Abstract
Metal nanoparticles, such as nanoparticles synthesized using gold, have numerous uncommon chemical and physical properties due to the effects of their quantum size and their large surface area, in comparison with other metal atoms or bulk metal. Gold nanoparticles (GNPs), in particular, are very attractive because of their size and shape-dependent properties. Metal nanoparticles have gathered extensive attention due to their uncommon properties and promising applications in photonics, electronics, biochemical sensing, and imaging. This review covers recent advances in the synthesis of GNPs.
Introduction
Nanotechnology generally refers to a field of science and engineering dedicated to materials of sizes ranging from 1–100 nm (CitationMody et al. 2010, CitationSalata 2004). The term ‘nano’ is extracted from the Greek word ‘dwarf ’, which means ‘extremely small’. When used as a prefix, it means 10 − 9 or 0.000000001. A nanometer (nm) is one billionth of a meter (CitationThakkar et al. 2010).
Nanoparticles are a unique group of materials with exceptional features and broad applications in various fields (CitationGeethalakshmi and Sarada 2012, CitationMatei et al. 2008).
In recent years, researchers have become increasingly interested in the synthesis of gold nanoparticles (AuNPs), because of their unique physical and chemical properties in wide areas like catalysis, biolabeling, nonlinear optical devices, and in the field of drug delivery (CitationPal et al. 2013, CitationDaniel and Astruc 2004).
AuNPs have exceptional optical properties due to surface plasmon resonance (SPR) effects. It is an optical phenomenon occurring from the interaction between an electromagnetic wave and the conduction of electrons in a metal. (CitationHu et al. 2006) The SPR properties of AuNPs makes them quite useful in the fields of bioimaging and biomedical therapeutics, and as biodiagnostic tools (CitationVerma et al. 2014, CitationJain et al. 2006).
AuNPs are being widely used in a variety of biomedical applications because of their compatibility of synthesis and functionalization, less toxicity, and facility of detection (CitationTiwari et al. 2011).
AuNPs can accumulate in the tumor cells and show optical scattering; thus, these nanoparticles can play an important role as a probe for the microscopy study of cancer cells. In addition, these nanoparticles can be used in chemotherapy and for the diagnosis of cancer (CitationTomar and Garg 2013, CitationCai and Chen 2007). The AuNPs also provide an applicable and promising scaffold for drug and gene delivery (CitationGhosh et al. 2008, CitationPaciotti et al. 2004, Citation2006).
This method comprises the assembly of atoms (produced by the reduction of ions) into desired nanostructures. Top-down techniques, such as photolithography and electron beam lithography (CitationShah et al. 2014, CitationSun et al. 2006, CitationSchaal et al. 2012), involve the removal of matter from the bulk material to get the desired nanostructure.
Synthesis of gold nanoparticles
A variety of techniques, including chemical, thermal, electrochemical and sonochemical pathways, have so far been introduced for the synthesis of GNPs (CitationMandal 2014, CitationPorta and Rossi 2003, CitationYu et al. 1997, CitationNakanishi et al. 2005).
Chemical method
Generally, the preparation of AuNPs by the chemical reduction method includes two main parts: (1) reduction by agents, for instance borohydrides, aminoboranes, formaldehyde, hydrazine, hydroxylamine, polyols, citric and oxalic acids, sugars, hydrogen peroxide, carbon monoxide, sulfites, hydrogen, acetylene, and ono electronic reducing agents including electron-rich transition-metal sandwich complexes; (2) stabilization using agents, for instance trisodium citrate dihydrate, sulfur ligands (in particular thiolates), phosphorus ligands, oxygen-based ligands, nitrogen-based ligands (including heterocyclic compounds), dendrimers, polymers and surfactants (in particular, cetyltrimethylammonium bromide (CTAB)). To avoid the aggregation of the particles, some kind of stabilizing agent is usually added (CitationZhao et al. 2013).
Turkevich method
One of the most well-known techniques for the synthesis of AuNPs is based on the reduction of HAuCl4 by citrate in water, which was first designed by Turkevich in 1951. In this method, the HAuCl4 solution is boiled, and the trisodium citrate dihydrate is then rapidly added into the boiling solution under vigorous stirring. After a few minutes, the color of the solution changes from light yellow to wine red. This method results in AuNPs measuring about 20 nm in diameter. In this technique, citrate ions play a double role, as both stabilizing and reducing agents (CitationHu et al. 2006, CitationTurkevich et al. 1951).
The schematic route for synthesis of AuNPs by the Turkevich method is shown in .
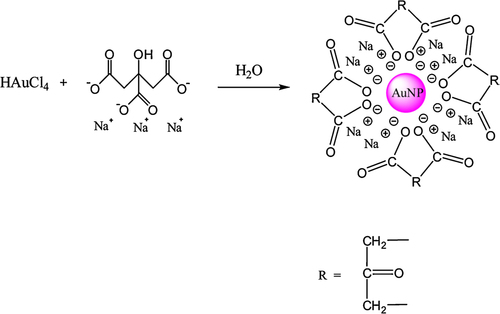
The Turkevich method was modified by Frens, in 1973, to obtain AuNPs with diameters ranging from 15 to 150 nm, by controlling the ratio of reducing agent/stabilizing agent (trisodium citrate/gold). The Turkevich-Frens method has been further modified by several research groups (CitationFrens 1973).
