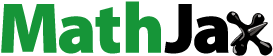
Abstract
In this work, the graft copolymer, poly(vinyl alcohol)-grafted polyacrylamide (PVA-g-PAAm), was synthesized and characterized by Fourier transform infrared spectroscopy, differential scanning calorimetry, and elemental analysis. Microspheres of PVA-g-PAAm/sodium alginate (NaAlg)/sodium carboxymethyl cellulose (NaCMC) were prepared by the emulsion-crosslinking method and used for the delivery of an Alzheimer's drug, donepezil hydrochloride (DP). The release of DP increased with the increase in drug/polymer ratio (d/p) and PVA-g-PAAm/NaAlg/NaCMC ratio, while it decreased with the increase in the extent of crosslinking. The optimum DP release was obtained as 92.9% for a PVA-g-PAAm/NaAlg/NaCMC ratio of 1/2/1, d/p ratio of 1/8, and FeCl3 concentration of 7% (w/v).
Introduction
For decades, polymeric structures have been used for pharmaceutical applications, particularly to provide controlled release of drugs. Polymer–drug systems may also be useful in protecting the drug from biological decomposition prior to its release. The development of such devices begins with the use of non-biodegradable polymers which depend on the diffusion process, and subsequently progresses to the use of biodegradable polymers in which swelling and erosion take place (CitationArifin et al. 2006).
Natural polymers such as methyl cellulose, sodium alginate (NaAlg), and chitosan have been the preferred polymers due to their biodegradability and biocompatibility. On the other hand, there are also some synthetic polymers that exhibit biocompatibility under the physiological conditions used in controlled release studies (CitationRamesh Babu et al. 2008). Poly(vinyl alcohol) (PVA) has been extensively used in controlled release studies due to its semicrystalline and hydrophilic nature (CitationSullad et al. 2010), biocompatibility and perfect mechanical properties to obtain stable microspheres (CitationÁlvarez et al. 2012). However, PVA is a highly hydrophilic polymer and has weak stability in water; therefore, the hydrophilicity of PVA must be controlled for its use (CitationŞanlı et al. 2007). This problem can be overcome through grafting (CitationKumbar and Aminabhavi 2002, CitationŞanlı et al. 2007), blending (CitationBajpai and Sharma 2005), and crosslinking (CitationŞanlı et al. 2009) techniques. Among these, the grafting technique can be considered as a practical tool for the preparation of new NaAlg/sodium carboxymethyl cellulose (NaCMC) microspheres with PVA.
NaAlg is a natural polysaccharide obtained from marine brown algae and composed of β-D-mannuronic acid and α-L-guluronic acid residues in varying proportions. Soluble NaAlg can be crosslinked with divalent or polyvalent cations to form an insoluble Alg (CitationMandal et al. 2010, CitationChan et al. 2002). CMC, a water-soluble polysaccharide possessing both hydroxyl and carboxylate groups, is an inexpensive polysugar that exerts strong interactions with metal ions (CitationButun et al. 2011). Metal–polymer composite materials mainly have been utilized in the development of sustained release drug delivery systems (CitationAlkhatib et al. 2006). Alg and CMC seem to be most commonly used in the preparation of polymer–metal composites (CitationMandal et al., 2010, CitationChan et al. 2002, CitationAlkhatib et al. 2006, CitationKim et al. 2012, CitationTaha et al. 2008, CitationSungur 1999, CitationRastogi et al. 2007, CitationXiao et al. 2009, CitationZheng et al. 2004, CitationŞanlı et al. 2008, CitationBajpai and Sharma 2004, CitationSilva et al. 2006, CitationHosny and al-Helw 1998). CitationKim et al. (2012) developed Fe3+-crosslinked Alg/CMC beads and investigated the release profile of albumin from the beads under simulated gastrointestinal conditions (pH 1.2, 4.5, and 7.4). They found that the blend beads were more effective at controlling the release of protein therapeutics. CitationSungur (1999) investigated the controlled release of erythromycin from CMC beads crosslinked with ferric ions, and applied different techniques to obtain a suitable delivery system. Sungur found minimal release in the stomach (simulated by pH 2.0) and a high release in the intestinal systems (simulated by pH 7.5), by applying a combination of wet and dry coating, drying and secondary crosslinking steps. CitationXiao et al. (2009) prepared Fe–CMC/PVA double network microparticles crosslinked via ionic crosslinking and freezing–thawing technology, and examined the stability of a protein in acidic and neutral media. They have found that the particles were special pH-sensitive vehicles with zero release in acidic medium and exhibited pH-responsive release behavior.
Donepezil is a piperidine-based reversible acetylcholinesterase inhibitor (AChEI) and the most prescribed pharmacological agent for the treatment of Alzheimer's disease (AD) (CitationChoi et al. 2012). AD is a progressive, neurodegenerative disease characterized by memory loss, impaired visuospatial skills, poor judgment, language deterioration, and indifferent attitude, but preserved motor function (CitationLiu et al. 2005). Donepezil, galantamine, and rivastigmine are the three AChEIs most widely used to treat AD (CitationChristodoulou et al. 2006). However, the large fluctuations in plasma concentration levels after oral administration of these drugs have been associated with gastrointestinal adverse effects including diarrhea, nausea, and vomiting (CitationChoi et al. 2012). For this reason, it is important to control delivery of the drug to improve its bioavailability. One way to overcome gastrointestinal problems is to encapsulate the drug in polymeric microspheres. The DP release profiles from microparticles (CitationZhang et al. 2007), tablets (CitationYan et al. 2010), and smectite clays (CitationPark et al. 2008) have been reported by researchers. CitationZhang et al. (2007) prepared poly(D,L-lactide-co-glycolide) microparticles by an oil–water emulsion solvent evaporation technique and investigated the controlled release of donepezil from the microparticles. Donepezil-loaded microparticles released the donepezil within 28 days in water, but showed a slower release in phosphate buffer solution (pH 7.4). CitationYan et al. (2010) prepared non-bitter orally disintegrating tablets of DP for enhanced patient compliance, by preparing microspheres with different ratios of drug and Eudragit® EPO using a spray drying method, and reported that the EPO-based drug-loaded microspheres neither decreased the bioavailability nor delayed the release of DP.
