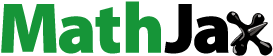
Abstract
The present study is designed to explore the localized delivery of fluconazole using mucoadhesive polymeric nanofibers. Drug-loaded polymeric nanofibers were fabricated by the electrospinning method using polyvinyl alcohol (PVA) as the polymeric constituent. The prepared nanofibers were found to be uniform, non-beaded and non-woven, with the diameter of the fibers ranging from 150 to 180 nm. Further drug release studies indicate a sustained release of fluconazole over a period of 6 h. The results of studies on anti-microbial activity indicated that drug-loaded polymeric nanofibers exhibit superior anti-microbial activity against Candida albicans, when compared to the plain drug.
Introduction
Recently, polymeric nanofibers have been extensively studied for their potential application in the delivery of biopharmaceuticals (CitationChaudhary et al. 2014, CitationSingh et al. 2014a). The electrospinning technique allows the convenient preparation of non-woven fibrous materials (CitationOhkawa et al. 2004, CitationGagandeep et al. 2014) with interesting characteristics such as fine diameters (ranging from submicron values to several nanometers) (CitationBuchko et al. 1999, CitationModgill et al. 2014), large surface area per unit mass (CitationMorie et al. 2014), high porosity (CitationSingh et al. 2014c), high gas permeability (CitationSingh et al. 2014b), and small pore size of the fiber (CitationSaeed et al. 2008). A typical electrospinning setup usually includes a reservoir of polymer solution with a metallic capillary connected to high voltage, and a metallic collector. The hemispheric shape of the droplet at the tip of the needle is destabilized by the charge accumulated on the surface, and is converted to a Taylor's cone when high voltage is applied to the polymer solution. At a critical value of voltage, the electric forces overcome the surface tension on the droplet and a jet of ultra-fine fibers is produced from the tip of the Taylor cone (CitationTaylor 1969, CitationSharma et al. 2014). The variables controlling the behavior of the electrified fluid jet during electrospinning can be classified as fluid properties and operating parameters. The relevant fluid properties are viscosity (CitationBaumgarten 1971), conductivity, dielectric constant, boiling point, and surface tension. The operating parameters are flow rate, applied electric potential, and the distance between the tip and the collector, called an air gap (CitationReneker and Chun 1996, CitationShin et al. 2001). These nanofibers hold great promise as excellent candidates for various biomedical applications, for example, as scaffolds for cell and tissue culture (CitationKwon et al. 2005, CitationLi et al. 2005, CitationGarg et al. 2012b), for vascular grafts (CitationMa et al. 2005, CitationGoyal et al. 2013a), and as carriers for drug delivery (CitationShin et al. 2001, CitationJoshi et al. 2014). For many biomedical applications, the most important characteristics that should be targeted include biocompatibility and mechanical performance (CitationRohilla et al. 2014, CitationParnami et al. 2013). PVA is a water-soluble synthetic polymer. PVA as a hydrophilic polymer is water-soluble and represents the largest volume of synthetic resin produced in the world (CitationBriscoe et al. 2000). The excellent chemical resistance, physical properties and biodegradability of PVA have led to the development of many commercial products based on this polymer. PVA is a truly biodegradable polymer, with the degradation products being water and carbon dioxide. Hence, it is used in many biomedical and pharmaceutical applications, due to its advantages such as nontoxicity, non carcinogenicity and bioadhesive characteristics, with ease of processing (CitationCai and Gupta 2000).
Complicated fungal vaginitis (CitationInagaki et al. 1992, CitationMonif 1985) requires a 6-month course of fluconazole taken once in a week (150 mg), and is associated with a number of side effects including systemic toxicity (CitationGlover and Larsen 2003), issues related to hepatotoxicity, slower and longer therapy (CitationDennerstein 1998), higher dose, and low therapeutic index (CitationSobel et al. 1998, CitationBeigi et al. 2004). In addition to these, poor aqueous solubility and impaired bioavailability of fluconazole provide a steep challenge for the formulation of a strategy to develop a dosage form with improved therapeutic outcomes. To address the aforesaid challenges, the current strategy is oriented towards localized drug delivery using polymeric nanofibers, particularly against infections at mucosal sites (CitationLala et al. 2007), to achieve improved therapeutic outcomes with reduced untoward effects. The solubilization potential of PVA and the specialized features of nanofibers, made use of in the present strategy, may further improve the antimicrobial potential of fluconazole. For the present study, drug-loaded polymeric nanofibers were prepared. The effects of parameters related to the process and formulation, on the physical characters of nanofibers, were determined. The antimicrobial potential of the formulation developed was determined against the infectious species and the common shell bacteria, to establish its therapeutic potential.