Kimling et al. demonstrated that a high citrate concentration more quickly stabilizes AuNPs of smaller sizes, while a small concentration of citrate will lead to the aggregation of the small particles into larger particles (CitationZhao et al. 2013, CitationKimling et al. 2006).
Recently, the important role of sodium citrate on the pH of the solution, and its role in controlling the size of the nanoparticle, were indicated based on a theoretical model and experimental results (CitationLi et al. 2011, CitationJi et al. 2007, CitationYang et al. 2007, CitationKumar et al. 2007).
Puntes et al. have reported that the addition of reagents in the inverse sequence (addition of HAuCl4 into a boiling sodium citrate solution) leads to the production of AuNPs with small size and a narrow size distribution () (CitationOjea-Jiménez et al. 2011).
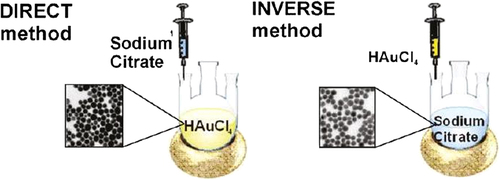
A considerable amount of literature has been published on the effect of temperature (CitationLink and El-Sayed 1999), pH (CitationPatungwasa and Hadak 2008), citrate concentration (CitationVolkert et al. 2011), and gold chloride concentrations (CitationZabetakis et al. 2012), on the characteristic properties of AuNPs synthesized using citrate as reductant.
The Brust-Schiffrin method
The Brust-Schiffrin method was discovered by Brust and Schiffrin in 1994. This method allowed an easy approach to the synthesis of thermally stable and air-stable AuNPs of controlled size and low dispersity. In this technique, AuCl4- was transferred to a toluene phase from an aqueous solution using tetraoctylammonium bromide (TOAB) as the phase-transfer agent, and reduced by NaBH4, in the presence of dodecanethiol. Addition of the reducing agent causes a color change of the organic phase, from orange to deep brown. This clearly indicates the formation of AuNPs (CitationBrust et al. 1994).
Electrochemical method
The electrochemical production of nanoparticles was first studied by Reetz et al., in 1994 (CitationReetz and Helbig 1994, CitationReetz et al. 1995). Their studies showed that size- selective nano scale of transition metal particles could be set electrochemically, using tetra alkyl ammonium salts as stabilizers of metal clusters in a nonaqueous medium.
Gold nanoparticles on the surface of multi-walled carbon nanotubes with glassy carbon electrodes can be prepared using the electrochemical synthesis technique (CitationSong et al. 2013).
The gold nanoparticles were prepared electrochemically using a simple two-electrode cell, with oxidation of the anode and reduction of the cathode. schematically depicts the electrochemical apparatus (CitationHuang et al. 2006).
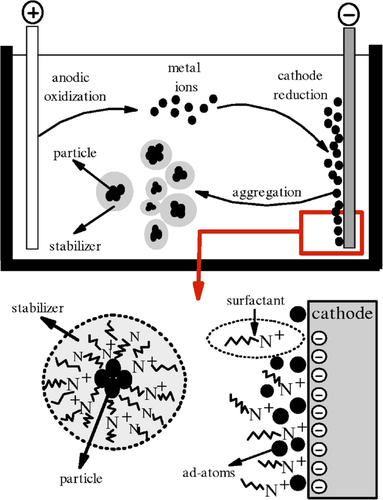
The electrochemical process has been verified to be superior to other methods of nanoparticle production, due to its modest equipment, low cost, lower processing temperature, high quality, and ease of controlling the yield (CitationFreeman et al. 1995, CitationChen and Yang 2002, CitationHaruta and Daté 2001, CitationKuge et al. 2000, CitationKamat et al. 1998).
Seeding growth method
Another method that has also been reported for the synthesis of gold nanoparticles is the seeding growth method. According to the seeding growth process, gold nanoparticles of diameters 5−40 nm and a narrow size distribution were synthesized. Particle size can be controlled by the changeable ratio of seed to metal salt, and therefore every size in the range 5−40 nm can be prepared (CitationJana et al. 2001). This method has the advantage of being a simple, quick, and low cost process; while trisodium citrate was used as a source of OH− ions in the seeding step, sodium borohydrate (NaBH4) was used as a reducing agent (CitationSiti et al. 2013) ().
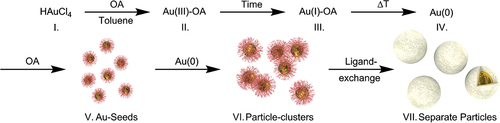
Biological method
Although chemical methods are the most common approach for the synthesis of metallic nanoparticles, the use of expensive and toxic reagents as reducing and stabilizing agents limits their applications. In addition, these nanoparticles may have harmful effects in biomedical applications (CitationNoruzi et al. 2011, CitationShankar et al. 2004). Hence, there is a growing need to develop eco-friendly and cost-effective procedures for the synthesis of nanoparticles that do not use any toxic chemicals. Biological synthesis of nanoparticles has been at the center of attention as a green and eco-friendly method in current years. In biological methods, nanoparticles are synthesized by microorganisms, enzymes, and plants or plant extracts (CitationMohanpuria et al. 2008, CitationSingh et al. 2013).