In this study, we report the development of novel, biologically compatible and pH-sensitive crosslinked microspheres of NaAlg/NaCMC and poly(vinyl alcohol)-grafted polyacrylamide (PVA-g-PAAm) blends for oral administration. For this purpose, AAm was grafted onto PVA and then blended with NaAlg and NaCMC to prepare PVA-g-PAAm/NaAlg/NaCMC microspheres which were crosslinked using different crosslinking agents. When exposed to the highly acidic (pH 1.2) environment of the stomach, the NaAlg microspheres may transform to the insoluble alginic acid form causing a reduction in their degree of crosslinking, and then degrade after arriving in the colon. PVA-g-AAm was used to increase the strength of the NaAlg/NaCMC microspheres so that after passing through the stomach, they can remain for a longer time in the intestinal pH conditions. The particle size, entrapment efficiency, microsphere yield, equilibrium swelling degree (ESD) of the microspheres, and DP release rates were studied at pH of 1.2, 6.8, and 7.4. The effects of the type of crosslinker, PVA-g-PAAm/NaAlg/NaCMC (w/w/w) ratio, crosslinker concentration, time of exposure to the crosslinker, and drug/polymer (d/p) ratio on the release of DP were investigated and discussed.
Experimental
Materials
NaAlg (medium viscosity) and NaCMC (medium viscosity) were purchased from Sigma Chemical Co. (USA). PVA and AAm were supplied by Merck (Darmstadt, Germany). The molecular weight and degree of saponification of PVA were 72,000 and greater than 98%, respectively. Light liquid paraffin was supplied by Aklar Chemistry Co. (Turkey). Glutaraldehyde (25% w/w), dimethyl sulfoxide (DMSO), FeCl3, CaCl2, AlCl3, ZnCl2, HCl, Span 85 (a commercial surfactant), n-hexane, acetone, and NaH2PO4 were all purchased from Merck (Darmstadt, Germany) and used as received. A gift sample of DP was provided by Sanovel Co. (Turkey).
Synthesis of the graft copolymer of PVA with AAm
The graft copolymer of PVA with AAm was synthesized at 40°C. The aqueous solution of PVA was degassed under a slow stream of nitrogen gas for 15 min. After that, AAm solution was added to this PVA solution and mixed for 15 min under a slow stream of nitrogen gas. At the end of this period, ceric ammonium nitrate was added slowly to the reaction mixture at the required concentration. A continuous supply of nitrogen was maintained throughout the reaction period. The grafting reaction was carried out for 3 h at a temperature of 40°C. At the end of 3 h, the reaction solution was added into an excess amount of acetone to precipitate the polymer, which was then filtered through suction and dried in a vacuum oven (Medcenter, Einrichtungen GmbH, Germany) at 60°C. The polymer was dissolved in DMSO and filtered to remove the homopolymer of AAm. The dissolved graft copolymer was reprecipitated in an excess amount of acetone and vacuum-dried at 40°C for 48 h.
The percentage of AAm in the graft copolymer was calculated from the elemental analysis (LECO CHNS-932 (USA) C, H, N analyzer) of PVA-g-PAAm as 34.32% (w/w) using Eq. (1), where A% is the mass% of AAM in the copolymer, Mm is the molar mass of the monomer, Me is the atomic weight of the element for which the elemental analysis was performed, and a is the mass % of an element (N%) in the copolymer other than carbon and hydrogen.
On the other hand, copolymerization efficiency was calculated according to the following formula (Eq. 2), where N and N0 are the nitrogen contents (%) found from the elemental analysis of the graft copolymer and the theoretical nitrogen content (%) of the graft copolymer, respectively.
Viscometric measurements
The viscosity measurements of PVA and PVA-g-PAAm solutions were determined using an Ubbelohde viscometer in water thermostated at 25°C. The intrinsic viscosity was determined by extrapolating the linear portion of the reduced viscosity versus concentration plot. The viscosity average molar mass, was calculated using the Mark–Houwink–Sakurada (MHS) relation:
The values of the MHS parameters, k and α, for PVA, were taken from the literature, assuming the constants did not change considerably with grafting (k = 1.4 × 10− 3 dL/g, α = 0.6] (CitationBrandrup and Immergut 1975).
Preparation of the PVA-g-PAAm/NaAlg/NaCMC microspheres
A water-in-oil emulsion technique was utilized for the preparation of the microspheres followed by crosslinking with crosslinker. NaAlg, NaCMC (2% (w/v) of each in water), PVA-g-PAAm (8% (w/v) in water), and DP in various d/p ratios were mixed and stirred for 12 h to form a homogenous solution. The aqueous phase was emulsified in light liquid paraffin in a ratio of 1:5 (v/v) containing 2% (w/v) Span 85 by magnetic stirring (Nuve, Turkey) at 400 rpm for 15–60 min. Then, crosslinker solution was added slowly to this emulsion and stirred to assure efficient crosslinking. Microspheres were collected by filtration, washed with n-hexane, and then dried completely in an oven (Memmert, Germany) at 40°C. The non-loaded microspheres were prepared in a similar way without DP. Different variables, such as crosslinker type, PVA-g-PAAm/NaAlg/NaCMC ratio, d/p ratio, concentration of crosslinking agent, and time required for crosslinking were considered in the optimization of the formulation of microspheres, which are listed in .
Swelling of the microspheres
ESDs of the crosslinked empty microspheres were determined gravimetrically by measuring the extent of their swelling in water and in solutions of pH 1.2, 6.8, and 7.4 at 37°C. To ensure complete equilibration, the samples were allowed to swell for 24 h. The excess surface-adhering liquid drops were removed by blotting. The swollen microspheres were weighed in an electronic balance (Sartorius, Korea), and then dried in an oven at 40°C until there was no change in the dried mass of the samples. The percent ESD was calculated as follows:
where Ms and Md are the masses of the swollen and dry microspheres, respectively.
Determination of DP content of the microspheres
A known mass of microspheres was crushed in an agate mortar and the polymeric powder was refluxed with 250 ml of distilled water for 4 h to ensure the complete extraction of DP from the microspheres. At the end of 4 h, precipitated polymer was filtered and the DP was analyzed using a TU-1880 Double Beam UV-VIS spectrophotometer at a wavelength of 270 nm, with distilled water as a blank. Actual DP loading was determined using a calibration curve. The entrapment efficiency (%) was then calculated as follows:
Fourier transform infrared spectroscopy
Fourier transform infrared (FTIR) spectra of DP, microspheres, and polymer samples were recorded within the wavelength of 400–4000 cm− 1 using KBr disks and a Perkin-Elmer BX-II (Germany) spectrometer.
Differential scanning calorimetry
Thermal analysis was performed using a differential scanning calorimeter (DSC; Shimadzu, Japan). Sample weights ranged from 5 to 8 mg. They were heated from 30°C to 300°C at a heating rate of 10°C/min.