Materials and methods
Materials
Fluconazole was supplied by Ind Swift Pvt Ltd (India) as a gift sample. PVA was purchased from Central Drug House (P) Ltd. The fungal strain (Candida albicans) (MTCC 7253) and the bacterial strain (Lactobacillus acidophilus) (MTCC 10307) were purchased from IMTECH, Chandigarh. All the solvents used were of analytical grade.
Preparation of polymer solutions
Aqueous PVA solutions, ranging in concentration from 8% to 12%, were prepared by dissolving the required amount of PVA in 10 ml of distilled water, with constant stirring for up to 3 h. In order to achieve clear PVA solutions, the solutions were stirred overnight a using magnetic stirrer (IKA T25 DS22, Cole Parmer Pvt. Ltd., India). They were then subjected to electrospinning (e-nano, IIT Kanpur) (CitationZhang et al. 2005).
Preparation of nanofiber mats
The polymer solutions were placed into a 5-mL syringe fitted to a needle with a tip diameter of 22 gauges (inner diameter 0.413 mm), and the syringe was then placed in the electrospinning apparatus. A syringe pump was used for delivering the polymer solution at a rate between 0.1 and 0.2 ml/h. Electrospinning was carried out at a voltage varying from 8 to 12 kV and with a needle to collector distance of 15 cm. The collector was covered with an aluminum foil on which the nanofibers were collected. The electrospinning voltage was supplied directly by a high DC voltage power supply.
Preparation of drug-loaded nanofiber mats
The drug-loading was carried out by a passive loading technique. Fluconazole was dissolved in the polymeric solution prior to electrospinning. Subsequently, a saturated solution of fluconazole in ethanol was added to the polymer solution and the mixture was stirred for 48 h at room temperature, to obtain a clear homogenous solution. The solution was then electrospun using the spinning parameters defined above. The drug-loaded nanofibers were collected and dried overnight under vacuum at room temperature.
Characterization of electrospun nanofibers
Morphology analysis
The morphology of the electrospun fibers and the diameter of the fibers were examined by scanning electron microscopy (SEM). At least 10 different positions on the fiber mat were tested to measure the diameter of the electrospun fibers. A small section of the fiber mat was placed on the SEM sample holder and sputter-coated with platinum. An accelerating voltage of 15 kV was employed to take the SEM images. The SEM images were then used to determine the average diameter of the fibers (CitationZhang et al. 2005, CitationKaur et al. 2014d).
Drug–polymer compatibility studies
The compatibility of the drug and polymer in the drug-loaded PVA nanofibers was observed using an IR spectrometer (Perkin-Elmer Spectrum RX1). The electrospun nanofibers were cut into small pieces and mixed with KBR to make sample pellets. Measurements were taken in the range between 4000 and 800 cm− 1, with a resolution of 2 cm− 1 (CitationKaur et al. 2014b).
Differential scanning calorimetry of drug-loaded nanofibers
The melting point of drug-loaded nanofibers was determined by using differential scanning calorimetry (DSC) (DSC 1 STAR system; Mettler Toledo-USA). The samples were first weighed in a crucible; the crucible was then covered and pressed using a pressing machine. Then, the crucible was fitted inside the instrument at the place marked for the sample. The desired gas was run and the samples analyzed, using the software control. Samples weighing 2–5 mg were studied using DSC, under a nitrogen atmosphere,with a scanning speed of 10°C/min, and at temperatures ranging from 0 to 250°C (CitationKaur et al. 2014a, CitationKataria et al. 2014).
Degree of swelling
The degree of swelling of the nanofibers developed was calculated by equation (1). The test was carried out using vaginal fluid simulant (VFS), in pH 4.2, at 37°C.
where M is the weight of swollen nanofiber sample which is wiped dry with filter paper, and Md is the dried mass of the sample immersed in buffer medium, which is measured by drying the swollen nanofiber mats in an oven at 40°C until a constant weight is reached (CitationKaur et al. 2014c).