Recently, the use of plants for the synthesis of nanoparticles is gaining importance, because of their availability, low cost, eco-friendliness and non-toxic nature. In recent years, the biosynthesis of AuNPs using plants such as Azadirachta indica (CitationShankar et al. 2004), Medicago sativa (CitationGardea-Torresdey et al. 2002), Aloe vera (CitationChandran et al. 2006), Cinnamomum camphora (CitationHuang et al. 2007), Pelargonium graveolens (CitationShankar et al. 2004), Coriandrum sativum (CitationNarayanan and Sakthivel 2008), Terminalia catappa (CitationAnkamwar 2010), and lemongrass (CitationShankar et al. 2004), have been reported (CitationSmithaa et al. 2009, CitationParida et al. 2011).
Many papers have been published, reporting the synthesis of AuNPs using plant extracts (CitationVadlapudi and Kaladhar 2014), such as Memecylon umbellatum (CitationArunachalam et al. 2013), Macrotyloma uniflorum (CitationAromal et al. 2012), Brevibacterium casei (CitationKalishwaralal et al. 2010, CitationMittal et al. 2013), Citrus limon, Citrus reticulata and Citrus sinensis (CitationSujitha and Kannan 2013), Piper pedicellatum (CitationTamuly et al. 2013), Terminalia chebula (CitationKumar et al. 2012), Memecylon edule (CitationElavazhagan and Arunachalam 2011), Nyctanthes arbor-tristis (CitationDas et al. 2011), Murraya koenigii (CitationPhilip et al. 2011), Mangifera indica (CitationPhilip 2010), Banana peel (CitationBankar et al. 2010), Cinnamomum zeylanicum (CitationSmitha et al. 2009), and Cochlospermum gossypium (CitationVinod et al. 2011).
Kumar et al. synthesized AuNPs, with a particle size in the range of 5–15 nm, using the extract of Zingiber officinale, which plays a double role as a reducing and stabilizing agent (CitationKumar et al. 2011).
Umesh Kumar et al. reported the green synthesis of gold nanoparticles using onion (Allium cepa) extract as the reducing agent. The reduction of AuNPs occurs due to the activity of vitamin C in onion extract (CitationParida et al. 2011).
Ionic liquids and gold nanoparticles
In recent years, ionic liquids (ILs) have been discovered to be remarkable and unique media for the synthesis and stabilization of metal nanoparticles (CitationRichter et al. 2013, CitationDupont et al. 2002, CitationMudring et al. 2009, CitationJacob et al. 2006).
ILs are salts with low melting points, which are becoming progressively more important as solvents because of their unique properties such as nonvolatility, thermal stability, and designable miscibility with cosolvents (CitationKumar et al. 2007, CitationWelton 1999, CitationGao et al. 2008).
ILs can act not only as the solvents, but can also serve as capping agents (CitationMu et al. 2005), templates (CitationFechler et al. 2013), and even precursors of the materials (CitationParaknowitsch et al. 2010, CitationZhang et al. 2014).
ILs have been broadly used in a variety of chemical reactions (CitationCassol et al. 2005, CitationLeitner 2003), separations (CitationArce et al. 2007), and electrochemical applications (CitationGao et al. 2008, CitationEnders Dickinson et al. 1999).
Synthesis of gold nanoparticles in ionic liquids
Kim et al. described a one-phase synthesis of AuNPs using thiol-functionalized ILs. Thiol-functionalized ILs acted as the stabilizing agents for the synthesis of gold nanoparticles. They reported that the size and stability of the nanoparticles were affected by the number and position of thiol groups in the IL (CitationKim et al. 2004).
In 2006, Kim et al. reported an easy one-pot method of synthesis of AuNPs using N-(2-hydroxyethyl)-N-methylmorpholinium tetrafluoroborate. The alcohol ionic liquids function both as the reducing agent and as a stabilizer, thus considerably simplifying the procedure of nanoparticle preparation (CitationKim et al. 2006).
Stable gold nanoparticles were synthesized in quaternary ammonium ionic liquids (QAILs) by simple heating. The QAILs can not only be used in the role of the reducing agent because of the reactivity of their hydroxyl groups, but can also function as the stabilizing agent due to their chelating ability. The molecular structures of QAILs and stabilized gold nanoparticles are shown in (CitationHuang et al. 2011).
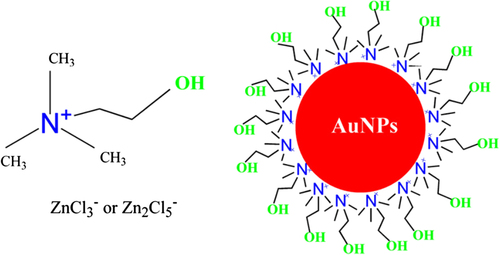
Synthesis of gold nanoparticles modified with ionic liquid
Itoh et al. have demonstrated the synthesis of GNPs modified with IL, based on the imidazolium cation (). They found the application of the aggregation-induced color changes of the gold nanoparticles in aqueous solutions as an optical sensor for anions, via anion exchange of the ionic liquid moiety (CitationItoh et al. 2004).