Scanning electron microscopy studies
Scanning electron microscopy (SEM) photographs were taken using a LEO 1430 VP (Germany) scanning electron microscope to examine the morphology and surface structure of the microspheres at the required magnification at room temperature. The microspheres were deposited on a brass holder and sputtered with a thin coating of gold under vacuum.
X-ray diffractometry
X-ray diffractometry (XRD) patterns of donepezil hydrochloride (DP), empty microsphere, and DP-loaded microspheres were recorded on an XRD-6000 model (Shimadzu) diffractometer equipped with Cu Kα radiation, at a generator voltage of 40 kV and a generator current of 40 mA. Dried microspheres were triturated to get fine powder before taking the scan. The samples were scanned using diffraction angle (2θ) ranging from 0o to 60o at the speed of 2 Co/min.
Optical microscopy
Particle size of the microspheres was measured by optical microscopy (Olympus CH20BIMF200, Japan).
In vitro drug release
In vitro drug release from the microspheres was studied in 250 ml of HCl (pH 1.2) and phosphate buffer solutions (pH 6.8 and 7.4). The microspheres were incubated in a shaking water bath (Medline BS-21, Korea) at a speed of 100 rpm at 37°C. At 2-h intervals, the pH of the DP release medium was changed to 1.2, 6.8, and 7.4, respectively. At specified time intervals, the DP content was determined using an ultraviolet spectrophotometer at 270 nm. All experiments were performed in triplicate to minimize the variational error. The average values were used for further data treatment and plotting. Equal volumes of fresh HCl or phosphate buffer solution were added to the release medium to maintain the constant volume.
Results and discussion
Characterization of the graft copolymer and the microspheres
Graft copolymerization of PVA with AAm was achieved by the ceric ion-induced solution polymerization technique. The elemental analysis results, AAm % of PVA-g-PAAm, grafting efficiency, and the results of viscosity measurements, along with the viscosity average molecular masses, are presented in . As it can be seen from the table, the grafting efficiency of AAm onto PVA was up to 99.8 with 34.32% of grafting. The increase in molecular mass and the intrinsic viscosity of PVA could be taken as the proof of the grafting. shows the scheme of the possible reaction between PVA and AAm.
Table I. Elemental analysis, AAm % of PVA-g-PAAm, grafting efficiency (%), intrinsic viscosity, and viscosity average molar mass of polymers.
FTIR spectra of PVA (A) and grafted PVA (B) are presented in . A broad band around 3334 cm− 1 could be attributed to the O–H stretching vibration of the hydroxyl group of PVA. Similar O–H stretching (3406 cm− 1) was seen in the grafted copolymer spectra, indicating that all the hydroxyl groups of PVA were not involved in the grafting. A sharp band at 1093 cm− 1 corresponds to C–O–C symmetrical stretching (in the acetyl group) present on the PVA backbone, because of the unhydrolyzed acetate groups of poly(vinyl acetate). The peak was due to the N–H stretching vibration of the primary amide overlapped with the O–H stretching vibrations. The band observed at 2941 cm− 1 represented the presence of C–H aliphatic stretching vibrations. The specific absorption bands at 1668 cm− 1 corresponding to the C = O group (amide band 1) and 1614 cm− 1 corresponding to the NH group (amide band 2) were found in the grafted copolymer spectra. Grafting was also confirmed by appearance of strong band at 1447 cm− 1 corresponding to C–N stretching (CitationŞanlı et al. 2007, CitationKumbar and Aminabhavi 2002, CitationTudorachi and Lipsa 2006).
Figure 2. (I) FTIR results of PVA (A), PVA-g-PAAm (B); (II) FTIR results of NaAlg (A), NaCMC (B), PVA (C), DP (D), empty microspheres (E), DP-loaded microspheres (F).
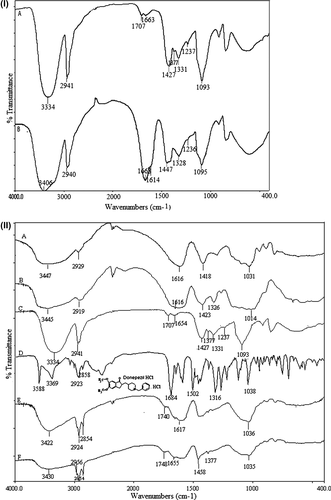
FTIR spectra of NaAlg, NaCMC, PVA, DP, and empty and drug-loaded crosslinked PVA-g-PAAm/NaAlg/NaCMC microspheres are shown in (A, B, C, D, E, and F). The spectrum of NaAlg ( (A)) showed peaks around 3447, 2929, 1616, 1418, and 1031 cm− 1 and the spectrum of NaCMC ( (B)) showed peaks around 3445, 2919, 1616, 1423, and 1014 cm− 1, indicating the stretching of O–H, aliphatic C–H, COO− (asymmetric), COO− (symmetric), and C–O–C, respectively (CitationBajpai et al. 2007, CitationRokhade et al. 2006, CitationPourjavadi et al. 2006). The spectrum of PVA was discussed in the previous paragraph. For DP, a sharp peak corresponding to the C = O stretching, C–N–C stretching, and C–H wagging bands were observed at 1684, 1502, and 1316 cm− 1, respectively. The stretching vibrations of sp3 C–H were also seen at 2923 and 2858 cm− 1 (CitationPark et al. 2008). DP-loaded microspheres showed no additional signals other than all the characteristic bands of the empty microsphere due to overlapping of DP-related signals. However, the bands in ∼3430 cm− 1 and ∼1608 cm− 1 being wider, indicated the chemical stability of the drug in the crosslinked microspheres.
DSC thermograms of PVA (A) and PVA-g-PAAm (B) are presented in . As it can be seen from , the Tg of the PVA used in this study was found to be 102°C, whereas the Tg of PVA-g-PAAm was found to be 148°C. This supports the modification of PVA by grafting with AAm that made the PVA thermally more stable, where similar results are available in literature (CitationŞanlı et al. 2007, CitationKumbar et al. 2003, CitationKumbar and Aminabhavi 2003). DSC thermograms of DP (A), DP-loaded microspheres (B), and empty microspheres (C) are presented in . DP showed a sharp endothermic peak at 230°C due to its melting. On the contrary, in case of DP-loaded microspheres, no characteristic peak was observed at 230°C, indicating the drug in the microspheres was molecularly dispersed.
Figure 3. (I) DSC thermograms of PVA (A), PVA-g-PAAm (B); (II) DSC thermograms of DP (A), DP-loaded microspheres (B), and empty microspheres (C).