In vitro release studies
Drug release from the electrospun nanofiber mats was carried out by placing a pre-defined size of nanofiber (1 × 1 cm2) in 100 mL of VFS. The release studies were carried out at 37°C, at 100 rpm in a thermostatic shaking incubator. The amount of drug present in the aliquots was determined at pre-defined intervals using a UV–visible spectrophotometer (UV-1700 Pharma Spec, Shimadzu, Japan) at a λmax of 261 nm (CitationMarwah et al. 2014, CitationKaur et al. 2014e), followed by the addition of fresh media to maintain sink conditions.
Drug entrapment efficiency
The drug entrapment efficiency of nanofibers was calculated by drying the drug-loaded nanofibers at 40°C, and then dissolving a nanofiber mat of known area (1 × 1 cm2) in its respective solvent (water, in the case of PVA nanofiber). The amount of drug in the respective solutions was calculated using a UV spectrophotometer (CitationJohal et al. 2014, CitationGoyal et al. 2013b, Citation2014). The entrapment efficiency was calculated by using the following equation:
Mucoadhesive test
The mucoadhesive strength of the formulation developed was determined using the Brookfield texture analyzer. In this study, goat vaginal mucosa with a diameter of 1.2 cm was attached to an attachment of the device with the help of a double-sided tape. Next, nanofibers with the same diameter were stuck to the other attachment. Both of these were kept in contact by applying a weight of 1000 gm for 15 s. After that, the force needed for the fibers to detach from the skin was calculated (CitationGarg et al. 2013).
Tensile strength of nanofibers
The tensile strength of nanofibers was determined using the Brookfield texture analyzer. Nanofibers having 6 cm length and 3 cm width were placed in a vertical position along the axis of the analyzer, with the help of clamps. The instrument was then allowed to run with a minimum sensitivity force of 3 gm/cm2. After that, the force required to split the mat into two pieces was determined (CitationGarg et al. 2012a, Citation2014a, Citation2014b).
Antimicrobial activity
The antimicrobial activity of the optimized formulation was determined against the causative organism of vaginal candidiasis (Candida albicans) and common shell bacteria (Lactobacillus acidophilus), to establish the therapeutic potential of the formulation developed. The fungal strain (MTCC 7253) and the bacterial strain (MTCC 10307) were obtained from IMTECH, Chandigarh. A quantity of 100 μl of the fungal suspension was streaked over the plate containing potato dextrose agar medium and was spread uniformly. A blank polymeric nanofiber with a size of 1 × 1 cm2 used as vehicle control, and drug-loaded nanofibers, were gently placed at the center of the solidified agar gel in different Petri dishes. The same test was repeated with Lactobacillus acidophilus in MRS media. The plates were incubated at 37°C for 48 h. The bacterial and fungal growth was compared with the controls (CitationGarg and Goyal 2014a, Citation2014b).
Results and discussion
Electro spinning of PVA aqueous blends
During the process of electrospinning, all the parameters which were involved in this process, including the concentration of polymer, applied voltage, flow rate and tip to collection distance (TCD), were very carefully optimized. The size ranged from 120 to 160 nm. In this study, the polymer solution (PVA) was electrospun under the applied electrical potential of 8–12 kV, over a TCD of 15 cm and a flow rate of 0.1 to 0.2 ml/h. The SEM micrograph illustrates the morphology of unloaded electrospun nanofibers (CitationHomayoni et al. 2009, CitationGarg and Goyal 2012, CitationGarg 2014).
Electrospinning of PVA yielded optimum fibers with an average diameter of 200–350 nm at 10% polymer concentration, under the applied electric potential (CitationDeitzel et al. 2001) of 12 kV, over a TCD of 15 cm. At a low concentration of PVA, the density of the fiber was very low, along with the formation of beads at regular intervals. As the concentration of PVA was increased, there was a gradual increase in the fiber density, with no droplet formation till a concentration of 10% was reached, beyond which constant droplet formation was observed. The observation clearly indicated that with an increase in the PVA concentration, there is an increase in the electrical force exerted on the jet, which in turn increases the mass throughput but results in the formation of drops. However, 10% PVA provides the optimum physical and electrical conditions under defined electrospinning parameters. The parameters were optimized on the basis of physical observation of fibers under a Motic optical microscope. The morphology of blank optimized fiber was observed using a scanning electron microscope. The formulation and process parameters that were optimized include a 10% concentration of PVA, an applied voltage of 12 kV, a flow rate of 0.1 ml/h, and a TCD of 15 cm, respectively.