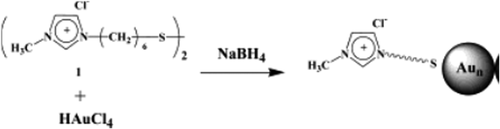
The sonochemical method is a unique technique for the synthesis of metal nanoparticles. The benefits of using this method for the synthesis of metal nanoparticles include the capability to form very small metal nanoparticles, and a rapid reaction rate (CitationPark et al. 2006).
However, metal nanoparticles synthesized by sonochemical reduction usually have wide size distributions. In order to eliminate these difficulties, surfactants and alcohols are generally used in the sonochemical method, to control the particle size and shape (CitationCao et al. 1995, CitationFujimoto et al. 2001, CitationOkitsu et al. 1996, CitationYeung et al. 1993, CitationNagata et al. 1996).
The sonochemical reduction of Au(III), in the presence of an organic additive, occurs in the following steps (CitationOkitsu et al. 2005, CitationYeung et al. 1993, CitationNagata et al. 1996, CitationOkitsu et al. 2002, CitationCaruso et al. 2002).
Okitsu, Kenji et al. proposed a method to synthesize gold nanoparticles deposited on chitosan powder using the sonochemical method. The average size of the prepared AuNPs was measured to be 22 nm (CitationOkitsu et al. 2007).
Highly stable AuNPs, with small particle size and uniform distribution, were successfully synthesized using the sonochemical method in thiol-functionalized ILs (CitationEbrahimnezhad et al. 2013) ().
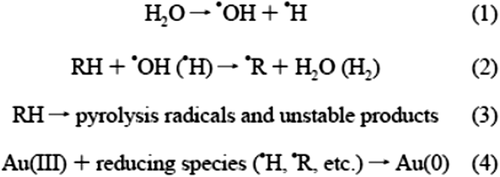
Conclusion
As we have seen, the synthesis of AuNPs is an important area of research in nanotechnology. Generally there are two approaches for the synthesis of AuNPs: the “bottom-up” approach and the “top-down” approach (CitationEustis and El-Sayed 2006). The bottom-up approach consists of nanosphere lithography, templating, chemical, photochemical, electrochemical, sonochemical, and thermal reduction techniques (CitationHaes et al. 2004, CitationPileni 1997, CitationOkitsu et al. 2005, CitationHall et al. 2001, CitationMagnusson et al. 1999).
Authors’ contributions
AA conceived of the study and participated in its design and coordination. RH, and EA participated in the sequence alignment and drafted the manuscript. All authors read and approved the final manuscript.
Acknowledgments
The authors thank the Department of Medical Nanotechnology, Faculty of Advanced Medical Science of Tabriz University, for all support provided . This work is funded by a 2014 grant by the Drug Applied Research Center, Tabriz University of Medical Sciences.
Declaration of interest
The authors have no declaration of interest. The authors alone are responsible for the content and writing of the paper.
References
- Ankamwar B. 2010. Biosynthesis of gold nanoparticles (green-gold) using leaf extract of Terminalia catappa. J Chem. 7:1334–1339
- Arce A, Earle MJ, Rodríguez H, Seddon KR. 2007. Separation of benzene and hexane by solvent extraction with 1-alkyl-3- methylimidazolium bis {(trifluoromethyl) sulfonyl} amide ionic liquids: effect of the alkyl-substituent length. J Phys Chem B. 111: 4732–4736.
- Aromal SA, Vidhu VK, Philip D. 2012. Green synthesis of well-dispersed gold nanoparticles using Macrotyloma uniflorum. Spectrochim Acta A Mol Biomol Spectrosc. 85:99–104
- Arunachalam KD, Annamalai SK, Hari S. 2013. One-step green synthesis and characterization of leaf extract-mediated biocompatible silver and goldnanoparticles from Memecylon umbellatum. Int J Nanomedicine. 8:307–1315
- Bankar A, Joshi B, Kumar AR, Zinjarde S. 2010. Banana peel extract mediated synthesis of gold nanoparticles. Colloids and Surfaces B: Biointerfaces. 80:45–50.
- Brust M, Walker M, Bethell D, Schiffrin DJ, Whyman RJ. 1994. Synthesis of thiol-derivatised gold nanoparticles in a two-phase liquid–liquid system. Chem Soc Chem Commun. 7:801–802
- Cai W, Chen X. 2007. Nanoplatforms for targeted molecular imaging in living subjects. Small. 3:1840–1854.
- Cao X, Koltypin Y, Kataby G, Prozorov R, Gedanken A. 1995. Controlling the particle size of amorphous iron nanoparticles. J Mater Res. 10:2952–2957.
- Caruso RA, Ashokkumar M, Grieser F. 2002. Sonochemical formation of gold sols. Langmuir. 18:7831–7836.
- Cassol CC, Umpierre AP, Machado G, Wolke SI, Dupont J. 2005. The role of Pd nanoparticles in ionic liquid in the Heck reaction. J Am Chem Soc. 127:3298–3299.
