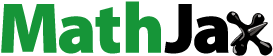
Abstract
In this study, the ability of methotrexate (MTX)-loaded stimuli-responsive novel silica nanocomposites (MSNs) (with mean diameter of ± 60 nm) in the induction of apoptosis, and change in the Bax/Bcl-2 mRNA levels, were investigated. MTT assay and RT -PCR analysis were performed on MDA-MB-231 breast cancer cells, to evaluate their anti-proliferative, apoptotic and anti-apoptotic effects. MTX-loaded MSNs caused marked decrease in the percentage of viable cells, with a significant down-regulation in the level of expression of the anti-apoptotic gene (Bcl-2), and up-regulation in the apoptotic gene (Bax). MTX-loaded MSNs increased the efficacy of the chemotherapeutic agents in the inhibition of cell proliferation and induction of apoptosis.
Introduction
Breast cancer is a malignant proliferation of epithelial cells lining the ducts or lobules of the breast. In the year 2010, about 180,000 cases of invasive breast cancer and 40,000 deaths will occur in the United States. Breast cancers are the most common cause of cancer in women (excluding skin cancer), accounting for about one-third of all cancer in women and the second greatest cause of death, after lung cancer (CitationMukerjee et al. 2012). Despite improvement in our understanding of cancer biology, radiotherapy, chemotherapy and conventional surgical procedures, the general survival rate of cancer patients has not improved noticeably in the past two decades (CitationRani et al. 2012). Nowadays, in clinical practice, a variety of different chemotherapeutic agents have been used for treatment of breast cancer, such as tamoxifen and methotrexate, alone, or in combination with adjuvant chemotherapy (CitationBerry et al. 2005). Furthermore, targeted cancer therapy using Trastuzumab (Herceptin), a HER2/nue-specific humanized monoclonal antibody, can be an efficient alternative. The resistance of tumor cells to conventional therapy remains a challenge in the management of breast cancer (CitationHassan et al. 2010). Methotrexate, an analog of folate, has been used for the treatment of breast cancer, with limited efficacy. The folate receptor is mostly responsible for tumor cell internalizing of MTX (CitationGaies et al. 2012). The important reason for choosing MDA-MB-231 breast cancer cells for our study is that these cells are highly resistant to MTX, because of lack expression of the folate carrier (RFC) (CitationWu et al. 2010, CitationWorm et al. 2001). The mechanism for resistance of these cells is defined by the loss of a cell surface receptor or transporter for an agent, alteration by mutation of the specific target of the drug, or specific metabolism of the drug (CitationGottesman 2002). One of the best ways to overcome drug resistance is to understand the molecular mechanisms of chemo resistance and apply nontoxic nanoparticles for efficient drug delivery (CitationHassan et al. 2010). Nanoparticles are used in both passive and active targeting strategies, to increase the concentration of the agents in cancer cells, while preventing toxicity in normal cells. Mesoporous silica nanoparticles (MSNs) have some advantages that make them suitable for drug delivery, including the vast functional surface, a very small size, the controllable release of cargo by specific stimuli signals (PH, temperature), cell specificity, internalization without detectable toxic effects in eukaryotic cells, excellent dispersibility in biological environments, and lower accumulation in liver and kidney due to less interaction with proteins. Chemical bonding of efficient polymers to MSNs by free-radical surface-graft polymerization provides exceptionally favorable, high surface chain protection. Polymer detachment is also prevented, because of the covalent connection of polymer chains to the MSNs (CitationRadu et al. 2004, CitationHuang et al. 2005, CitationLin et al. 2005, CitationRasouli et al. 2014a, Citation2014b).
The aim of this study is to defeat MTX resistance in MDA-MB231 breast cancer cells by loading MTX into the MSNs and targeting the folate receptor (FR). In this study, we evaluate the efficacy of MTX when it is loaded onto MSNs in targeted therapy, in overcoming drug resistance.