Drug loading in the nanofiber mat
The drug (320 mg) was first dissolved in 3 ml of ethanol and the solution was then poured into 4 ml of 10% PVA solution. Here, the ethanol served as a cosolvent (CitationWannatong et al. 2004), to improve the solubility of fluconazole in the PVA solution. An increase in the viscosity and a decrease in the conductivity of the drug-loaded polymer solution led to the formation of beads, which can be attributed to a decrease in the voltage gradient across the TCD. In order to optimize the electrospinning conditions, the values of TCD and the potential applied were again varied and an optimal value was obtained to get bead-free, continuous, and thin nanofibers of high density (CitationRamakrishna et al. 2005). Optical microscopy of the drug-loaded nano fibers under the above specified spinning conditions showed beaded, non-uniform and woven nanofibers. This could be due to the change in polymer viscosity and conductivity after the addition of the ethanolic fluconazole solution. Therefore, the spinning parameters were further optimized for the fabrication of drug-loaded nano fibers. Smooth and uniform drug-loaded nano fibers were obtained at higher applied voltage, that is, 14 kV, when compared to the plain polymeric nano fibers obtained at 12 kV. This could be attributed to the higher viscosity and lower conductivity of the resultant drug-polymer solution, which requires a higher potential to form an electrical jet under the specified spinning conditions. Moreover, the lower conductivity may be attributed to the non-polar nature of the drug. In addition, the presence of alcohol facilitates the penetration of water into the polymeric nanostructure, which leads to the decrease in aqueous volume in the continuous phase, in turn decreasing the overall polarity and conductivity of the resultant solution. The optimized spinning conditions for the drug-loaded nano fibers were found to be an applied voltage of 14 kV and a TCD of 12 cm. From these results, we may infer that a higher applied voltage and lower TCD would increase the voltage gradient (CitationHeikkilä and Harlin 2008) across the electrode, to address the poor conductivity issues of the resultant drug–polymer solution, which would help to develop an electrical jet under the above spinning conditions. shows the optimization parameters and microscope observations of the different trials conducted on nanofibers.
Table I. Optimization parameters and microscope observations of different trials.
Formulation R3 was found to be optimal for drug-loaded nanofibers, resulting in the formation of non-woven, ultra-thin, high density nanofibers with no beads. The optical microscopy images of the final optimized drug-loaded nano fibers is seen in the following figures: .
Morphology of nanofibers (SEM)
Electrospinning of PVA yielded optimum fibers with an average diameter of 150–180 nm, at 10% polymer concentration under an applied electric potential of 14 kV, and over a TCD of 12 cm. At a low concentration of PVA, the density of the fiber was very low, along with the formation of beads at regular intervals. As the concentration of PVA was increased, there was a gradual increase in the fiber density, with no droplet formation till a concentration of 10% was reached, beyond which constant droplet formation was observed. The observation clearly indicated that with an increase in the PVA concentration, there is an increase in the electrical force exerted on the jet, which in turn increases the mass throughput, resulting in the formation of drops. However, 10% PVA provides optimum physical and electrical conditions under defined electrospinning parameters. and show SEM images of blank and drug-loaded nano fibers respectively.
Drug–polymer compatibility study
The infrared (IR) spectra of PVA as well as its corresponding electrospun nanofibers are shown in . The IR spectra were obtained and studied for any kind of interactions between their functional groups. All the characteristic peaks of drug and polymer found in the IR spectra were found to be concordant with the standard peaks. This result shows that there was no change in the wave number of functional groups, indicating the stability of nanofibers with the polymer. Hence, it can be concluded that there is no incompatibility between the drug and the polymer.
