mL methanol. Polyvinyl alcohol (PVA) was added to this solution and the whole solution was stirred for 2 mins to allow curcumin to be entrapped within the nanoparticle network. Then, dichloromethane was evaporated using a rotary evaporator and the remaining solution was centrifuged at 9000 rpm for 25 min. The supernatant was isolated and compared with the total amount of curcumin to determine the curcumin loading efficiency of the nanoparticles. The amount of non-entrapped curcumin in aqueous phase was determined using an ultraviolet 2550 (λex 420 nm) spectrophotometer (Shimadzu). This procedure permits the analysis of a curcumin solution with the removal of most interfering substances (CitationHosseininasab et al. 2014, CitationRezaei-Sadabady et al. 2013, CitationNejati-Koshki et al. 2013, CitationGhasemali et al. 2013, CitationMollazade et al. 2013, CitationAkbarzadeh et al. 2014, CitationAkbarzadeh et al. 2013a, CitationAhmadi et al. 2014). The amount of curcumin entrapped within the nanoparticles was calculated by the difference between the total amount used to prepare the nanoparticles and the amount of curcumin present in the aqueous phase, employing the following formula: the E% was calculated by
In vitro drug release profile study
To study the drug release profile of the synthesized curcumin-loaded nanoparticles, 3 mg of drug-loaded nanoparticles were dispersed in 30 mL of phosphate-buffered solution (pH 7.4) and acetate buffer (pH 8, the pH value for survey of pH-dependent and pH-sensitive drug release kinetics). Samples were incubated at diverse temperatures from 37°C to 40°C. At designated time intervals, a 3 mL sample was removed and an equal volume was reconstituted by adding 3 mL of fresh phosphate-buffered solution and acetate buffer to every sample. After the experiment, the samples were evaluated using ultraviolet spectrofluorometry to determine the amount of curcumin released (λex 420 nm for curcumin measurement) (CitationAlizadeh et al. 2014, CitationPourhassan-Moghaddam et al. 2014, CitationAnganeh et al. 2014, CitationDavoudi et al. 2014, CitationAkbarzadeh et al. 2012b, CitationValizadeh et al. 2012, CitationAkbarzadeh et al. 2013b, CitationPourhassan-Moghaddam et al. 2013).
In vitro cytotoxicity and cell culture study
Cell culture
An MCF-7 human breast cancer cell line (Pasteur institute, Iran) was cultured in an RPMI1640 medium (Gibco, Invitrogen, Carlsbad, CA) supplemented with 10% heat-inactivated fetal bovine serum (FBS) (Gibco, Invitrogen), 2 g/ml sodium bicarbonate, 80 mg/mL penicillin G (Serva, Germany), 50 mg/ml streptomycin (Merck, Germany), and incubated at 37°C with humidified air containing 5% CO2.
Cell viability and MTT-based cytotoxicity assay
After culturing a sufficient amount of cells, the cytotoxic effects of pure and PLGA-PEG-loaded curcumin were studied using 24-h, 48-h, and 72-h MTT assays. Briefly, 10000 cells/well were cultivated in a 96-well plate (Costar from Corning, NY). After 24 h of incubation at 37°C in a humidified atmosphere including 5% CO2, the cells were treated with serial concentrations of pure and PLGA-PEG-loaded curcumin (0.5–70 μM) for 24, 48, and 72 h in quadruplicate, as cells treated with 0 mg/mL extract and 200 μL culture medium containing 10% dimethyl sulfoxide served as a control. After incubation, the medium in all wells of the plate was replaced with fresh medium and the cells were held for 24 h in an incubator. The medium in all the wells was then eliminated carefully, and 50 μL of 2 mg/mL MTT (Sigma Co., Germany) dissolved in phosphate- buffered solution was added to each well, and the plate was covered with aluminum foil and incubated for 4 h. Next, 200 μL of pure dimethyl sulfoxide was added to the wells after eliminating the contents of the wells. Next, 25 μL of Sorensen's glycine buffer was added and the absorbance of each well was instantly read at 570 nm using an EL × 800 microplate absorbance reader (Bio Tek Instruments, Winooski, VT) with a reference wavelength of 630 nm.