- Chandran SP, Chaudhary M, Pasricha R, Ahmad A, Sastry M. 2006. Synthesis of gold nanotriangles and silver nanoparticles using Aloevera plant extract. Biotechnol Progress. 22:577–583
- Chen S, Yang Y. 2002. Magnetoelectrochemistry of gold nanoparticle quantized capacitance charging. J Am Chem Soc. 124:5280–5281
- Daniel MC, Astruc D. 2004. Gold nanoparticles: assembly, supramolecular chemistry, quantum-size-related properties, and applications toward biology, catalysis, and nanotechnology. Chem Rev. 104:293–346.
- Das RK, Gogoi N, Bora U. 2011. Green synthesis of gold nanoparticles using Nyctanthes arbortristis flower extract. Bioprocess Biosyst Eng. 34:615–619.
- Dupont J, Fonseca GS, Umpierre AP, Fichtner PFP, Teixeira SR. 2002. Transition-metal nanoparticles in imidazolium ionic liquids: recycable catalysts for biphasic hydrogenation reactions. J Am Chem Soc. 124:4228–4229.
- Ebrahimnezhad Z, Zarghami N, Keyhani M, Amirsaadat S, Akbarzadeh A, Rahmati M, et al. 2013. Inhibition of hTERT gene expression by silibinin-loaded PLGA-PEG-Fe3O4 in T47D breast cancer cell line. BioImpacts. 3:67–74.
- Elavazhagan T, Arunachalam KD. 2011. Memecylon edule leaf extract mediated green synthesis of silver and gold nanoparticles. Int J Nanomedicine. 6:1265–1278.
- Enders Dickinson V, Williams ME, Hendrickson SM, Masui H, Murray RW. 1999. Hybrid redox polyether melts based on polyether-tailed counterions. J Am Chem Soc. 121:613–616.
- Eustis S, El-Sayed MA. 2006. Why gold nanoparticles are more precious than pretty gold: noble metal surface plasmon resonance and its enhancement of the radiative and nonradiative properties of nanocrystals of different shapes. Chem Soc Rev. 35:209–217.
- Fechler N, Fellinger T-P, Antonietti M. 2013. Salt templating”: a simple and sustainable pathway toward highly porous functional carbons from ionic liquids. Adv Mater. 25:75–79.
- Freeman RG, Grabar KC, Allison KJ, Bright RM, Davis JA, Guthrie AP, et al. 1995. Self-assembled metal colloid monolayers: an approach to SERS substrates. Science. 267:1629–1632
- Frens G. 1973. Controlled nucleation for the regulation of the particle size in monodisperse gold suspensions. Nature. 241:20–22.
- Fujimoto T, Terauchi S, Umehara H, Kojima I, Henderson W. 2001. Sonochemical preparation of single-dispersion metal nanoparticles from metal salts. Chem Mater. 13:1057–1060.
- Gao Y, Voigt A, Zhou M, Sundmacher K. 2008. Synthesis of single-crystal gold nano-and microprisms using a solvent-reductant- template ionic liquid. Eur J Inorg Chem. 24:3769–3775.
- Gardea-Torresdey JL, Parsons JG, Gomez E, Peralta-Videa J, Troiani HE, Santiago P, Jose Yacaman M. 2002. Formation and growth of Au nanoparticles inside live alfalfa plants. Nano Lett. 2:397–401
- Geethalakshmi R, Sarada DVL. 2012. Gold and silver nanoparticles from Trianthema decandra: synthesis, characterization, and antimicrobial properties. Int J Nanomed. 7:5375–5384.
- Ghosh P, Han G, De M, Kim CK, Rotello VM. 2008. Gold nanoparticles in delivery applications. Adv Drug Deliv Rev. 60:1307–1315.
- Haes AJ, Hall WP, Chang L, Klein WL, Van Duyne RP. 2004. A localized surface plasmon resonance biosensor: first steps toward an assay for Alzheimer's disease. Nano Lett. 4:1029–1034.
- Hall SR, Shenton W, Engelhardt H, Mann S. 2001. Site-specific organization of gold nanoparticles by biomolecular templating. Chem Phys Chem. 2:141–191
- Haruta M, Daté M. 2001. Advances in the catalysis of Au nanoparticles. Appl Catal A General. 222:427–437.
- Hu M, Chen J, Li ZY, Au L, Hartland GV, Li X, et al. 2006. Gold nanostructures: engineering their plasmonic properties for biomedical applications. Chem Soc Rev. 35:1084–1094.
- Huang CJ, Chiu PH, Wang YH, Chen KL, Linn JJ, Yang CF. 2006. Electrochemically controlling the size of gold nanoparticles. Je Electrochem Soc. 153:D193–D198
- Huang J, Li Q, Sun D, Lu Y, Su Y, Yang X, et al. 2007. Biosynthesis of silver and gold nanoparticles by novel sundried Cinnamomum camphora leaf. Nanotechnology. 18:105104
- Huang W, Chen S, Liu Y, Fu H, Wu G. 2011. The controlled synthesis of stable gold nanoparticles in quaternary ammonium ionic liquids by simple heating. Nanotechnology. 22:025602.
- Itoh H, Naka K, Chujo Y. 2004. Synthesis of gold nanoparticles modified with inic liquid based on the imidazolium cation. J Am Chem Soc. 126:3026–3027.
- Jacob DS, Bitton L, Grinblat J, Felner I, Koltypin Y, Gedanken A. 2006. Are ionic liquids really a boon for the synthesis of inorganic materials? A general method for the fabrication of nanosized metal fluorides. Chem Mater. 18:3162–3168.