DSC of drug-loaded nano fibers
shows the DSC thermograms of PVA drug-loaded nanofibers prepared by electrospinning. One peak was observed at 196.20°C, as a characteristic peak of PVA, and another peak was observed at 142.40°C, showing the presence of fluconazole in the nanofibers and indicating the stability of the PVA nanofiber. These peaks have shown the stability of polymer as well as the drug in the nanofibers. Further, the slight decrease in the melting point of the drug in the electrospun nanofibers may be attributed to the amorphous state of the drug. The distinguished peak of the drug and polymer further indicate that there is no chemical interaction between the drug and polymer. shows the DSC of drug-loaded nanofibers.
Degree of swelling
The degree of swelling of a nanofiber mat plays a major role in the release of drug from the nanofiber mat. The results of swelling index study of the nanofibrous mat in VFS indicated that the swelling index of blank nano fibers is more than that of drug-loaded nano fibers. graphically represents the difference in the degree of swelling between the blank and drug-loaded nanofibers, at different time intervals.
The degree of swelling was found to be 125% and 115% for blank and drug-loaded nanofibers respectively, after 1 h, which increased to 195% and 185% after 4 h. This can be correlated to the average diameter of these fibers. The fibers with the finest diameter showed maximum swelling, and vice versa. However, it was observed that after 4 h, the swelling index decreased, which after 6 h was found to be 171% and 154% for blank and drug-loaded nanofibers respectively. This could be due to the fact that the polymer is soluble in water, and hence, after 4 h, the polymer was being eroded from the nanofiber mat, thereby diminishing its ability to swell in VFS.
In vitro release studies
An in vitro drug release study was conducted to determine the optimized formulation in VFS with a pH of 4.2. The graphic representation of data obtained from the in vitro release study of the drug-loaded nanofibers has been shown in . The release study was carried out in a Franz diffusion cell using the vaginal skin of goat, for permeation of the drug. The study was carried out in triplicate. There was an initial burst release of about 35.13% of the drug in the first 2 h, followed by a slower release pattern of 97.8% for up to 6 h. The initial burst release may be attributed to the enormous surface area of the nanofibers, followed by slower release, as the drug is also present in the core of the nanofibers, which is governed by both diffusion and erosion mechanisms. The reference data is also supported by the swelling studies, where the degree of swelling was found to be maximum after 4 h, indicating an extensive diffusion process. Further, the erosion of polymer facilitates the transport of drug from the nanofibers, which was also indicated by the swelling index which was decreased after 4 h, indicating the erosion of polymer in VFS. shows the cumulative release pattern of the drug with respect to time.
Drug entrapment efficiency
The drug entrapment efficiency of the optimized formulation was found to be 93.7%. This could be attributed to the fact that all nanofibers were not collected at the collector site, as some of them were deposited on other conducting surfaces of the equipment. This could be due to the decreasing potential drainage capacity of collector as the fiber gets deposited on the surface of the collector, leading to the distraction of fibers from the collector site.
Mucoadhesive test
The mucoadhesive detachment force for drug-loaded nanofibers was found to be 190 gm/cm2, and 245 gm/cm2 for blank nanofibers. The lower value of mucoadhesive force in the case of drug-loaded nanofibers can be attributed to the decrease in specific area associated with a wider diameter of drug-loaded nanofibers than that of the blank nanofibers. Moreover, the presence of the hydrophobic drug on the surface of the nanofiber reduces the hydrophilicity of the drug-loaded nano fibers (CitationHeikkilä and Harlin 2008). The polymeric nanofiber with drug has less effective specific surface area in contact with the mucosal site, resulting in the decreased mucoadhesive detachment force.
Tensile strength of nanofibers
The result of studies on mechanical strength indicated that the drug-loaded nanofibers (53 g/cm2) exhibit better mechanical strength than the plain polymeric nanofibers (45 g/cm2). This could be attributed to partial congealing of PVA caused by ethanol. During the preparation of the drug/polymer solution, ethanol, due to its low surface tension, rises quickly to the surface of the polymer, which leads to displacement of water from the polymer surface. It results in the formation of an extra hydrogen bond, which could be attributed to the higher mechanical strength of the drug-loaded polymeric nanofibers.
Anti-microbial activity
The zones of inhibition by the optimized formulation against Candida albicans and Lactobacillus acidophilus were compared with those of the plain drug, as shown in .
Table II. Table showing zones of inhibition of various formulations on Candida albicans and Lactobacillus acidophilus.