Statistical analysis
Statistical analysis was performed using SPSS 16 and the t-test to measure statistical differences among groups. Data with P < 0.05 were considered to be statistically significant.
Results
Measurement and characterization of nanoparticles
The structure of the copolymer is consistent through the FTIR spectrum. Using FTIR spectroscopy, the structure of PLGA-PEG copolymer was monitored.1H NMR spectra that were recorded at RT with a Brucker DRX 300 spectrometer operating at 400 MHz endorsed the basic chemical structure of the PLGA-PEG co-polymer. Tetramethylsilane (TMS) was applied as an internal reference for measuring chemical shift (δ) in ppm. In order to investigate the physicochemical characterization of nanoparticles, the nanoparticles were observed using SEM images.
FTIR spectroscopy
The FTIR spectrum is consistent with the structure of the supposed copolymer. FTIR spectroscopy was applied to demonstrate the structure of PLGA-PEG copolymer nanoparticles () and PLGA-PEG-loaded curcumin ().
1H NMR spectrum of the PEG-PLGA copolymer
The fundamental chemical structure of the PEG-PLGA copolymer is confirmed by 1H NMR spectra that were recorded in real-time with a Brucker DRX 300 spectrometer operating at 400 MHz. Chemical shift (δ) was calculated in ppm using tetramethylsilane as an internal reference. Because of the methylene groups of the PEG, there was a large peak at 3.65 ppm. Methyl groups of the D-lactic acid and L-lactic acid repeat units provide overlapping doublets at 1.55 ppm. There were peaks observed at 5.2 and 4.8 ppm, attributed to the lactic acid CH and the glycolic acid CH respectively ().
SEM Analysis
In order to investigate the physicochemical characterization of nanoparticles, the nanoparticles were observed through SEM images. The micrographs of the PLGA-PEG copolymers and PLGA-PEG-loaded curcumin are shown in and , respectively. From the photograph, the aggregation of nanoparticles can be clearly observed, which, due to size of PLGA-PEG, was 55 ± 6 nm. After encapsulation of curcumin in the PLGA-PEG nanoparticles, the size of the particles changed to 70–300 nm, and dispersion of the particles was considerably improved. These nanographs showed that the prepared PLGA-PEG nanoparticles were uniform and spherical in shape and of a standard size.
Drug loading and release study in vitro
Encapsulation efficiency was 84.5% and it was established that 1 mg of PLGA-PEG included 845 μg of curcumin. It was demonstrated that curcumin release is time-dependent and greater in a pH of 5.8 and a temperature of 40° C, which are characteristic of an environment with cancerous cells ().
Cell cytotoxicity (MTT assay)
In this study, to assess the cytotoxic effect (MTT assay) of free curcumin and curcumin-loaded PLGA-PEG, cells of the MCF-7 human breast cancer cell line were treated with diverse concentration (0.5–70μM) of free curcumin and curcumin-loaded PLGA-PEG for 24, 48 and 72h. IC50 after 24 h treatment with free curcumin and curcumin-loaded PLGA-PEG was 31.14 ± 1.24 and 17.86 ± 1.08 μM (p < 0.05) respectively (). PLGA-PEG and methanol 1% demonstrated an absorbance value equivalent to 98% and 96% of control respectively. It suggests that PLGA-PEG and methanol 1% have an extremely low effect on the cells. The IC50 after 48 h treatment with curcumin and curcumin-loaded PLGA-PEG were 21.32 ± 1.61 and 16.31 ± 1.31μM (p < 0.05) respectively (). After 72 h treatment with curcumin and curcumin-loaded PLGA-PEG nanoparticles, the IC50 values were 11.31 ± 1.47 and 6.21 ± 1.18 μM (p < 0.05) respectively () ().
