Abstract
Over the last 50 years, compelling evidence has accumulated on the beneficial role of selenium in human health. In the present study, different proteins were evaluated as reducing agents for the eco-friendly synthesis of selenium nanoparticles from an aqueous solution of sodium selenite. This method is a simple, low cost green synthesis alternative to chemical synthesis. The high conversion of selenium ions to selenium nanoparticles (SeNPs) was achieved by a reaction mixture of 0.1 g bovine serum albumin and 0.1 g sodium selenite at a reaction temperature of 121°C for 20 min duration. The selenium nanoparticles were characterized by fourier transform infrared (FTIR), scanning electron microscopy (SEM) and energy-dispersive X-ray spectroscopy. The FTIR spectral bands were sharp with strong absorption peaks at 1649 and 1551 cm− 1. SEM analysis of the synthesized selenium nanoparticles clearly showed the spherical shape with an average size ranging from 500 to 600 nm. The toxicity of SeNPs was evaluated using zebrafish embryos as a model system. SeNPs induced malformations in zebrafish embryos in a concentration-dependent manner. Selenium nanoparticles at 15–25 μg/ml concentration caused pericardial edema, tail malformation and decrease in heart rate in zebrafish embryos. Treatments with lower concentrations did not alter the heart rate or display any heart abnormalities. This study underlines the importance of identifying optimal SeNP concentration that could have potential therapeutic applications.
Introduction
Selenium is an essential trace element identified in the early 1950s as vital for the survival of several organisms, including mammals; selenium is important for various aspects of human health, including cardiovascular health (CitationKlayman and Gunter 1973, CitationSuadicani et al. 1992). Due to the developments in nanotechnology, much effort has been made to produce selenium at the nanoscale level and to evaluate its potential use in various medicinal applications such as cancer therapy (CitationHuang et al. 2013). Selenium nanoparticles have been found to exhibit strong anti-oxidative (CitationLi et al. 2013), anti-leishmanial (CitationSoflaei et al. 2014) and anti-bacterial effects (CitationTran and Webster 2011).
Recent developments in nanotechnology mainly focus on the synthesis and development of metal nanoparticles. The methods of nanoparticle synthesis still face the challenge of optimizing the size and monodispersity (CitationMónica et al. 2013). The physical and chemical methods of nanoparticle synthesis are more popular. They are expensive processes involving the use of toxic chemicals and the possibilities of toxic chemicals absorbed on the surface of the nanoparticles is high (CitationSchrade et al. 2013). These short comings can be overcome by the use of eco-friendly synthesis of nanoparticles (CitationSriram et al. 2010). Recently, several reports are available on the use of bacteria, yeast, fungi and plant sources that all play an important role in biosynthesis of nanoparticles (CitationKalishwaralal et al. 2010, CitationSyed et al. 2013). Bacteria such as Zooglea ramigera (CitationSrivastava and Mukhopadhyay 2013), Bacillus selenitireducens (CitationSwitzer et al. 1998), Selenihalanaerobacter shriftii (CitationBlum et al. 2001), Bacillus cereus (CitationDhanjal and Cameotra 2010) and Pseudomonas alcaliphila (CitationZhang et al. 2011), can survive and grow at high selenium concentrations and they have applications in the adsorption of these metal ions. The toxicity of selenium ions is reduced or eliminated by changing the redox state of the selenium ions and in the process leading to the formation of well-defined nanoscale particles. However, synthesis of selenium nanoparticles by bacteria also has some drawbacks. The major problem encountered is the isolation and purification of the nanoparticle from the selenium resistant bacteria. Most of these techniques involve capital and energy intensive downstream processing steps including sonication and ultracentrifugation (CitationKalishwaralal et al. 2010, CitationKalimuthu et al. 2008). As an alternative to this, a low cost and eco-friendly method for the biosynthesis of selenium ions to selenium nanoparticles (SeNPs) is described here; the method involves one-pot synthesis of selenium nanoparticles using bovine serum albumin as the reducing and stabilizing agent.