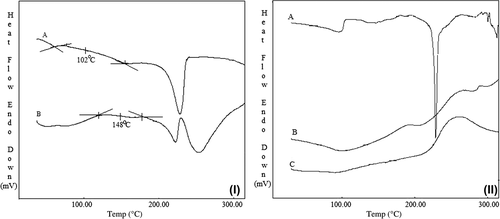
XRD diffractograms of DP (A), empty microspheres (B), and DP-loaded microspheres (C) are presented in . Here, DP revealed characteristic intense peaks between 2θ of 10o and 60o due to its crystalline nature. However, these peaks were not observed in the DP-loaded microspheres, indicating the dispersion of DP in polymer matrices at the molecular level.
SEM micrographs of empty and DP-loaded microspheres are shown in . As it can be seen from the figure, empty and DP-loaded microspheres were almost spherical in shape and empty microspheres showed a smooth surface, whereas DP-loaded microspheres showed roughness in the surface.
Particle size, entrapment efficiency, and yield value evaluation of the microspheres
The results of microsphere diameter, entrapment efficiency (%), and microsphere yield (%) measurements are displayed in . Particle size was measured by optical microscopy. The microspheres formed had particle sizes ranging from 42.92 μm to 123.53 μm in diameter. The size of the microspheres changed with the crosslinker type, PVA-g-PAAm/NaAlg/NaCMC (w/w/w) ratio, d/p ratio, crosslinker concentration, and the crosslinking time. Diameters of the microspheres prepared with FeCl3 and AlCl3 were larger than those prepared with CaCl2 and ZnCl2. Moreover, diameters of the microspheres prepared with GA were the largest among the all microspheres.
Table II. Preparation conditions, entrapment efficiency (%), microsphere yield (%), and microsphere size (μm) of the DP-loaded microspheres.
In the formulations, the diameter of the microspheres decreased with increasing PVA-g-PAAm content and crosslinking time. However, as the crosslinker concentration and d/p ratio were increased, the diameter of microspheres increased. These results are in accordance with the literature (CitationRastogi et al. 2007, CitationKumbar et al. 2003). CitationRastogi et al. (2007) prepared NaAlg microspheres for the delivery of isoniazid. They observed an increase in the particle size with the increase in the concentration of the crosslinker; however, a concentration of crosslinker above 10% caused the formation of irregular lumps due to extensive crosslinking of the guluronic acid units of NaAlg. CitationKumbar et al. (2003) prepared microspheres of PAAm-g-chitosan crosslinked with GA, and reported that as the percent of drug loading increased, the diameter of the microspheres increased, which might be due to the filling of the free volume of the microspheres.
The percentage of microsphere yield and entrapment efficiency might change depending on the preparation conditions of the microspheres. The results of entrapment efficiency (%) and microsphere yield (%) are presented in . As it can be seen from the table, the microspheres prepared with FeCl3 had a higher percentage of entrapment efficiency than the microspheres prepared with other crosslinkers. Entrapment efficiency might be related to the crosslinking mechanism of ions with NaAlg and NaCMC. The Ca2+ and Zn2+ cations are divalent and their bonding with Alg and CMC occurs in a planar two-dimensional manner which is usually described by the “egg-box” model (CitationTaha et al. 2008, CitationBajpai and Sharma 2004). In contrast, the trivalent cations, Fe3+ and Al3+, are expected to form a three-dimensional bonding structure (CitationKim et al. 2012, CitationHosny and al-Helw 1998). Although both ions are divalent, the percentage of entrapment efficiency of the microspheres prepared with Ca2+ ions was higher than that of the microspheres prepared with Zn2+. Similar results were stated by CitationTaha et al. (2008). Taha et al. prepared zinc-crosslinked Alg and calcium-crosslinked Alg beads for the release of chlorpheniramine maleate as a model drug and found that calcium crosslinking accessed higher drug loading levels compared to zinc crosslinking. They believed that this difference was related to the fact that calcium is a hard electrophile capable of forming quicker electrostatic attractive interactions with algin's carboxylates compared to the softer zinc ions.
A decrease in entrapment efficiency (%) and microsphere yield (%) was observed with an increasing amount of PVA-g-PAAm in the microspheres, which is due to the formation of a loose network that allows the leaching out of more drug particles during the production stage of the microspheres (CitationRokhade et al. 2006). In the microspheres, as the d/p ratio increased from 1/8 to 1/2, the entrapment efficiency and microsphere yield decreased from 98.74 to 36.64% and from 88.82 to 50.28%, respectively. This phenomenon may be explained as follows: when the d/p ratio increases, the polymer traps less DP and thus entrapment efficiency decreases (CitationŞanlı et al. 2008). With increasing time of exposure to the crosslinking agent, the entrapment efficiency (%) decreases and this decreasing trend might be attributed to the high aqueous solubility of DP resulting in loss of the drug during crosslinking, washing, and filtering processes (CitationRastogi et al. 2007, CitationŞanlı et al. 2008). As can also be seen from , an increase in the crosslinker concentration increased the entrapment efficiency. Similar results were stated by CitationRokhade et al. (2007). CitationRokhade et al. (2007) prepared interpenetrating polymer network microspheres of chitosan and methylcellulose for controlled release of theophylline and reported such a trend due to higher extent of crosslinking, resulting in the formation of a more rigid network, and this caused retention of more drug particles during the microsphere preparation.
In , microsphere yield (%) of the C6 formulation was found to be 108%. The increase from 7% to 10% (w/v) of concentration of FeCl3 solution caused the formation of irregular lumps due to extensive crosslinking which could prevent complete removal of the crosslinker from the microspheres.
Effect of crosslinker type on DP release
The effect of crosslinker type on the cumulative release of DP is shown in . The DP release rate from the microspheres crosslinked with Fe3+ ions was faster than that of the other microspheres. This observation might be related to the crosslinking mechanism of ions with NaAlg and NaCMC. As described in the previous section, calcium and zinc cations are divalent and their bonding to Alg and CMC is expected to occur in a planar two-dimensional manner (CitationTaha et al. 2008, CitationBajpai and Sharma 2004). In contrast, the trivalent cations, Fe3+ and Al3+, are expected to form a three-dimensional bonding structure (CitationKim et al. 2012, CitationHosny and al-Helw 1998). CitationAiedeh et al. (2007) prepared caffeine-containing chitosan diacetate (CDA) matrices ionotropically crosslinked with Al3+, Zn2+, and Ca2+. They have reported that the most probable explanation for this interesting behavior was based on the electrophilic softness/hardness of the three metallic cations. In another study, similar results were found by CitationTaha et al. (2008), who investigated the effects of sodium lauryl sulfate (anionic surfactant) on ionotropically crosslinked Alg beads with zinc and calcium ions. They have stated that calcium ions are hard electrophiles and therefore, interact with carboxylates via electrostatic attractions, which tend to be readily hydrated and thus broken under aqueous conditions. On the other hand, softer zinc ions tend to form covalent-like coordinate bonds with carboxylates, which are more resistant to hydration.