Figure 8. MTT assay results for 24h. Cytotoxic effects of different concentrations of free curcumin and curcumin-loaded PLG-PEG in the MCF-7 human breast cancer cell line.
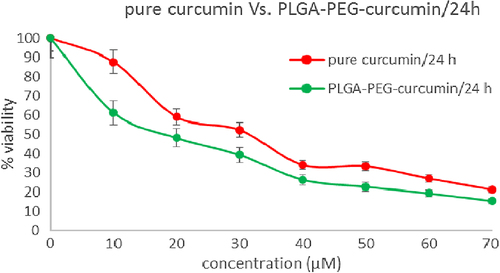
Figure 9. MTT assay results for 48h. Cytotoxic effects of different concentrations of free curcumin and curcumin-loaded PLG-PEG in the MCF-7 human breast cancer cell line.
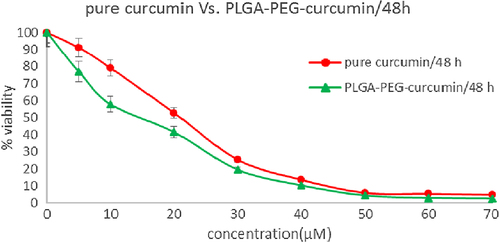
Figure 10. MTT assay results for 72h. Cytotoxic effects of different concentrations of free curcumin and curcumin-loaded PLG-PEG in the MCF-7 human breast cancer cell line.
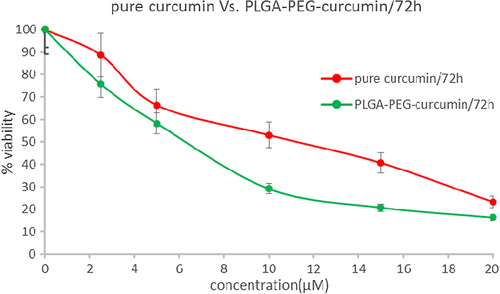
Table I. IC50 values of curcumin and curcumin-loaded PLGA-PEG at different times of incubation.
Discussion
Nanoparticles have become exceptionally attractive for their functions in the fields of biology and medicine in recent years. Nanoparticles also allocate a targeted direction to specific organs or cells or controlled drug delivery. The main purposes in designing nanoparticles as a delivery system are to manage surface properties, particle size and release of pharmacologically active agents, in order to achieve the site-specific action of the drug at the therapeutically optimal rate and dose regimen. Nanoparticle delivery systems are attractive as they target tumors and increase the tumor accumulation of anticancer agents in tumor cells more than in healthy tissues. For targeted delivery, persistence of nanoparticles is required in the systemic circulation of the body. In addition, a wide diversity of drugs can be delivered by using nanoparticles (CitationSadat Tabatabaei Mirakabad et al. 2014b).
Curcumin is a hydrophobic polyphenol extracted from turmeric, the rhizome of the herb Curcuma longa. Curcumin has been shown to exhibit therapeutic potential for a variety of different cancers (CitationAnand et al. 2008). Owing to its poor aqueous solubility, low bioavailability, and quick degradation and metabolism, the application of curcumin is limited in the treatment for animals and humans. To conquer these problems, different kinds of nano-preparations of this natural product have been developed (CitationLiu et al. 2013). In this paper, we used PLGA-PEG nanoparticles to boost curcumin solubility in an aqueous environment of cancer cells. By encapsulating drug molecules in a nanocarrier, the stability and solubility of drugs can be enhanced, providing a chance to re-evaluate the therapeutic potential of drugs due to poor pharmacokinetics. PLGA nanoparticles are generally used for the encapsulation of different cancer related drugs and their effective delivery in vivo. These loaded nanoparticles take care of poorly soluble and unstable payloads from the biological milieu and are minute enough for capillary penetrations, internalization and endosomal escape. Curcumin-loaded PLGA nanoparticles have emerged as a novel hope in curcumin delivery to tumor sites (CitationSadat Tabatabaei Mirakabad et al. 2014b). In this work, curcumin was encapsulated into PLGA-PEG by using the double emulsion method (w/o/w). FT-IR, 1H NMR and SEM analysis proved curcumin loading on PLGA-PEG. Curcumin-loaded PLGA-PEG nanoparticles were 70–300 nm in size and their encapsulation efficiency was 84.5%. It also demonstrated that curcumin has maximum amount of release in a cancer cell-like situation (40°C, pH = 5.8) (CitationKouhi et al. 2014, CitationSadat Tabatabaei Mirakabad et al. 2014a, CitationEatemadi et al. 2014, CitationAbbasi et al. 2014b, CitationAbbasi et al. 2014a, CitationAkbarzadeh et al. 2012a).