Selenium-deficiency leads to endemic cardiomyopathy and Keshan disease in humans (CitationTiekink 2012). Both the diseases were first identified in parts of China where the soil is low in selenium content. An inverse association between the risk of heart disease and serum selenium concentration below 79 μg/l was also reported from a Danish study (CitationKardinaal et al. 1997). Cardiopulmonary bypass surgery, in patients with a low level of selenium in the blood, leads to organ failure (CitationStoppe et al. 2013). Treatment with SeNPs could be used as a therapeutic agent in cardio-related disorders; but pre-evaluation of toxicity of SeNPs is necessary before considering the therapeutic options. To address this issue, zebrafish (Danio rerio) embryos were selected as an experimental model for evaluating cardiotoxicity. To the best of our knowledge, there is no report available till date on the biocompatibility and chronotropic effects (heart rate) of selenium nanoparticles in zebrafish embryos.
Materials and methods
Biosynthesis of selenium nanoparticles
Three different proteins namely bovine serum albumin (BSA), lipase and protease purchased from HiMedia Laboratories, India were used. Aliquots (0.1 g) of each of these proteins were mixed with 50 ml volume of sodium selenite (0.1 g) (HiMedia, India). The solution was kept in an autoclave under conventional sterilization conditions (121°C, 15–20 psi for 20 min).
Purification of nanoparticles
The nanoparticles carrying protein suspension were centrifuged at 12,000 rpm (1725 × g) for 10 min and then the supernatant was discarded. Protein containing the nanoparticles was washed and resuspended in deionized water and again centrifugation was done at the same speed mentioned above. The washing step was repeated three times at room temperature.
Size determination with SEM
The size and shape of nanoparticles were measured by Scanning Electron Microscopic analysis using a Philips JSM 6390 model (USA) electron microscope. Essentially for analysis, one drop of sample suspension was taken for scanning electron microscopy (SEM). The selected areas within SEM sections were subjected to elemental composition analysis using an energy-dispersive X-ray spectroscopy (EDX) microanalysis system coupled to the scanning electron microscope.
FTIR spectral analysis
The synthesized selenium nanoparticles were further analyzed for their chemical structure using Fourier Transformer - Infrared Spectrophotometer (Shimadzu – 8400, Japan) by KBr pellet method as described by CitationNarmato (1997).
Treatment of zebrafish embryos with selenium nanoparticles
Briefly, the zebrafish embryos at the early blastula stage were transferred to a petri plate at 10 embryo/petri plate. The embryos were treated with different concentrations of selenium nanoparticles (5, 10, 15, 20, 25 μg/ml) for 24 h. The experiment was performed in triplicate and 10 embryos were used as a control. The development of the zebrafish embryos until hatching of the larvae was observed with an optical microscope (Primo Star Carl Zeiss, Germany).
Statistical methods
The data of results are reported as the mean ± standard deviation from at least three separate experiments.
Results and discussion
Bovine serum albumin-mediated synthesis of selenium nanoparticles
BSA is a most abundant plasma protein which consists of three homologous domains (domains I, II, and III) in their tertiary structure, and its cysteine residues form 17 disulfide bonds to produce a double-loop bridging pattern (CitationCarter and Ho 1994, CitationHe and Carter 1992). When the protein is exposed to heat at 121°C, the structure of BSA will facilitate the breaking of the disulfide bonds and further promote the unfolding of the protein, exposing more -SH groups (). These -SH and hydroxyl groups could be used for the reduction of Se (IV) to Se (0) by BSA, resulting in change of color of the reactant solution from clear white to clear red (data not shown). The color formation in the reaction mixture is due to the excitations of surface plasmon resonance of the selenium nanoparticles formed in the reaction mixture (CitationCharles et al. 2011). However, it is interesting to note that when the same reaction was carried out in the presence of other proteins tested like lipase and protease, they could not produce any color change in the solution. This may be due to the lack of free and exposed -SH groups in lipase and protease enzymes which could not have contributed for the production of the selenium nanoparticles (CitationAu et al. 2010).
Analysis of selenium nanoparticles using SEM
Particle size analysis of selenium nanoparticles was done using SEM and the result is presented in . The photomicrograph of SeNPs showed a wide distribution of size in the sample; the observed SeNPs were spherical in shape (). The SEM photomicrograph of selenium nanoparticles showed a size of 500–600 nm which is very narrow in size distribution (). This observation is in contrast to the previous reports; selenite-resistant bacteria such as Sulfurospirillum barnesii, Bacillus selenitireducens, and Selenihalanaerobacter shriftii produce selenium nanoparticles as unstructured, amorphous aggregates that range in diameter from 200 to 800 nm as analyzed by SEM (CitationOremland et al. 2004). However, CitationCharles et al. (2011) demonstrated the synthesis of Se nanospheres (approximately 300 nm) using both E. coli expressing recombinant Se factor A and purified recombinant protein. The EDX profile shows a strong selenium signal along with weak sulfur group peaks. The result indicated that 92.76% (wt.) of the sample had the presence of selenium nano particles. The detection of the presence of sulfur 7.24% (wt.) in the EDX spectra, confirms the presence of sulfur containing protein/peptide molecules bound to the surface of the nanoparticles (CitationSyed et al. 2013) ().