Figure 6. Effect of crosslinker type on the DP release. PVA-g-PAAm/NaAlg/NaCMC: 1/2/1, d/p: 1/4, exposure time to crosslinker: 30 min, concentration of crosslinker: 7%.
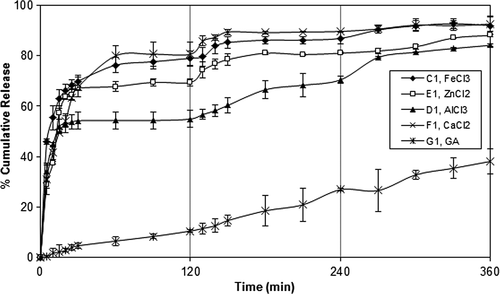
A slow release was observed from the microspheres crosslinked with GA. These results were also supported by swelling measurements shown in .
Table III. ESD of the empty microspheres.
FeCl3 was preferred as the crosslinking agent in the rest of the study due to the high entrapment efficiency.
Effect of PVA-g-PAAm/NaAlg/NaCMC ratio on DP release
In vitro release of DP from crosslinked PVA-g-PAAm/NaAlg/NaCMC microspheres was studied in gastric (2 h), input intestinal (2 h), and intestinal (2 h) pH conditions at 37°C. presents the data of cumulative release of DP from the microspheres with different PVA-g-PAAm/NaAlg/NaCMC ratios at pH of 1.2, 6.8, and 7.4, respectively. It can be seen from the figure that an increase in PVA- g-PAAm/NaAlg/NaCMC ratio from 1/2/1 to 3/2/1 caused an increase in the DP release from the microspheres. These results could be attributed to the increase in the hydrophilic character of the microspheres due to the presence of PVA-g-PAAm. The release results were also supported by the swelling measurements given in . As it can be seen from , the swelling degree of the PVA-g-PAAm/NaAlg/NaCMC microspheres increased with increasing amount of PVA-g-PAAm in the microspheres. However, as shows, the ESDs (%) of some microspheres at pH 1.2 (C1,0–C8,0) were higher than that of water. This could be attributed to the possible hydrolysis of amide groups of PVA-g-PAAm at pH 1.2, which leads to high ESD% of PVA-g-AAm/NaAlg/NaCMC microspheres at this pH () (CitationAsman et al. 2008).
Figure 7. Effect of PVA-g-PAAm/NaAlg/NaCMC ratio on the DP release. d/p: 1/4, exposure time to FeCl3: 30 min, concentration of FeCl3: 7%.
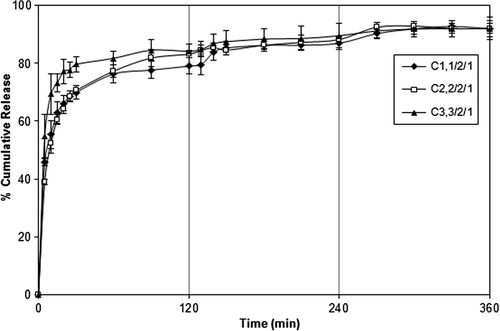
In the study on the controlled release of cefadroxil from pH-sensitive IPN microgels based on chitosan, by CitationKrishna Rao et al. (2006), AAm-g-PVA and hydrolyzed AAm-g-PVA were studied. They have reported an increase in the swelling of the blends due to incorporation of hydrophilic PVA segments along with PAAm chains into the IPN matrix. Similar results were also found in our previous study (CitationŞanlı et al. 2008, CitationŞanlı et al. 2009), where diltiazem hydrochloride (DT) containing PVP/NaAlg blend microspheres were prepared (CitationŞanlı et al. 2008) to achieve a controlled drug release profile suitable for oral administration. We have reported that DT release from the microspheres increased with an increase in the PVP/NaAlg ratio. Also, in the other study (CitationŞanlı et al. 2009), it was found that as the chitosan/PVA ratio in the microspheres decreased, drug release increased.
A PVA-g-PAAm/NaAlg/NaCMC ratio of 1/2/1 was preferred in the rest of the study due to more controlled DP release and high cumulative release at a pH of 7.4.
Effect of the concentration of FeCl3 and crosslinking time on the DP release
The release of DP from the microspheres depends on a number of physical and chemical parameters, including those related directly to the release medium, the release conditions (pH and temperature), preparation conditions, and those resulting from the changes in the characteristics of the microspheres. One of the most effective ways of changing the release rate of microspheres is to change the crosslinking density of the matrix, by employing varying concentrations of the crosslinking agent and time of exposure to crosslinking agent. The effect of concentration of FeCl3 on the release of DP was investigated by varying the concentration of FeCl3 solution from 3% to 10% (w/v). The results are given in , which clearly indicate that as the FeCl3 concentration increased (3% to 10%), the release of DP from the microspheres decreased. Maximum DP release was found to be 94.07% for the microspheres prepared with FeCl3 concentration of 3%. However, since the burst effect was high at this concentration, a FeCl3 concentration of 7% was preferred in the rest of the study.
Figure 8. (I) Effect of FeCl3 concentration on DP release. PVA-g-PAAm/NaAlg/NaCMC ratio: 1/2/1, d/p: 1/4, exposure time: 30 min; (II) Effect of exposure time to FeCl3 on DP release. PVA-g-PAAm/NaAlg/NaCMC ratio: 1/2/1, d/p: 1/4, concentration of FeCl3: 7%.
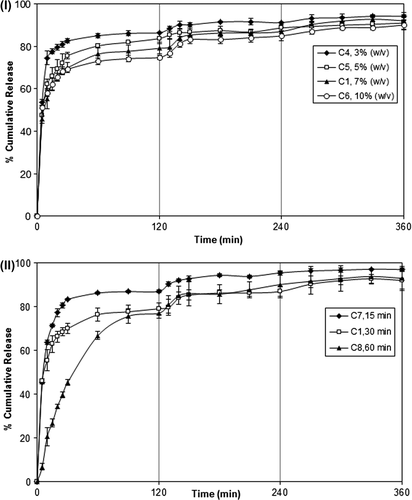
Another way of changing the crosslink density of the microspheres is to change the time of exposure to the crosslinking solution. For this purpose, exposure time to FeCl3 was changed from 15 min to 60 min and the results are presented in . indicates that increasing crosslinking time decreased the release rate, and the release of DP became more controlled.