Experimental section
Materials
The MDA MB 123 cell lines were purchased from National Cell Bank of Iran, Pasteur Institute. N-Isopropylacrylamide (NIPAAm) (97%, Sigma-Aldrich, Schnelldorf, Germany) was purified by recrystallization in n-hexane, and dried in vacuum. Methacrylic acid (MAA) and N, N-dimethyl aminoethyl methacrylate (DMAEMA) monomers (Sigma-Aldrich Co., Steinem, Germany) were distilled under reduced pressure in a nitrogen atmosphere. Tetraethyl orthosilicate (TEOS), (3-chloropropyl) trimethoxysilane, ammonia solution (37%), absolute methanol, dichloro-methane, 1,4-dioxane, dimethyl sulfoxide (DMSO), and n-hexane, were purchased from Sigma-Aldrich, Steinem, Germany. Trizol (Sigma-Aldrich, Schnelldorf, Germany), Chloroform (Sigma-Aldrich, Schnelldorf, Germany) and cDNA synthesis kit, fermentase ABI master mix, and Benzoyl peroxide (BPO) were provided from Sigma-Aldrich Co., Steinem, Germany. RPMI and FBS were purchased from Invitrogen (Carlsbad, CA). Trypsin, Sorenson buffer, Trypan blue, dimethylsulfoxide (DMSO), MTT, (3-(4,5-dimethylthiazol-2-yl)-2,5-diphe-nyltetrazolium bromide, and other biological reagents were purchased from Invitrogen (Carlsbad, CA).
Synthesis of modified mesoporous silica nanoparticles
We have previously reported the synthesis root and chemical structure of cationic, pH and thermo-sensitive, modified silica nanocomposites (NIPMACIL@MSNs) (CitationSalehi et al. 2014a). Firstly, silica nanoparticles were prepared according to the sol-gel method (CitationSun et al. 2011, Montalti et al. 2010). In a 250 mL round bottom flask, 60 mL (10 mmol) of ammonia solution (37%) and 1.98 g (10 mmol) of water were added to 100 mL of absolute methanol. The solution was stirred vigorously for 5 min. Then, 10.41 g (500 mmol) tetraethoxysilane (TEOS) was added dropwise. The final solution was stirred for three days at ambient temperature. Then, silica nanoparticles were precipitated with n-hexane and separated through twice centrifugation at 14,000 rpm (Sigma 1-15pK, Germany). The silica nanoparticles obtained were freeze dried (Lyotrap Plus, LTE Scientific Ltd., UK), to give a white powder. Then, a novel N,N-dimethyl-aminoethyl-methacrylate-based ionic liquid (cationic-IL) monomer was synthesized with the reaction of N,N-dimethyl-aminoethyl-methacrylate (4 g, 25.091 mmol) and (3-chloropropyl) trimethoxysilane (6.042 g, 25.091 mmol) under an argon atmosphere, and refluxed for three days at 80°C. The suspension was filtered off and the solvent evacuated. By the addition of 150 mL dry dichloromethane, the precipitate was filtered off under argon atmosphere. The product was then separated by distillation under vacuum (1 mbar), and a cationic-IL monomer was obtained with a honey-like consistency at room temperature, with 98% yield. Then, a novel cationic, pH, and thermo-responsive methacrylic-type ionic liquid terpolymer linker for surface modification of MSN was synthesized, with polymerization of NIPAAm, MAA and cationic-IL monomer, in 1,4-dioxane at 70°C, under argon atmosphere, for 48 h, with molar feed ratios of 50, 40 and 10, respectively. After completion of the reaction, the product (which was named NIPMACIL; the abbreviated name of NIPAAm, MAA and cationic-IL monomer) was collected by centrifugation for further use. The copolymer with a honey-like consistency at room temperature was obtained with 95% yield. Finally, cationic, pH, and thermo-sensitive silica nanocomposites (NIPMACIL@MSNs) were obtained, by dissolving 300 mg of stimuli-responsive ionic liquid-based linker (NIPMACIL) as a novel coupling agent, and 1 g nanosilica in DMSO. After the completion of the reaction in three days, with stirring of the mixture at room temperature, the suspension was centrifuged and the mixture was kept under magnetic stirring at room temperature for another 24 h, and the precipitate was washed with deionized water and completely pulverized in a mortar to achieve a fine powder. After washing, the samples were vacuum-dried and stored in desiccators until use. The chemical structure, thermal behavior, morphology, size and charge of the nanocomposite were fully characterized by 1H-NMR, FT-IR, TGA, SEM, and DLS (Salehi et al. 2014).