Fourier transformer – infrared spectrophotometer studies
FTIR analysis was performed to characterize the surface chemistry of selenium nanoparticles produced by BSA and analysis of FTIR indicated protein mediated synthesis of selenium nanoparticles; the strong absorption bands at 1649 and at 1551 cm− 1 are characteristic of amide I and C-H vibrations of CH2 groups of protein moiety respectively, with albumin as the stabilizing and capping agent surrounding the selenium nanoparticles ().
Effect of selenium nanoparticles on zebrafish embryo
Zebrafish embryos have been used as an in vivo assay model and could potentially be used for preclinical drug development for human diseases. Zebrafish and humans share 85% homology at the genome level (CitationBarbazuk et al. 2000). In order to evaluate the toxicity of SeNPs, zebrafish embryos were exposed to 500 nm SeNPs at different concentrations (5–25 μg/ml). Zebra fish eggs contain a layer of chorionic membrane as an outside barrier with numerous distributed pore canals (0.5–0.7 μm in diameter). As illustrated in , large sized selenium nanoparticles were adsorbed on the outer surface of the embryonic chorion in a concentration dependent manner. This is in corroboration with the observation of CitationBai et al. (2010), who observed direct penetration of SeNPs into the zebrafish embryo. The effect of SeNPs on the viability of zebrafish embryo was also observed (). Though there was no mortality noted in lower concentrations (5–10 μg/mL), exposing these embryos to higher SeNP concentrations (20 and 25 μg/mL) resulted in a significant percentage of mortality compared to that of control group at 96 h post fertilization (hpf). Zebrafish embryos exposed to 15–25 μg/ml of SeNPs induced various developmental abnormalities which were observed and documented at 96 hpf. The lower concentrations (5–10 μg/mL) of SeNP did not show any significant malformation (such as pericardial edema and tail malformation) throughout the tested time points () whereas both pericardial edema and tail malformation were observed at 20–25 μg/ml SeNPs-treated embryos (). These observations corroborate with that of CitationDuan et al. (2013), who also reported malformations of pericardial edema and tail malformation in embryos treated with silica nanoparticles.
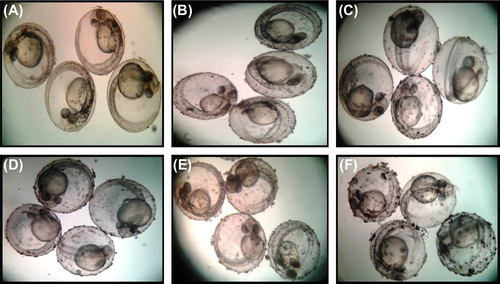
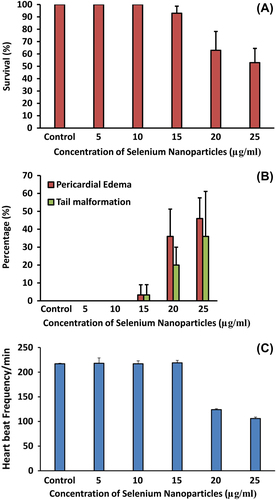
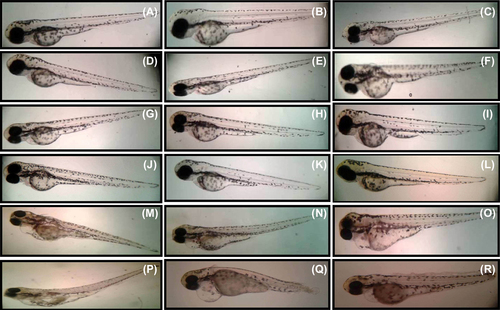
Effect of selenium nanoparticles on cardiovascular functions of zebrafish embryo
Several studies have shown that selenium may have a protective effect against cardiovascular disease (CitationNeve 1996). The exact link between selenium nanoparticles and cardiac dysfunction is not well known. A 2–3 fold risk of cardiovascular dysfunction was found to be associated with serum selenium concentrations below 45 μg/l (CitationSalonen et al. 1982). Another study found a significant inverse correlation between toenail selenium content and the risk of myocardial infarctions, but only in the population with the lowest level of selenium (CitationKardinaal et al. 1997) A lower concentration of selenium can play a significant role in cardiovascular prevention and possible therapy (CitationTiekink 2012). Before evaluating the therapeutic potential of selenium nanoparticles, it is important to evaluate cardio toxicity in zebrafish embryos. The present study suggests that the direct effect of heart rate was also greatly reduced in the embryos treated with higher concentrations of SeNPs (). Zebrafish embryos treated with 20–25 μg/ml SeNPs exhibited blood congestion at the cardiac inflow tract and moderate pericardial edema which is an early feature of cardiac dysfunction (). Video recording is used to assess blood regurgitation and heart arrhythmias between the atrium and ventricle for analysis of selenium nanoparticle-treated zebra fish embryos (see attached supplementary videos 1–3 to be found online at http://informahealthcare.com/doi/abs/10.3109/21691401.2014.962744). The present findings indicate that very low concentration of SeNPs could be useful as therapeutic molecules for cardiovascular prevention.