Mukerjee and Vishwanatha devised curcumin-loaded PLGA particles, and recommended that a nanoparticle-based formulation of curcumin has high probability as adjuvant therapy in prostate tumors (CitationDinarvand et al. 2011). In earlier reports, a number of polymeric nano-carriers were applied for curcumin drug delivery. CitationShaikh et al. (2009) formulated curcumin-loaded PLGA nanoparticles with 264 nm size and 76.9% encapsulation efficiency. These nanoparticles raised oral bioavailability 9-fold. Anand et al. employed PLGA-PEG nanoparticles for curcumin delivery. These nanoparticles (80.9 nm) have 97.5% encapsulation efficiency. They found that curcumin encapsulation into PLGA-PEG improves cellular uptake, enhances performance in vitro and improves bioavailability in vivo (CitationAnand et al. 2010). It was found in a recent study that PLGA nanoparticles enclosing curcumin stop the cell cycle at the G2/M in the MCF-7 breast cancer cell line (CitationVerderio et al. 2013). Furthermore, it was demonstrated that curcumin-loaded PLGA nanoparticles cause cellular uptake of this compound by the breast cancer cell line (MDA-MB-231) and the ovarian cancer cell line (A2780CP) to be augmented by 6 and 2-fold, respectively (CitationYallapu et al. 2010).
In this study, curcumin was encapsulated into PLGA-PEG for curcumin delivery to the MCF-7 human breast cancer cell line. Our study demonstrated that encapsulation of curcumin in PLGA-PEG improves curcumin cell cytotoxicity, releases in time and reduces IC50, which may be owing to enhanced accumulation of curcumin in an environment of cancer cells. This work is the first application of curcumin-loaded PLGA-PEG nanoparticles in the MCF-7 human breast cancer cell line treatment. Consequently, our results suggest that PLGA-PEG containing curcumin can be used for developing novel and effectual drug delivery systems for breast cancer treatment.
Conclusion
In conclusion, the present study revealed that curcumin-loaded PLGA-PEG nanoparticles have an inhibitory effect on the MCF-7 human breast cancer cell line to a greater degree than pure curcumin. This inhibition is dose and time dependent, as an analysis of our data demonstrates that PLGA-PEG particles release curcumin dose and time dependently. To discriminate between normal and cancerous cells, it is suggested that modifications be made to the surface of the PLGA-PEG nanoparticles with cell-surface receptors that over express via cancer cells. In addition, if in vivo studies confirm the efficacy of curcumin-loaded PLGA-PEG, it could be considered in a clinical experiment for patients with breast cancer in the future. Nevertheless, the future remains exciting and wide open, and more advances are needed to turn the concept of curcumin-loaded PLGA-PEG nanoparticle technology into a realistic practical function as the next generation of drug-delivery systems to overcome breast cancer.
Acknowledgements
The authors thank the Department of Medical Biotechnology, Faculty of Advanced Medical Sciences of Tabriz University of Medical Sciences, for all the support provided. This is a report of a database from a thesis entitled “The comparison between the effects of pure curcumin and curcumin-loaded PLGA-PEG nanoparticles on telomerase and PinX1 gene expression in breast cancer cell line”, registered in Tabriz University of Medical Sciences (No. 92/2-3/2).