- Jain PK, Lee KS, El-Sayed IH, El-Sayed MA. 2006. Calculated absorption and scattering properties of gold nanoparticles of different size, shape, and composition: applications in biological imaging and biomedicine. J Phys Chem B. 110:7238–7248.
- Jana NR, Gearheart L, Murphy CJ. 2001. Seeding growth for size control of 5–40 nm diameter gold nanoparticles. Langmuir. 17:6782–6786
- Ji XH, Song XN, Li J, Bai YB, Yang WS, Peng XG. 2007. Size control of gold nanocrystals in citrate reduction: the third role of citrate. J Am Chem Soc. 129:13939
- Kalishwaralal K, Deepak VV, Ram Kumar Pandian S, Kottaisamy M, Barathmani Kanth S, Kartikeyan B, Gurunathan S. 2010. Biosynthesis of silver and gold nanoparticles using Brevibacterium casei. Colloids Surf B Biointerfaces. 77:257–262
- Kamat PV, Flumiani M, Hartland GV. 1998. Picosecond dynamics of silver nanoclusters. Photoejection of electrons and fragmentation. J Phys Chem B. 102:3123–3128
- Kim KS, Choi S, Cha JH, Yeon SH, Lee H. 2006. Facile one-pot synthesis of gold nanoparticles using alcohol ionic liquids. J Mater Chem. 16:1315–1317.
- Kim KS, Demberelnyamba D, Lee H. 2004. Size-selective synthesis of gold and platinum nanoparticles using novel thiolfunctionalized ionic liquids. Langmuir. 20:556–560.
- Kimling J, Maier M, Okenve B, Kotaidis V, Ballot H, and Plech A. 2006. Turkevich method for gold nanoparticle synthesis revisited. J Phys Chem B. 110:15700–15707
- Kuge K, Arisawa M, Aoki N, Hasegawa A. 2000. Preparation of gelatin layer film with gold clusters in using photographic film. Jpn J Appl Phys. 39:6550.
- Kumar A, Murugesan S, Pushparaj V, Xie J, Soldano C, John G, et al. 2007. Conducting organic–metallic composite submicrometer rods based on Ionic liquids. Small. 3:429–433.
- Kumar KM, Mandal BK, Sinha M, Krishnakumar V. 2012. Terminalia chebula mediated green and rapid synthesis of gold nanoparticles. Spectrochim Acta A Mol Biomol Spectrosc. 86:490–494
- Kumar KP, Paul W, Sharma CP. 2011. Green synthesis of gold nanoparticles with Zingiber officinale extract: characterization and blood compatibility. Proc Biochem. 46:2007–2013.
- Kumar S, Gandhi KS, Kumar R. 2007. Modeling of formation of gold nanoparticles by citrate method. Ind Eng Chem Res. 46:3128
- Leitner W. 2003. Catalysis: A greener solution. Nature. 423:930–931.
- Li C, Li D, Wan G, Xu J, Hou W. 2011. Facile synthesis of concentrated gold nanoparticles with low size-distribution in water: temperature and pH controls. Nanoscale Res Lett. 6:1–10
- Link S, El-Sayed MA. 1999. Size and temperature de- pendence of the plasmon absorption of colloidal gold nanoparticles. J Phys Chem B. 103:4212–4217
- Magnusson MH, Deppert K, Malm JO, Bovin JO and Samuelson L. 1999. Gold nanoparticles: production, reshaping, and thermal charging. J Nano Res. 1:243–251
- Mandal S. 2014. Synthesis of radioactive gold nanoparticle in surfactant medium. J Radioanal Nucl Chem. 299:1209–1212
- Matei A, Cernica I, Cadar O, Roman C, Schiopu V. 2008. Synthesis and characterization of ZnO-polymer nanocomposites. Int J Mater Form. 1:767–770.
- Mittal AK, Chisti Y, Banerjee UC. 2013. Synthesis of metallic nanoparticles using plant extracts. Biotechnol Adv. 31:346–356
- Mody VV, Siwale R, Singh A, Mody HR. 2010. Introduction to metallic nanoparticles. J Pharm Bioall Sci. 2:282–9
- Mohanpuria P, Rana NK, Yadav SK. 2008. Biosynthesis of nanoparticles: technological concepts and future applications. J Nanopart Res. 10:507–517.
- Mu X-D, Meng J-Q, Li Z-C, Kou Y. 2005. Rhodium nanoparticles stabilized by ionic copolymers in ionic liquids: long lifetime nanocluster catalysts for benzene hydrogenation. J Am Chem Soc. 127:9694–9695.
- Mudring A-V, Alammar T, Backer T, Richter K. 2009. Nanoparticle synthesis in ionic liquids. In: ACS Symposium Series, Vol. 1030. Oxford University Press, pp. 177–188.
- Nagata Y, Mizukoshi Y, Okitsu K, Maeda Y. 1996. Sonochemical formation of gold particles in aqueous solution. Radiat. Res. 146:333–338.
- Nakanishi M, Takatani H, Kobayashi Y, Hori F, Taniguchi R, Iwase A, and Oshima R. 2005. Characterization of binary gold/platinum nanoparticles prepared by sonochemistry technique. Appl Surf Sci. 241:209–212.