Conclusion
A novel, simple method for the synthesis of selenium nanoparticles in the range of 500 nm using BSA is proposed. The selenium nanoparticles prepared by this method are highly stable as well as economical. Due to their biological properties, the selenium nanoparticles could play a significant role in future biomedical applications. Toxicity studies, which are necessary for identifying the therapeutic concentrations, were done using zebrafish embryos. The results indicate that SeNPs at 5–10 μg/ml could be an economical therapeutic alternative for cardiovascular diseases.
Supplementary material available online
ianb_a_962744_sm1140.zip
Download Zip (13 MB)Acknowledgments
The authors thank Kalasalingam University for the facilities provided. Mr. A. Raja of Karunya University, Coimbatore is acknowledged for his help with SEM & EDX analysis.
Declaration of interest
The authors report no declarations of interest. The authors alone are responsible for the content and writing of the paper.
References
- Au L, Lim B, Colletti P, Jun YS, Xia Y. 2010. Synthesis of gold microplates using bovine serum albumin as a reductant and a stabilizer. Asian J Chem. 5:123–129.
- Bai W, Zhang Z, Tian W, He X, Ma Y, Zhao Y, Chai Z. 2010. Toxicity of zinc oxide nanoparticles to zebrafish embryo: a physicochemical study of toxicity mechanism. J Nanopart Res. 12:1645–1654.
- Barbazuk WB, Korf I, Kadavi C, Heyen J, Tate S, Wun E, et al. 2000. The syntenic relationship of the zebrafish and human genomes. Genome Res. 10:1351–1358.
- Blum JS, Stolz JF, Oren A, Oremland RS. 2001. Selenihalanaerobacter shriftii gen. nov., sp. nov., a halophilic anaerobe from Dead Sea sediments that respires selenate. Arch Microbiol. 175:208–219.
- Carter DC, Ho JX. 1994. Structure of serum albumin. Adv Protein Chem. 45:153–203.
- Charles MD, Elizabeth JD, Claudia MM, Peter S, Konrad P, Iona K, et al. 2011. A bacterial process for selenium nanosphere assembly. PNAS. 108:13480–13485.
- Dhanjal S, Cameotra SS. 2010. Aerobic biogenesis of selenium nanospheres by Bacillus cereus isolated from coalmine soil. Microb Cell Fact. 9:52.
- Duan J, Yu Y, Shi H, Tian L, Guo C, Huang P, et al. 2013. Toxic effects of silica nanoparticles on zebrafish embryos and larve. PLoS One. 8:e74606.
- He XM, Carter DC. 1992. Atomic structure and chemistry of human serum albumin. Nature. 358:209–215.
- Huang Y, He L, Liu W, Fan C, Zheng W, Wong YS, Chen T. 2013. Selective cellular uptake and induction of apoptosis of cancer-targeted selenium nanoparticles. Biomaterials. 34:7106–7116.
- Kalimuthu K, Suresh Babu R, Venkataraman D, Bilal M, Gurunathan S. 2008. Biosynthesis of silver nanocrystals by Bacillus licheniformis. Colloids Surf B Biointerfaces. 65:150–153.
- Kalishwaralal K, Deepak V, Ram Kumar Pandian S, Kottaisamy M, Barathmanikanth S, Kartikeyan B, Gurunathan S. 2010. Biosynthesis of silver and gold nanoparticles using Brevibacterium casei. Colloid Surf B Biointerfaces. 77:257–262.