Declaration of interest
The authors report no declarations of interest. The authors alone are responsible for the content and writing of the paper.
References
- Abbasi E, Aval SF, Akbarzadeh A, Milani M, Nasrabadi HT, Joo SW, Hanifehpour Y, Nejati-Koshki K, et al. 2014a. Dendrimers: synthesis, applications and properties. Nanoscale Res Lett. 9:247.
- Abbasi E, Milani M, Aval SF, Kouhi M, Akbarzadeh A, Nasrabadi HT, et al. 2014b. Silver nanoparticles: synthesis, properties, bio- applications and limitations. Crit Rev Microbiol. 1–8.
- Ahmadi A, Shirazi H, Pourbagher N, Akbarzadeh A, Omidfar K. 2014. An electrochemical immunosensor for digoxin using core-shell gold coated magnetic nanoparticles as labels. Mol Biol Rep. 41: 1659–1668.
- Akbarzadeh A, Mikaeili H, Zarghami N, Mohammad R, Barkhordari A, Davaran S. 2012a. Preparation and in-vitro evaluation of doxorubicin-loaded Fe3O4 magnetic nanoparticles modified with biocompatible copolymers. Int J Nanomedicine. 7:511–526.
- Akbarzadeh A, Nejati-Koshki K, Soghrati MM, Alimohammadi S, Ghamari MF, Davaran S. 2013a. In vitro studies of NIPAAM-MAA-VP copolymer-coated magnetic nanoparticles for controlled anticancer drug release. J Encapsulation Adsorpt Sci. 3:108–115.
- Akbarzadeh A, Rezaei A, Nejati-Koshki K, Alimohammadi S, Davaran S. 2014. Synthesis and physicochemical characterization of biodegradable star-shaped poly lactide-co-glycolide–β-cyclodextrin copolymer nanoparticles containing albumin. Adv Nanoparticles. 3:14–22.
- Akbarzadeh A, Rezaei-Sadabady R, Davaran S, Joo SW, Zarghami N, Hanifehpour Y, et al. 2013b. Liposome: classification, preparation, and applications. Nanoscale Res Lett. 8:102.
- Akbarzadeh A, Samiei M, Davaran S. 2012b. Magnetic nanoparticles: preparation, physical properties and applications in biomedicine. Nanoscale Res Lett. 7:144–157.
- Akbarzadeh A, Zarghami N, Mikaeili H, Asgari D, Goganian AM, Khiabani HK, Davaran S. 2012d. Synthesis, characterization and in vitro evaluation of novel polymer-coated magnetic nanoparticles for controlled delivery of doxorubicin. Int J Nanotechnol Sci Environ. 5:13–25.
- Akbarzadeh A, Samiei M, Joo SW, Anzaby M, Hanifehpour Y, Nasrabadi HT, Davaran S. 2012c. Synthesis, characterization and in vitro studies of doxorubicin-loaded magnetic nanoparticles grafted to smart copolymers on A549 lung cancer cell line. J Nanobiotechnol. 10:46.
- Alimirzalu S, Akbarzadeh A, Abbasian M, Alimohammadi S, Davaran S, Hanifehpour Y, et al. 2014. Synthesis and study of physicochemical characteristics of Fe3O4 magnetic nanocomposites based on poly(Nisopropylacrylamide)for anti-cancer drugs delivery. Asian Pac J Cancer Prev. 15:49–54.
- Alizadeh E, Zarghami N, Baghaban Eslaminejad MR, Akbarzadeh A, Jahangir S, Barzegar A, et al. 2014. The effect of dimethyl sulfoxide on hepatogenic differentiation of mesenchymal stem cells. Int J Pediatr. (2):57–58.