An increase in the concentration of FeCl3 and exposure time resulted in an increase in the crosslink density of the microspheres, which gave rise to a tighter network structure. Consequently, free volume decreased, and permeation of water molecules and diffusion of drug molecules became more complicated. Similar observations were reported in some studies in the literature (CitationŞanlı et al. 2008, Citation2009, CitationKurkuri and Aminabhavi 2004, CitationAgnihotri and Aminabhavi 2006). CitationŞanlı et al. (2009) prepared chitosan/PVA blend microspheres for salicylic acid. They have reported that as the concentration of GA and crosslinking time increased, salicylic acid release decreased. CitationAgnihotri and Aminabhavi (2006) investigated release of the capecitabine from chitosan-poly(ethylene oxide-g-AAm) hydrogel microspheres and reported that drug release at higher amounts of GA was slower than that at lower amounts of GA due to high crosslinking density. DP release results were also supported by swelling measurements. As it can be seen from , as the exposure time to FeCl3 and concentration of FeCl3 increased, a decrease in ESD occurred because of the increase in crosslinking density. Similar results were reported in some of the studies in the literature (CitationŞanlı et al. 2007, Citation2008, CitationKumbar and Aminabhavi 2003, CitationAgnihotri and Aminabhavi 2004, CitationMundargi et al. 2008). CitationMundargi et al. (2008) studied controlled release of ampicillin from starch-based tableted microspheres crosslinked with epichlorohydrin (EPI). They have reported that as the amount of EPI increased from 4 to 8 ml, equilibrium water uptake decreased significantly from 586% to 380% due to the formation of a rigid network structure at a higher concentration of the crosslinking agent.
An exposure time of 30 min was preferred in the rest of the study.
Effect of d/p ratio on the DP release
Another parameter affecting DP release from microspheres could be the effect of d/p ratio. The effect of the d/p ratio on the release of DP is shown . As it can be seen from the figure, the cumulative DP release from the microspheres with d/p ratio of 1/2 was higher than that of microspheres with 1/4 and 1/8 d/p ratio. This is obvious because the release rate becomes quite high with the high amount of drug molecules in the matrix. CitationRamesh Babu et al. (2006) investigated the controlled release of ibuprofen (IB) from pH-sensitive interpenetrating network microgels of NaAlg/acrylic acid. They have reported that formulations containing the highest amount of drug (75%) displayed faster and higher release rates than those formulations containing a small amount of IB. A similar finding was reported for 5-fluorouracil-loaded semi-IPN microspheres from NaAlg and N-isopropylAAm by CitationMallikarjuna Reddy et al. (2008).
pH-responsive characteristics of microspheres
The role of pH in the degree of swelling of polymeric structures is of a great significance since it affects release characteristics of the polymeric structures. The degree of swelling in the ionically crosslinked carboxyl group containing anionic polymers decreases in an acidic medium due to the formation of hydrogen bonds between the COOH and OH groups. However, in phosphate solutions of pH 6.8 and 7.4, the carboxyl groups are partially ionized and electrostatic repulsion increases the degree of swelling (CitationKim et al. 2012). However, it is clear from –9 that PVA-g-PAAm/NaAlg/NaCMC microspheres have demonstrated a higher release of DP in the acidic pH of 1.2 than in the medium of pH 6.8 and 7.4. The drug–polymer interactions are an important factor governing drug release from the polymeric structures (CitationŞanlı et al. 2009). Interactions between –O– or –NR3 group of DP and the –OH, –NH2, or –COOH groups of PVA-g-PAAm/NaAlg/NaCMC blend microsphere may also affect release behavior.
Analysis of the kinetic results
Solvent sorption by a polymeric microsphere depends mechanistically on the diffusion of water molecules into the gel matrix and subsequent relaxation of macromolecular chains of the microsphere (CitationBajpai and Sharma 2005). Release results were analyzed by fitting the fraction release data Mt/M∞ using the empirical equations proposed by CitationPeppas (1985),
where k is a constant, characteristic of the drug–polymer system, and n is an empirical parameter characterizing the release mechanism; Mt is the amount of DP released at time t and M∞ is the drug released at equilibrium time. Fickian release is defined by an initial t1/2 time dependence of the fractional release for slabs, cylinders, and spheres. Analogously, Case-II transport is defined by an initial linear time dependence of the fractional release for all geometries (CitationRitger and Peppas 1987). A value of n = 0.5 indicates the Fickian transport (mechanism), while n = 1 is of Case II or non-Fickian transport (swelling controlled). The intermediary values ranging from 0.5 to 1.0 are attributed to the anomalous transport (CitationBabu et al. 2007). The least-squares estimations of the fractional release data along with the estimated correlation coefficient values, r, are presented in . According to these data, the n value ranged from 0.437 to 1.685, indicating that DP release from the microspheres has shown mainly anomalous transport (n = 0.5–1.0), in which drug release is governed by coupling of the drug diffusion and polymer relaxation processes.
The diffusion coefficient value, D, was calculated using the equations as follows (CitationRokhade et al. 2007):
where θ is slope of the linear portion of the plot of Mt/M∞ versus t1/2, r is the radius of the dry microspheres, and M∞ is the maximum drug release. The data reported in show the relationship between D and the crosslinker type, PVA-g-PAAm/NaAlg/NaCMC ratio, exposure time, concentration of FeCl3, and d/p ratio. It was noticed that the diffusion coefficient values showed an increase from 2.67 × 10− 12 to 30.39 × 10− 12 cm2/s with increasing PVA-g-PAAm/NaAlg/NaCMC ratio, which was also in agreement with the release results. The D value decreased from 2.67 × 10− 12 to 1.72 × 10− 12 cm2/s with increasing FeCl3 concentration. An increase in exposure time to FeCl3 decreased the diffusion coefficient, which was also in agreement with the release results. The D values also increased with increasing d/p ratio. Similar results were reported by many other workers (CitationRokhade et al. 2007, CitationRamesh Babu et al. 2006, CitationKulkarni et al. 1999). CitationRokhade et al. (2007) prepared IPN microspheres of chitosan and methylcellulose for controlled release of theophylline. They have observed that diffusion coefficient values were higher in the case of microspheres crosslinked with 5 ml of GA than those crosslinked with 10 ml of GA.