- Narayanan K, Sakthivel N. 2008. Coriander leaf mediated biosynthesis of gold nanoparticles. Mater Lett. 62:4588–4590
- Noruzi M, Zare D, Khoshnevisan K, Davoodi D. 2011. Rapid green synthesis of gold nanoparticles using Rosa hybrida petal extract at room temperature. Spectrochim Acta A Mol Biomol Spectrosc. 79:1461–1465
- Ojea-Jiménez I, Bastús NG, Puntes V. 2011. Influence of the sequence of the reagents addition in the citrate-mediated synthesis of gold nanoparticles. J Phys Chem C. 115:15752–15757
- Okitsu K, Ashokkumar M, Grieser F. 2005. Sonochemical synthesis of gold nanoparticles: effects of ultrasound frequency. J Phys Chem B. 109:20673–20675.
- Okitsu K, Mizukoshi Y, Bandow H, Maeda Y, Yamamoto T, Nagata Y. 1996. Formation of noble metal particles by ultrasonic irradiation. Ultrason Sonochem. 3:S249–S251.
- Okitsu K, Mizukoshi Y, Yamamoto TA, Maeda Y, Nagata Y. 2007. Sonochemical synthesis of gold nanoparticles on chitosan. Mater Lett. 61:3429–3431.
- Okitsu K, Yue A, Tanabe S, Matsumoto H, Yobiko Y, Yoo Y. 2002. Sonolytic control of rate of gold (III) reduction and size of formed gold nanoparticles: relation between reduction rates and sizes of formed nanoparticles. Bull Chem Soc Jpn. 75:2289–2296.
- Paciotti GF, Kingston DGI, Tamarkin L. 2006. Colloidal gold nanoparticles: a novel nanoparticle platform for developing multifunctional tumor-targeted drug delivery vectors. Drug Dev Res. 67:47–54.
- Paciotti GF, Myer L, Weinreich D, Goia D, Pavel N, McLaughlin RE, Tamarkin L. 2004. Colloidal gold: a novel nanoparticle vector for tumor directed drug delivery. Drug Deliv. 11:169–183.
- Pal R, Panigrahi S, Bhattacharyya D, Chakraborti AS. 2013. Characterization of citrate capped gold nanoparticle-quercetin complex: experimental and quantum chemical approach. J Mol Struct. 1046:153–163.
- Paraknowitsch JP, Zhang J, Su D, Thomas A, Antonietti M. 2010. Ionic liquids as precursors for nitrogen-doped graphitic carbon. Adv Mater. 22:87–92.
- Parida UK, Bindhani BK, Nayak P. 2011. Green synthesis and characterization of gold nanoparticles using onion (Allium cepa) extract. World J Nano Sci Eng. 1:93–98
- Park J-E, Atobe M, Fuchigami T. 2006. Synthesis of multiple shapes of gold nanoparticles with controlled sizes in aqueous solution using ultrasound. Ultrason Sonochem. 13:237–241.
- Patungwasa W, Hadak JH. 2008. pH tunable morphology of the gold nanoparticles produced by citrate re- duction. Mater Chem Phys. 108:45–54
- Philip D, Unni C, Aromal SA, Vidhu VK. 2011. Murraya Koenigii leaf-assisted rapid green synthesis of silver and gold nanoparticles. Spectrochim Acta A Mol Biomol Spectrosc. 78:899–904.
- Philip D. 2010. Rapid green synthesis of spherical gold nanoparticles using Mangifera indica leaf. Spectrochim Acta A Mol Biomol Spectrosc. 77:807–810.
- Pileni MP. 1997. Nanosized particles made in colloidal assemblies. Langmuir. 13:3266–3276.
- Porta F, Rossi M. 2003. Gold nanostructured materials for the selective liquid phase catalytic oxidation. J Mol Catal A Chem. 204:553–559.
- Reetz MT, Helbig W, Quaiser SA, Stimming U, Breuer N, Vogel R. 1995. Visualization of surfactants on nanostructured palladium clusters by a combination of STM and high-resolution TEM. Science. 267:367–369.
- Reetz MT, Helbig W. 1994. Size-selective synthesis of nanostructured transition metal clusters. J Am Chem Soc. 116:7401–7402.
- Richter K, Campbell PS, Baecker T, Schimitzek A, Yaprak D, Mudring A-V. 2013. Ionic liquids for the synthesis of metal nanoparticles. Phys Status Solidi B. 250:1152–1164.
- Salata OV. 2004. Applications of nanoparticles in biology and medicine. J Nanobiotechnol. 2:3.
- Schaal PA, Besmehn A, Maynicke E, Noyong M, Beschoten B, Simon U. 2012. Electrically conducting nanopatterns formed by chemical e-beam lithography via gold nanoparticle seeds. Langmuir. 28:2448–2454
- Shah M, Badwaik V, Kherde Y, Waghwani HK, Modi T, Aguilar ZP, et al. 2014. Gold nanoparticles: various methods of synthesis and antibacterial applications. Front Biosci. 19:1320–1344
- Shankar SS, Rai A, Ahmad A, Sastry M. 2004. Rapid synthesis of Au, Ag, and bimetallic Au core–Ag shell nanoparticles using Neem (Azadirachta indica) leaf broth. J Colloid Interface Sci. 275:496–502.