- Anand P, Nair HB, Sung B, Kunnumakkara AB, Yadav VR, Tekmal RR, Aggarwal BB. 2010. Design of curcumin-loaded PLGA nanoparticles formulation with enhanced cellular uptake, and increased bioactivity in vitro and superior bioavailability in vivo. Biochem Pharmacol. 79:330–338.
- Anand P, Sundaram C, Jhurani S, Kunnumakkara AB, Aggarwal BB. 2008. Curcumin and cancer: an “old-age” disease with an “age-old” solution. Cancer lett. 267:133–164.
- Anganeh MT, Sadat Tabatabaei Mirakabad F, Izadi M, Zeighamian V, Badrzadeh F, Salehi R, et al. 2014. The comparison between effects of free curcumin and curcumin loaded PLGA-PEG on telomerase and TRF1 expressions in calu-6 lung cancer cell line. Int J Biosci. 4:134–145.
- Danhier F, Ansorena E, Silva JM, Coco R, Le Breton A, Préat V. 2012. PLGA-based nanoparticles: an overview of biomedical applications. J Control Release. 161:505–522.
- Davoudi Z, Akbarzadeh A, Rahmatiyamchi M, Movassaghpour AA, Alipour M, Nejati-Koshki K, et al. 2014. Molecular target therapy of Akt and NF-kB signaling pathways and multidrug resistanceby specific cell penetrating inhibitor peptides in HL-60 cell line. Asian Pac J Cancer Prev. 9:4353–4358.
- Dinarvand R, Sepehri N, Manoochehri S, Rouhani H, Atyabi F. 2011. Polylactide-co-glycolide nanoparticles for controlled delivery of anticancer agents. Int J Nanomedicine. 6:877–895.
- Eatemadi A, Daraee H, Zarghami N, Melat Yar H, Akbarzadeh A, Hanifehpour Y. 2014. Nanofiber: synthesis and biomedical applications. Artif Cells Nanomed Biotechnol. 1–11.
- Ebrahimnezhad Z, Zarghami N, Keyhani M, Amirsaadat S, Akbarzadeh A, Rahmati M. 2013. Inhibition of hTERT gene expression by silibinin-loaded PLGA-PEG-Fe3O4 in T47D breast cancer cell line. BioImpacts. 3:67–74.
- Ghasemali S, Nejati-Koshki K, Tafsiri E, Rahmati-Yamchi M, Akbarzadeh A, Alizadeh E, et al. 2013. Study of inhibitory effect of β-cyclodextrin-helenalin complex on HTERT gene expression in T47D breast cancer cell line by real time quantitative PCR (q-PCR). Asian Pac J Cancer Prev. 14:6949–6953.
- Hosseininasab S, Pashaei-Asl R, Khandaghi AA, Nasrabadi HT, Nejati-Koshki K, Akbarzadeh A, et al. 2014. Synthesis, characterization, and In vitro studies of PLGA-PEG nanoparticles for oral Insulin delivery. Chem Biol Drug Design. 84:307–315.
- Jemal A, Bray F, Center MM, Ferlay J, Ward E, Forman D. 2011. Global cancer statistics. CA Cancer J Clin. 61:69–90.
- Kouhi M, Vahedi A, Akbarzadeh A, Hanifehpour Y, Joo SW. 2014. Investigation of quadratic electro-optic effects and electro absorption process in GaN/AlGaN spherical auantum dot. Nanoscale Res Lett. 9:1–6.
- Liu J, Chen S, Lv L, Song L, Guo S, Huang S. 2013. Recent progress in studying curcumin and its nano-preparations for cancer therapy. Curr Pharm Des. 19:1974–1993.
- Mahapatro A, Singh DK. 2011. Biodegradable nanoparticles are excellent vehicle for site directed in-vivo delivery of drugs and vaccines. J Nanobiotechnology. 9:55.
- McPherson K, Steel C, Dixon J. 2000. ABC of breast diseases: breast cancer—epidemiology, risk factors, and genetics. BMJ. 321:624.