The higher activity of the nanofibers can be attributed to the fact that PVA here acted as a permeation enhancer, facilitating the drug release as well as drug permeability across microbial membranes. Moreover, the electrospinning process enables the phase transition of the drug, as during this process, the liquid state changes into solid state in fractions of second, resulting in the phase transformation of the drug from the crystalline state to an amorphous state, This hypothesis is also supported by CitationMegelski et al. (2002), who reported the phase transformation of the drug in electrospun nanofibers. As the drug is solely anti-fungal in nature, it did not show any anti-bacterial activity. These activities cleared the fact that the formulation will only affect the population of fungus in the vagina and will not affect the common shell bacteria which exist as the normal vaginal flora.
Conclusion
The electrospinning process serves as a simple unit operation process for the fabrication of nanofibers. Further, the selection of polymer and optimization of spinning conditions can play an important role in achieving the therapeutic outcomes. Above all, electrospun nanofibers could open a new plethora in pharmaceutical carrier systems to treat microbial infections at the local sites, thereby reducing the systemic toxicity of anti-microbial drugs. Unit operation steps involved in the electrospinning process make the fabrication process simple, reproducible and scalable.
Acknowledgement
The authors, Mr. Goutam Rath & Dr. Amit K. Goyal, are thankful to Department of Biotechnology (DBT), New Delhi, INDIA for providing financial assistance to carry out research.
Declaration of interest
The authors report no declarations of interest. The authors alone are responsible for the content and writing of the paper.
References
- Baumgarten PK. 1971. Electrostatic spinning of acrylic microfibers. J Colloid Interface Sci. 36:71–79.
- Beigi RH, Meyn LA, Moore DM, Krohn MA, Hillier SL. 2004. Vaginal yeast colonization in nonpregnant women: a longitudinal study. Obstet Gynecol. 104:926–930.
- Briscoe B, Luckham P, Zhu S. 2000. The effects of hydrogen bonding upon the viscosity of aqueous poly(vinyl alcohol) solutions. Polymer. 41:3851–3860.
- Buchko CJ, Chen LC, Shen Y, Martin DC. 1999. Processing and microstructural characterization of porous biocompatible protein polymer thin films. Polymer. 40:7397–7407.
- Cai W, Gupta RB. 2000. Hydrogels. In: Kirk-Othmer Encyclopedia of Chemical Technology. New York: John Wiley & Sons, Inc: 367–410.
- Chaudhary S, Garg T, Murthy RS, Rath G, Goyal AK. 2014. Recent approaches of lipid-based delivery system for lymphatic targeting via oral route. J Drug Target. 1–12.
- Deitzel JM, Kleinmeyer J, Harris D, Beck Tan NC 2001. The effect of processing variables on the morphology of electrospun nanofibers and textiles. Polymer. 42:261–272.
- Dennerstein G. 1998. Pathogenesis and treatment of genital candidiasis. Aust Fam Physician. 27:363–369.
- Gagandeep Garg T., Malik B, Rath G, Goyal AK. 2014. Development and characterization of nano-fiber patch for the treatment of glaucoma. Eur J Pharm Sci. 53:10–16.
- Garg T. 2014. Current nanotechnological approaches for an effective delivery of bio-active drug molecules in the treatment of acne. Artif Cells Nanomed Biotechnol. 1–8.
- Garg T, Goyal AK. 2012. Iontophoresis: Drug delivery system by applying an electrical potential across the skin. Drug Deliv Lett. 2:270–280.
- Garg T, Goyal AK. 2014a. Biomaterial-based scaffolds–current status and future directions. Expert Opin Drug Deliv. 11:767–789.
- Garg T, Goyal AK. 2014b. Liposomes: Targeted and controlled delivery system. Drug Deliv Lett. 4:62–71.
- Garg T, Goyal AK, Arora S, Murthy R. 2012a. Development, optimization & evaluation of porous chitosan scaffold formulation of gliclazide for the treatment of type-2 diabetes mellitus. Drug Deliv Lett. 2:251–261.
- Garg T, Rath G, Goyal AK. 2014a. Ancient and advanced approaches for the treatment of an inflammatory autoimmune disease-psoriasis. Crit Rev Ther Drug Carrier Syst. 31:331–364.