Conclusions
PVA-g-PAAm/NaAlg/NaCMC microspheres were successfully prepared by the emulsification crosslinking method and used for the delivery of DP. The microspheres formed were characterized using SEM, DSC, XRD, and FTIR techniques. DSC, XRD, and FTIR studies indicated that DP was molecularly dispersed in the microspheres. Microsphere size, entrapment efficiency, microsphere yield, and ESD of the microspheres were determined and it was found that these parameters were affected by the various processing parameters, such as PVA-g-PAAm/NaAlg/NaCMC ratio, type of crosslinker, crosslinker concentration, time of exposure to the crosslinker, and d/p ratio. The release of DP increased with the increase in d/p and PVA-g-PAAm/NaAlg/NaCMC ratio while it decreased with the increase in the extent of crosslinking. The optimum DP release was obtained as 92.9% for PVA-g-PAAm/NaAlg/NaCMC ratio of 1/2/1, d/p ratio of 1/8, and crosslinker concentration of 7% (w/v) at the end of 6 h. Diffusion constants and ESDs of all the formulations were found to be consistent with the release results. The n values calculated for the release of drug from microspheres indicated that DP release mainly displayed anomalous transport.
Declaration of interest
The authors report no declarations of interest. The authors alone are responsible for the content and writing of the article.
The authors are grateful to the Gazi University Scientific Research Foundation for their support and to Sanovel Company for the supply of the drug (Donepezil hydrochloride).
References
- Agnihotri SA, Aminabhavi TM. 2004. Controlled release of clozapine through chitosan microparticles prepared by a novel method. J Control Release. 96:245–259.
- Agnihotri SA, Aminabhavi TM. 2006. Novel interpenetrating network chitosan-poly(ethylene oxide-g-acrylamide) hydrogel microspheres for the controlled release of capecitabine. Int J Pharm. 324:103–115.
- Aiedeh KM, Taha MO, Al-Hiari Y, Bustanji Y, Alkhatib HS. 2007. Effect of ionic crosslinking on the drug release properties of chitosan diacetate matrices. J Pharm Sci. 96:38–43.
- Alkhatib HS, Taha MO, Aiedeh KM, Bustanji Y, Sweileh B. 2006. Synthesis and in vitro behavior of iron-crosslinked N-methyl and N-benzyl hydroxamated derivatives of alginic acid as controlled release carriers. Eur Polym J. 42:2464–2474.
- Álvarez AL, García AB, Callejas FG, Couso HG, Méndez JB. 2012. In vitro evaluation of the suppressive effect of chitosan/poly(vinyl alcohol) microspheres on attachment of C. parvum to enterocytic cells. Eur J Pharm Sci. 47:215–227.
- Arifin DY, Lee LY, Wang C-H. 2006. Mathematical modeling and simulation of drug release from microspheres: Implications to drug delivery systems. Adv Drug Deliv Rev. 58:1274–1325.
- Asman G, Şanlı O, Tuncel D. 2008. pH- and temperature-sensitive in vitro release of salicylic acid through poly(vinyl alcohol-g- acrylamide) membranes. J Appl Polym Sci. 107:3005–3012.
- Babu VR, Sairam M, Hosamani KM, Aminabhavi TM. 2007. Preparation of sodium alginate–methylcellulose blend microspheres for controlled release of nifedipine. Carbohydr Polym. 69:241–250.
- Bajpai AK, Sharma M. 2005. Preparation and characterization of binary grafted polymeric blends of polyvinyl alcohol and gelatin and evaluation of their water uptake potential. J Macromol Sci Part A Pure Appl Chem. 42:663–682.
- Bajpai J, Mishra S, Bajpai AK. 2007. Dynamics of controlled release of potassium nitrate from a highly swelling binary polymeric blend of alginate and carboxymethyl cellulose. J Appl Polym Sci. 106:961–972.
- Bajpai SK, Sharma S. 2004. Investigation of swelling/degradation behaviour of alginate beads crosslinked with Ca2+ and Ba2+ ions. React Func Polym. 59:129–140.
- Brandrup J, Immergut EH. 1975. Polymer Handbook, 2nd ed. New York: John Wiley and Sons.
- Butun S, Ince FG, Erdugan H, Sahiner N. 2011. One-step fabrication of biocompatible carboxymethyl cellulose polymeric particles for drug delivery systems. Carbohydr Polym. 86:636–643.
- Chan LW, Lee HY, Heng P. 2002. Production of alginate microspheres by internal gelation using an emulsification method. Int J Pharm. 242:259–262.
- Choi J, Choi M-K, Chong S, Chung S-J, Shim C-K, Kim D-D. 2012. Effect of fatty acids on the transdermal delivery of donepezil: In vitro and in vivo evaluation. Int J Pharm. 422:83–90.
- Christodoulou C, Melville P, Scherl WF, MacAllister WS, Elkins LE, Krupp LB. 2006. Effects of donepezil on memory and cognition in multiple sclerosis. J Neuro Sci. 245:127–136.
- Hosny EA, al-Helw A al-R. 1998. Effect of coating of aluminium carboxymethylcellulose beads on the release and bioavailability of diclofenac sodium. Pharm Acta Helv. 72:255–261.
- Kim MS, Park SJ, Gu BK, Kim C-H. 2012. Ioinically crosslinked alginate-carboxymethyl cellulose beads for the delivery of protein therapeutics. Appl Surf Sci. 262:28–30.
- Krishna Rao KSV, Naidu BVK, Subha MCS, Sairam M, Aminabhavi TM. 2006. Novel chitosan-based pH-sensitive interpenetrating network microgels for the controlled release of cefadroxil. Carbohydr Polym. 66:333–344.
- Kulkarni AR, Soppimath KS, Aminabhavi TM. 1999. Controlled release of diclofenac sodium from sodium alginate beads crosslinked with glutaraldehyde. Pharm Acta Helv. 74:29–36.
- Kumbar SG, Aminabhavi TM. 2002. Preparation and characterization of interpenetrating network beads of poly(viny alcohol)-grafted-Poly(acrylamide) with sodium alginate and their conrtrolled release characteristics for cypermethrin pesticide. J Appl Polym Sci. 84: 552–560.
- Kumbar SG, Soppimath KS, Aminabhavi TM. 2003. Synthesis and characterization of polyacrylamide-grafted chitosan hydrogel microspheres for the controlled release of indomethacin. J Appl Polym Sci. 87:1525–1536.