- Kardinaal AF, Kok FJ, Kohlmeier L, Martin-Moreno JM, Ringstad J, Gomez-Aracena J, et al. 1997. Association between toenail selenium and risk of acute myocardial infarction in European men. The EURAMIC Study. European Antioxidant Myocardial Infarction and Breast Cancer. Am J Epidemiol. 145:373–379.
- Klayman DL, Gunter WHH. 1973. Organic Selenium Compounds-Their Chemistry and Biology. New York: John Wiley & Sons, Inc., pp. 223–243.
- Li Y, Li X, Zheng W, Fan C, Zhang Y, Chen T. 2013. Functionalized selenium nanoparticles with nephroprotective activity, the important roles of ROS-mediated signaling pathways. J Mater Chem B. 1:6365–6372.
- Mónica FU, Laura TA, José MCF, Rosario P, Alfredo Sanz M. 2013. One-step aqueous synthesis of fluorescent copper nanoclusters by direct metal reduction. Nanotechnology. 24:495601.
- Narmato K. 1997. Infrared and Raman spectra of Inorganic and Coordination compounds. New York: John Wiley & Sons, Inc., pp. 296–346.
- Neve J. 1996. Selenium as a risk factor for cardiovascular diseases. J Cardiovasc Risk. 3:42–47.
- Oremland RS, Mitchell JH, Jodi SB, Sean L, Terry JB, et al. 2004. Structural and spectral features of selenium nanospheres produced by se-respiring bacteria. Appl Environ Microbiol. 70:52–60.
- Salonen JT, Alfthan G, Huttunen JK, Pikkarainen J, Puska P. 1982. Association between cardiovascular death and myocardial infarction and serum selenium in a matched-pair longitudinal study. Lancet. 2:175–179.
- Schrade A, Landfester K, Ziener U. 2013. Pickering-type stabilized nanoparticles by heterophase polymerization. Chem Soc Rev. 42:6823–6839.
- Soflaei S, Dalimi A, Abdoli A, Kamali M, Nasiri V, Shakibaie M, Tat M. 2014. Anti-leishmanial activities of selenium nanoparticles and selenium dioxide on Leishmania infantum. Comp Clin Path. 23:15–20
- Sriram MI, Mani Kanth SB, Kalishwaralal K, Gurunathan S. 2010. Antitumor activity of silver nanoparticles in Dalton's lymphoma ascites tumor model. Int J Nanomedicine. 5:753–762.
- Srivastava N, Mukhopadhyay M. 2013. Biosynthesis and structural characterization of selenium nanoparticles mediated by Zooglea ramigera. Powder Technol. 244:26–29.
- Stoppe C, Spillner J, Rossaint R, Coburn M, Schälte G, Wildenhues A, et al. 2013. Selenium blood concentrations in patients undergoing elective cardiac surgery and receiving perioperative sodium selenite. Nutrition. 29:158–165.
- Suadicani P, Hein H, Gyntelberg F. 1992. Serum selenium concentration and risk of ischaernic heart disease in a prospective cohort study of 3000 males. Atherosclerosis. 96:33–42.
- Switzer BJ, Burns BA, Buzzelli J, Stolz JF, Oremland RS. 1998. Bacillus arsenicoselenatis, sp. nov., and Bacillus selenitireducens, sp. nov.: two haloalkaliphiles from Mono Lake, California that respire oxyanions of selenium and arsenic. Arch Microbiol. 171:19–30.
- Syed A, Saraswati S, Kundu GC, Ahmad A. 2013. Biological synthesis of silver nanoparticles using the fungus Humicola sp. and evaluation of their cytoxicity using normal and cancer cell lines. Spectrochim Acta A Mol Biomol Spectrosc. 114:144–147.
- Tiekink ER. 2012. Therapeutic potential of selenium and tellurium compounds: opportunities yet unrealised. Dalton Trans. 41: 6390–6395.
- Tran PA, Webster TJ. 2011. Selenium nanoparticles inhibit Staphylococcus aureus growth. Int J Nanomedicine. 6:1553–1558.
- Zhang W, Chen Z, Liu H, Zhang L, Gao P, Li D. 2011. Biosynthesis and structural characteristics of selenium nanoparticles by Pseudomonas alcaliphila. Colloids Surf B Biointerfaces. 88: 196–201.