- Garg T, Rath G, Goyal AK. 2014b. Comprehensive review on additives of topical dosage forms for drug delivery. Drug Deliv.
- Garg T, Singh O, Arora S, Murthy R. 2012b. Scaffold: a novel carrier for cell and drug delivery. Crit Rev Ther Drug Carrier Syst. 29:1–63.
- Garg T, Singh S, Goyal AK. 2013. Stimuli-sensitive hydrogels: an excellent carrier for drug and cell delivery. Crit Rev Ther Drug Carrier Syst. 30:369–409.
- Glover DD, Larsen B. 2003. Relationship of fungal vaginitis therapy to prior antibiotic exposure. Infect Dis Obstet Gynecol. 11:157–160.
- Goyal AK, Rath G, Garg T. 2013a. Nanotechnological approaches for genetic immunization. In: Erdmann VA & Barciszewski J, Eds. DNA and RNA Nanobiotechnologies in Medicine: Diagnosis and Treatment of Diseases Berlin: Springer, pp. 67–120.
- Goyal G, Garg T, Malik B, Chauhan G, Rath G, Goyal AK. 2013b. Development and characterization of niosomal gel for topical delivery of benzoyl peroxide. Drug Deliv.
- Goyal G, Garg T, Rath G, Goyal AK. 2014. Current nanotechnological strategies for an effective delivery of drugs in treatment of periodontal disease. Crit Rev Ther Drug Carrier Syst. 31:89–119.
- Heikkilä P, Harlin A. 2008. Parameter study of electrospinning of polyamide-6. Eur Polym J. 44:3067–3079.
- Homayoni H, Ravandi SAH, Valizadeh M. 2009. Electrospinning of chitosan nanofibers: processing optimization. Carbohydr Polym. 77:656–661.
- Inagaki K, Takagi J, Lor E, Okamoto MP, Gill MA. 1992. Determination of fluconazole in human serum by solid-phase extraction and reversed-phase high-performance liquid chromatography. Ther Drug Monit. 14:306–311.
- Johal HS, Garg T, Rath G, Goyal AK. 2014. Advanced topical drug delivery system for the management of vaginal candidiasis. Drug Deliv.1–14.
- Joshi D, Garg T, Goyal AK, Rath G. 2014. Advanced drug delivery approaches against periodontitis. Drug Deliv. 1–15.
- Kataria K, Sharma A, Garg T, Goyal AK, Rath G. 2014. Novel technology to improve drug loading in polymeric nanofibers. Drug Deliv Lett. 4:79–86.
- Kaur M, Garg T, Rath G, Goyal AK. 2014a. Current nanotechnological strategies for effective delivery of bioactive drug molecules in the treatment of tuberculosis. Crit Rev Ther Drug Carrier Syst. 31:49–88.
- Kaur M, Malik B, Garg T, Rath G, Goyal AK. 2014b. Development and characterization of guar gum nanoparticles for oral immunization against tuberculosis. Drug Deliv.
- Kaur P, Garg T, Rath G, Murthy RS, Goyal AK. 2014c. Surfactant-based drug delivery systems for treating drug-resistant lung cancer. Drug Deliv. 1–12.
- Kaur R, Garg T, Das Gupta U., Gupta P, Rath G, Goyal AK. 2014d. Preparation and characterization of spray-dried inhalable powders containing nanoaggregates for pulmonary delivery of anti-tubercular drugs. Artif Cells Nanomed Biotechnol.1–6.
- Kaur R, Garg T, Malik B, Gupta UD, Gupta P, Rath G, Goyal AK. 2014e. Development and characterization of spray-dried porous nanoaggregates for pulmonary delivery of anti-tubercular drugs. Drug Deliv.1–6.
- Kwon IK, Kidoaki S, Matsuda T. 2005. Electrospun nano- to microfiber fabrics made of biodegradable copolyesters: structural characteristics, mechanical properties and cell adhesion potential. Biomaterials. 26:3929–3939.
- Lala NL, Ramaseshan R, Bojun L, Sundarrajan S, Barhate RS, Ying-Jun L, Ramakrishna S. 2007. Fabrication of nanofibers with antimicrobial functionality used as filters: protection against bacterial contaminants. Biotechnol Bioeng. 97:1357–1365.