MTX loading
The NIPMACIL@MSN nanocomposite (100 mg) was ultrasonically dispersed in a predetermined amount of MTX solution with sonication (400 W) by using the probe-type ultrasonic generator (Sonics Vibra cell, Model: VCX 130 PB, Newton, CT) for 3 min. The weight ratios of the composite nanoparticles to the drug were 10/1, 5/1, and 2/1. Then, the MTX@NIPMACIL@MSNs dispersion was left for 2 h to allow the sedimentation of the fine precipitate. For the determination of encapsulation efficiency, the dispersion was centrifuged at 14,000 rpm for 10 min to collect the MTX-loaded composite nanoparticles. The supernatant containing unentrapped MTX was removed, and the remaining pellets in the centrifuge tube were resuspended in sodium hydroxide (0.1 N) (as MTX is highly soluble in 0.1N NaOH) and vortexed thoroughly for 3 min (CitationSamadi et al. 2011, CitationGhanbari et al. 2014). After vortexing 1 mL of the suspension, it was taken in a micropipette and transferred to a test tube. Then, the suspension was further vortexed for 2 min after adding 5 mL of methanol. The absorbance of the resulting solution was measured using the HPLC-UV method. The HPLC system consists of a Waters 2690 separations module equipped with a UV-visible detector, Waters 2487 dual λ absorbance detector (Waters, Milford, MA). Chromatographic separation was performed at room temperature using a C18 μBondapak (250 mm, 4.6 mm, 10 μm, 125A˚ Waters, Ireland) chromatographic column. The mobile phase of 0.05 M disodium hydrogen phosphate/acetonitrile (65/35 v/v) containing 0.5 mL/L triethylamine (pH 3.7) was pumped. The flow-rate and injection volume were 1 mL/min and 20 μL, respectively. The UV detection of MTX was set at 303 nm. The total run time was 8 min, and the retention time for MTX was 3.2 min. MTX solutions of known concentrations (1–100 μg/mL) were used to generate calibration curves.
The encapsulation efficiency was calculated using the formula:
A: Initial amount of MTX and B: Supernatant-free amount of MTX.
The MTX@NIPMACIL@MSNs obtained were applied for subsequent tests of in vitro drug release study and cytotoxicity tests against MDA MB 231 cells.
In vitro drug release
The MTX@NIPMACIL@MSNs (30 mg) were re-dispersed in 3 mL of desired buffer solutions. The dispersions were then transferred into a dialysis bag (cutoff molecular weight 10,000 g/mol). The bags were subsequently immersed in 10 mL buffer solutions with different pH values (i.e. pH 5.5 and 7.4), with stirring at a rate of 100 rpm, for more than 25 days at 37°C. At the required incubation time, the sample was transferred to 10 mL of fresh buffer solution, and the MTX released in the original buffer solution was determined. The detected absorbance of MTX was converted to their concentrations according to the calibration curve of MTX in the same buffer. Then, the relative percentage of the MTX released was calculated as a function of incubation time, based on the amount of the drug existing in the nanoparticles. Samples were measured using HPLC-UV. The amount of drug released from nanocomposites was calculated by the following equation:
A is the amount of drug in the release medium and B is the amount of drug loaded in the nanocomposites.