- Mollazade M, Nejati-Koshki K, Akbarzadeh A, Zarghami N, Nasiri M, Jahanban-Esfahlan R, Alibakhshi A. 2013. PAMAM dendrimers arugment inhibitory effect of curcumin on cancer cell proliferation: possible inhibition of telomerase. Asian Pac J Cancer Prev. 14:6925–6928.
- Nejati-Koshki K, Akbarzadeh A, Pourhasan-Moghadam M, Joo SW. 2013. Inhibition of leptin and leptin receptor gene expression by silibinin- curcumin combination. APJCP. 14:6595.
- Pourhassan-Moghaddam M, Rahmati-Yamchi M, Akbarzadeh A, Daraee H, Nejati-Koshki K, Hanifehpour Y, Joo SW. 2013. Protein detection through different platforms of immuno-loop-mediated isothermal amplification. Nanoscale Res Lett. 8:485.
- Pourhassan-Moghaddam M, Zarghami N, Mohsenifar A, Rahmati-Yamchi M, Gholizadeh D, Akbarzadeh A, et al. 2014. Watercress-based gold nanoparticles: biosynthesis, mechanism of formation and study of their biocompatibility in vitro. Micro Nano Lett. 9:345–350.
- Rasul A, Parveen S, Ma T. 2012. Costunolide: A novel anti-cancer sesquiterpene lactone. Bangladesh J Pharmacol. 7: 6–13.
- Rezaei-Sadabady R, Zarghami N, Barzegar A, Eidi A, Akbarzadeh A, Rezaei-Tavirani M. 2013. Studies of the relationship between structure and antioxidant activity in interesting systems, including tyrosol, hydroxytyrosol derivatives indicated by quantum chemical calculations. Soft. 2:13–18.
- Sadat Tabatabaei Mirakabad F, Akbarzadeh A, Zarghami N, Zeighamian V, Rahimzadeh A, Alimohammadi S. 2014a. PLGA-cased nanoparticles as cancer drug delivery systems. Asian Pac J Cancer Prev. 15:517–535.
- Sadat Tabatabaei Mirakabad F, Nejati-Koshki K, Akbarzadeh A, Yamchi MR, Milani M, Zarghami N, et al. 2014b. PLGA-based nanoparticles as cancer drug delivery systems. Asian Pac J Cancer Prev. 15: 517–535.
- Sadeghi-Aliabadi H, Ahmadi A. 2000. Cytotoxicity and antitumor properties of a marine compound on cancer cells (HESA-A). Med J Islam Acad Sci. 13:55–61.
- Shaikh J, Ankola DD, Beniwal V, Singh D, Kumar MN. 2009. Nanoparticle encapsulation improves oral bioavailability of curcumin by at least 9-fold when compared to curcumin administered with piperine as absorption enhancer. Eur J Pharm Sci. 37:223–230.
- Singh R, Lillard JW Jr. 2009. Nanoparticle-based targeted drug delivery. Exp Mol Pathol. 86:215–223.
- Valizadeh A, Mikaeili H, Samiei M, Farkhani SM, Zarghami N, Kouhi M, et al. 2012. Quantum dots: synthesis, bioapplications, and toxicity. Nanoscale Res Lett.7:276.
- Verderio P, Bonetti P, Colombo M, Pandolfi L, Prosperi D. 2013. Intracellular drug release from curcumin-loaded PLGA nanoparticles induces G2/M block in breast cancer cells. Biomacromolecules. 14:672–682.
- Yallapu MM, Gupta BK, Jaggi M, Chauhan SC. 2010. Fabrication of curcumin encapsulated PLGA nanoparticles for improved therapeutic effects in metastatic cancer cells. J Colloid Interface Sci. 351:19–29.
- Zhou QM, Wang XF, Liu XJ, Zhang H, Lu YY, Su SB. 2011. Curcumin enhanced antiproliferative effect of mitomycin C in human breast cancer MCF-7 cells in vitro and in vivo. Acta Pharmacol Sin. 32: 1402–1410.