- Li W-J, Tuli R, Huang X, Laquerriere P, Tuan RS. 2005. Multilineage differentiation of human mesenchymal stem cells in a three- dimensional nanofibrous scaffold. Biomaterials. 26:5158–5166.
- Ma Z, Kotaki M, Yong T, He W, Ramakrishna S. 2005. Surface engineering of electrospun polyethylene terephthalate (PET) nanofibers towards development of a new material for blood vessel engineering. Biomaterials. 26:2527–2536.
- Marwah H, Garg T, Goyal AK, Rath G. 2014. Permeation enhancer strategies in transdermal drug delivery. Drug Deliv. 1–15.
- Megelski S, Stephens JS, Chase DB, Rabolt JF. 2002. Micro- and nanostructured surface morphology on electrospun polymer fibers. Macromolecules. 35:8456–8466.
- Modgill V, Garg T, Goyal AK, Rath G. 2014. Permeability study of ciprofloxacin from ultra-thin nanofibrous film through various mucosal membranes. Artif Cells Nanomed Biotechnol. 1–6.
- Monif GR. 1985. Classification and pathogenesis of vulvovaginal candidiasis. Am J Obstet Gynecol. 152:935–939.
- Morie A, Garg T, Goyal AK, Rath G. 2014. Nanofibers as novel drug carrier – An overview. Artif Cells Nanomed Biotechnol. 1–9.
- Ohkawa K, Cha D, Kim H, Nishida A, Yamamoto H. 2004. Electrospinning of chitosan. Macromol Rapid Commun. 25:1600–1605.
- Parnami N, Garg T, Rath G, Goyal AK. 2013. Development and characterization of nanocarriers for topical treatment of psoriasis by using combination therapy. Artif Cells Nanomed Biotechnol.
- Ramakrishna S, Fujihara K, Teo WE, Lim TC, Ma Z. 2005. An Introduction to Electrospinning and Nanofibers, vol. 1. New Jersey: World Scientific, pp. 91–101.
- Reneker DH, Chun I. 1996. Nanometre diameter fibers of polymer, produced by electrospinning. Nanotechnology. 7:216–223.
- Rohilla R, Garg T, Goyal AK, Rath G. 2014. Herbal and polymeric approaches for liver-targeting drug delivery: novel strategies and their significance. Drug Deliv. 1–17.
- Saeed K, Haider S, Oh TJ, Park SY. 2008. Preparation of amidoxime-modified polyacrylonitrile (PAN-oxime) nanofibers and their applications to metal ions adsorption. J. Membr Sci. 322:400–405.
- Sharma R, Singh H, Joshi M, Sharma A, Garg T, Goyal AK, Rath G. 2014. Recent advances in polymeric electrospun nanofibers for drug delivery. Crit Rev Ther Drug Carrier Syst. 31:187–217.
- Shin YM, Hohman MM, Brenner MP, Rutledge GC. 2001. Experimental characterization of electrospinning: the electrically forced jet and instabilities. Polymer. 42:09955–09967.
- Singh B, Garg T, Goyal AK, Rath G. 2014a. Recent advancements in the cardiovascular drug carriers. Artif Cells Nanomed Biotechnol.1–10.
- Singh H, Sharma R, Joshi M, Garg T, Goyal AK, Rath G. 2014b. Transmucosal delivery of Docetaxel by mucoadhesive polymeric nanofibers. Artif Cells Nanomed Biotechnol.
- Singh O, Garg T, Rath G, Goyal AK. 2014c. Microbicides for the treatment of sexually transmitted HIV infections. J Pharm. 1–18.
- Sobel JD, Faro S, Force RW, Foxman B, Ledger WJ, Nyirjesy PR, et al. 1998. Vulvovaginal candidiasis: epidemiologic, diagnostic, and therapeutic considerations. Am J Obstet Gynecol. 178:203–211.
- Taylor GI. 1969. Electrically driven jets. Proc R Soc Lond A. 313: 453–475.
- Wannatong L, Sirivat A, Supaphol P. 2004. Effects of solvents on electrospun polymeric fibers: preliminary study on polystyrene. Polym Int. 53:1851–1859.
- Zhang C, Yuan X, Wu L, Han Y, Sheng J. 2005. Study on morphology of electrospun poly(vinyl alcohol) mats. Eur Polym J. 41:423–432.