- Shankar SS, Rai A, Ankamwar B, Singh A, Ahmad A, Sastry M. 2004. Biological synthesis of triangular gold nanoprisms. Nat Mater. 3:482–488.
- Shankar SS, Rai A, Ankamwar B, Singh A, Ahmad A, Sastry M. 2004. Biological synthesis of triangular gold nanoprisms. Nat Mater. 3:482–488
- Singh M, Kalaivani R, Manikandan S, Sangeetha N, Kumaraguru AK. 2013. Facile green synthesis of variable metallic gold nanoparticle using Padina gymnospora, a brown marine macroalga. Appl Nanosci. 3:145–151
- Siti RM, Khairunisak AR, Azlan AZ, Rahmah N. 2013. Green synthesis of 10 nm gold nanoparticles via seeded-growth method and its conjugation properties on lateral flow immunoassay. Adv Mater Res. 686:8–12
- Smitha SL, Philip D, Gopchandran KG. 2009. Green synthesis of gold nanoparticles using Cinnamomum zeylanicum leaf broth. Spectrochim Acta A Mol Biomol Spectrosc. 74:735–739.
- Smithaa SL, Philip D, Gopchandrana KG. 2009. Green synthesis of gold nanoparticles using Cinnamomum zeylanicum leaf broth. Spectrochim Acta A. 74:735–739
- Song YZ, Li X, Song Y, Cheng ZP, Zhong H, Xu JM, et al. 2013. Electrochemical synthesis of gold nanoparticles on the surface of multi-walled carbon nanotubes with glassy carbon electrode and their application. Russ J Phys Chem A. 87:74–79
- Sujitha MV, Kannan S. 2013. Green synthesis of gold nanoparticles using Citrus fruits Citrus limon, Citrus reticulata and Citrus sinensis aqueous extract and its characterization, Spectrochim Acta A Mol Biomol Spectrosc. 102:15–23
- Sun S, Mendes P, Critchley K, Diegoli S, Hanwell M, Evans SD, et al. 2006. Fabrication of gold micro-and nanostructures by photolithographic exposure of thiol-stabilized gold nanoparticles. Nano Lett. 6:345–350.
- Tamuly C, Hazarika M, Borah SCh, Das MR, Boruah MP. 2013. In situ biosynthesis of Ag, Au and bimetallic nanoparticles using Piper pedicellatum C.D.C., greenchemistry approach. Colloids Surf B Biointerfaces. 1:627–634
- Thakkar KN., Mhatre SS, and Parikh RY. 2010. Biological synthesis of metallic nanoparticles. Nanomedicine. 6:257–262.
- Tiwari PM, Vig K, Dennis VA, Singh SR. 2011. Functionalized gold nanoparticles and their biomedical applications. Nanomaterials. 1:31–63.
- Tomar A, Garg G. 2013. Short review on application of gold nanoparticles. Global J Pharmacol. 7 34–38.
- Turkevich J, Stevenson PC, Hillier J. 1951. Nucleation and growth process in the synthesis of colloidal gold. Discuss Faraday Soc. 11: 55–75
- Vadlapudi V, D. S. V. G. K. Kaladhar. 2014. Review: green synthesis of silver and gold nanoparticles. Middle-East J Sci Res. 19:834–842
- Verma HN, Singh P, Chavan RM. 2014. Gold nanoparticle: synthesis and characterization. Vet World. 7:72–77.
- Vinod VTP, Saravanan P, Sreedhar B, Keerthi Devi D, Sashidhar RB. 2011. A facile synthesis and characterization of Ag, Au and Pt nanoparticles using a natural hydrocolloid gum kondagogu (Cochlospermum gossypium). Colloids Surf B Biointerfaces. 83:291–298.
- Volkert AA, Subramaniam V, Haes AJ. 2011. Implications of citrate concentration during the seeded growth synthesis of gold nanoparticles. Chem Commun. 47:478–480
- Welton T. 1999. Room-temperature ionic liquids. Solvents for synthesis and catalysis. Chem Rev. 99:2071–2084.
- Yang SC, Wang YP, Wang QF, Zhang RL, Ding BJ. 2007. UV irradiation induced formation of Au nanoparticles at room temperature: The case of pH values. Colloid Surf A Physicochem Eng Asp. 301:174
- Yeung SA, Hobson R, Biggs S, Grieser F. 1993. Formation of gold sols using ultrasound. J Chem SocChemCommun. 4:378–379.
- Yu YY, Chang SS, Lee CL, Wang CR. 1997. Gold nanorods: electrochemical synthesis and optical properties. J Phys Chem B. 101:6661–6664.
- Zabetakis K, Ghann WE, Kumar S, Daniel M. 2012. Effect of high gold salt concentrations on the size and polydispersity of gold nanoparticles prepared by an extended turkevich-frens method. Gold Bull. 45:203–211
- Zhang P, Wu T, Han B. 2014. Preparation of catalytic materials using ionic liquids as the media and functional components. Adv Mater. [Epub ahead of print].
- Zhao P, Li N, Astruc D. 2013. State of the art in gold nanoparticle synthesis. Coord Chem Rev. 257:638–665.