- Kumbar SG, Aminabhavi TM. 2003. Synthesis and characterization of modified chitosan microspheres: Effect of the grafting ratio on the controlled release of nifedipine through microspheres. J Appl Polym Sci. 89:2940–2949.
- Kurkuri MD, Aminabhavi TM. 2004. Poly(vinyl alcohol) and poly(acrylic acid) sequential interpenetrating network pH-sensitive microspheres for the delivery of diclofenac sodium to the intestine. J Control Release. 96:9–20.
- Liu WH, Song JL, Liu K, Chu DF, Li YX. 2005. Preparation and in vitro and in vivo release studies of Huperzine A loaded microspheres for the treatment of Alzheimer's disease. J Control Release. 107:417–427.
- Mallikarjuna Reddy K, Ramesh Babu V, Krishna Rao KSV, Subha MCS, Chowdoji Rao K, Sairam M, Aminabhavi TM. 2008. Temperature sensitive semi-IPN microspheres from sodium alginate and n-isopropylacrylamide for controlled release of 5-fluorouracil. J Appl Polym Sci. 107:2820–2829.
- Mandal S, Basu SK, Sa B. 2010. Ca2+ ion cross-linked interpenetrating network matrix tablets of polyacrylamide-grafted-sodium alginate and sodium alginate for sustained release of diltiazem hydrochloride. Carbohydr Polym. 82:867–873.
- Mundargi RC, Shelke NB, Rokhade AP, Patil SA, Aminabhavi TM. 2008. Formulation and in-vitro evaluation of novel starch-based tableted microspheres for controlled release of ampicillin. Carbohydr Polym. 71:42–53.
- Park JK, Choy YB, Oh J-M, Kim JY, Hwang S-J, Choy J-H. 2008. Controlled release of donepezil intercalated in smectite clays. Int J Pharm. 359:198–204.
- Peppas NA. 1985. Analysis of Fickian non Fickian drug release from polymers. Pharm Acta Helv. 60:110–111.
- Pourjavadi A, Barzegar SH, Mahdavinia GR. 2006. MBA-crosslinked Na-Alg/CMC as a smart full-polysaccharide superabsorbent hydrogels. Carbohydr Polym. 66:386–395.
- Ramesh Babu V, Hosamani KM, Aminabhavi TM. 2008. Preparation and in-vitro release of chlorothiazide novel pH-sensitive chitosan-N,N’-dimethylacrylamide semi-interpenetrating network microspheres. Carbohydr Polym. 71:208–217.
- Ramesh Babu V, Krishna Rao KSV, Sairam M, Naidu BVK, Hosamani KM, Aminabhavi TM. 2006. pH sensitive interpenetrating network microgels of sodium alginate-acrylic acid for the controlled release of ibuprofen. J Appl Polym Sci. 99:2671–2678.
- Rastogi R, Sultana Y, Aqil M, Ali A, Kumar S, Chuttani K, Mishra AK. 2007. Alginate microspheres of isoniazid for oral sustained drug delivery. Int J Pharm. 334:71–77.
- Ritger PL, Peppas NA. 1987. A simple equation for description of solute release. II. Fickian and anomalous release from swellable devices. J Control Release. 5:37–42.
- Rokhade AP, Agnihotri SA, Patil SA, Mallikarjuna NN, Kulkarni PV, Aminabhavi TM. 2006. Semi-interpenetrating polymer network microspheres of gelatin and sodium carboxymethyl cellulose for controlled release of ketorolac tromethamine. Carbohydr Polym. 65:243–252.
- Rokhade AP, Shelke NB, Patil SA, Aminabhavi TM. 2007. Novel interpenetrating polymer network microspheres of chitosan and methylcellulose for controlled release of theophylline. Carbohydr Polym. 69:678–687.
- Silva CM, Ribeiro AJ, Figueiredo IV, Gonçalves AR, Veiga F. 2006. Alginate microspheres prepared by internal gelation: Development and effect on insulin stability. Int J Pharm. 311:1–10.
- Sullad AG, Manjeshwar LS, Aminabhavi TM. 2010. Controlled release of theophylline from interpenetrating blend microspheres of poly(vinyl alcohol) and methyl cellulose. J Appl Polym Sci. 116:1226–1235.
- Sungur S. 1999. Investigations on drug release systems using CMC crosslinked with ferric ions. Art Cells Blood Subs Immob Biotech. 27:279–290.
- Şanlı O, Ay N, Işıklan N. 2007. Release characteristics of diclofenac sodium from poly(vinyl alcohol)/sodium alginate and poly(vinyl alcohol)-grafted-poly(acrylamide)/sodium alginate blend beads. Eur J Pharm Biopharm. 65:204–214.
- Şanlı O, Biçer E, Işıklan N. 2008. In vitro release study of diltiazem hydrochloride from poly(vinyl pyrrolidone)/sodium alginate blend microspheres. J Appl Polym Sci. 107:1973–1980.
- Şanlı O, Karaca İ, Işıklan N. 2009. Preparation, characterization, and salicylic acid release behavior of chitosan/poly(vinyl alcohol) blend microspheres. J Appl Polym Sci. 111:2731–2740.
- Taha MO, Nasser W, Ardakani A, AlKhatib HS. 2008. Sodium lauryl sulfate impedes drug release from zinc-crosslinked alginate beads: Switching from enteric coating release into biphasic profiles. Int J Pharm. 350:291–300.
- Tudorachi N, Lipsa R. 2006. Copolymers based on poly(vinyl alcohol) and acrylamide. J Optoelect Advanc Mater. 8:659–662.
- Xiao C, Li H, Gao Y. 2009. Preparation of fast pH-responsive ferric carboxymethyl cellulose/poly(vinyl alcohol) double-network microparticles. Polym Int. 58:112–115.
- Yan Y-D, Woo JS, Kang JH, Yong CS, Choi HG. 2010. Preparation and evaluation of taste-masked donepezil hydrochloride orally disintegrating tablets. Biol Pharm Bull. 33:1364–1370.
- Zhang P, Chen L, Gu W, Xu Z, Gao Y, Li Y. 2007. In vitro and in vivo evaluation of donepezil-sustained release microparticles for the treatment of Alzheimer's disease. Biomaterials. 28:1882–1888.
- Zheng C-H, Gao J-Q, Zhang YP, Liang W-Q. 2004. A protein delivery system: biodegradable alginate-chitosan-poly(lactic-co-glycolic acid) composite microspheres. Biochem Biophys Res Commun. 323:1321–1327.