In vitro cytotoxicity assay
MTT assay was applied to evaluate the cytotoxic effects of MTX, modified MSN, and MTX-loaded modified MSN in the MDA MB 231 human breast adenocarcinoma cell line (CitationSalehi et al. 2013, Citation2014a, Citation2014b, CitationSamadi et al. 2011, CitationGhanbari et al. 2014). The cells were cultured in a RPMI 1640 medium containing 10% fetal bovine serum, 100 IU/mL penicillin, and 100 μg/mL streptomycin, and incubated at 37°C in an atmosphere of 5% CO2. After dissociation with trypsin 0.25%, the cells were seeded into 96-well plates at a density of 2 ˟ 104 cells/well and incubated for 24 h. The medium was aspirated, and various concentrations of modified MSNs, MTX and MTX@NIPMACIL@MSNs (i.e., 0.8, 0.4, 0.2 and 0.1 mg/mL) were respectively added to the cell growth medium. Control cells were included, with an equivalent volume of fresh media. After incubation for 24, 48, and 72 h, microplates were used for MTT assay. The absorbance of each sample was measured at 570 nm, with a background correction at 630 nm.
Real-time RT-PCR analyses
The trizol RNA isolation system was used to isolate RNA according to the protocol. The total RNA was DNase-treated and purified by using the RNAeasy Mini Kit (Qiagen, Valencia, CA). First-strand DNA was synthesized with MMLV reverse transcriptase (fermentase), by adding 2 μg total extracted RNA. One microliter of cDNA from each sample was used for Real Time RT- PCR amplification using the Bio-Rad iCycler instrument, with flow conditions of 95°C, denatured for 10 min, followed by 40 cycles of 35 s at 94°C, 35 s at 55°C, and 25 s at 72°C .
The primers used in this research have been shown in . The results were analyzed by the ddCt method (CitationPfaffl 2002), using REST.
Table I. Sequence of primers.
Results and discussion
The chemical structure of NIPMACIL@MSNs is given in . As shown in , cationic polymer-conjugated silica nanoparticles contain NIPAAm thermo-sensitive and methacrylic acid pH-sensitive moieties. The SEM () and DLS results revealed that these novel nanocomposites have a mean diameter of ± 60 nm.
Figure 2. Scanning electron micrographs of pH and thermo-responsive silica nanocomposites (NIPMACIL@MSNs).
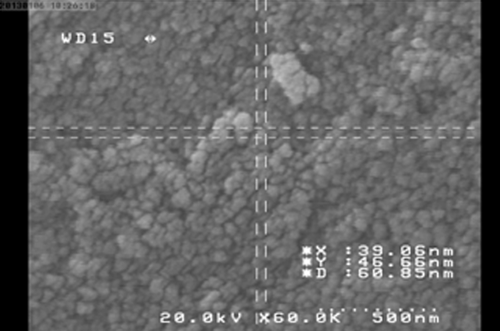
MTX loading and release
In this study, the novel NIPMADM-MSNs nanocomposite () (CitationSalehi et al. 2014a) with special pendant groups, that was able to interact chemically and reversibly with MTX in response to external stimuli, was used . The conjugation of MTX with nanocarriers caused a significant elevation in MTX concentration in cancer cells, because of its potential for ionic interaction with positively and negatively charged components of the carrier and the formation of strong hydrogen bonds. Therefore, large amounts of the MTX molecules (more than 98%) could be attached into the cationic-modified silica nanoparticles (CitationSalehi et al. 2014a).We suggest that MTX can be applied for the construction of a MSN-based drug carrier and then utilize their positive and negative charges to gain control over the adsorption/desorption equilibrium of MTX in an aqueous environment.
The MTX release profile from MTX@NIPMADM-MSNs is represented in . After 1 week, the MTX release results for polymer/drug ratio of (2/1), (5/1) and (10/1) were 50%, 30% and 28% (pH 7.4), and 35%, 29% and 92% (pH 5.5), respectively. In polymer/drug ratios of 2/1 and 5/1, the pH-sensitive release profile disappeared because of surface absorption of MTX, as a result of the lack of adequate interaction between drug and polymer. Therefore, even a large amount of MTX absorbed by the surface of silica can cause burst release. In contrast, at a polymer/drug ratio of 10/1, the release rate was greatly dependent on the pH value. Consequently, a significant release of MTX, with no burst release, was observed at a pH of 5.5, indicating high sensitivity of the drug delivery system to the pH stimulus, which is considered a desirable attribute for a “triggered” formulation to respond to a specific tumoral microenvironment.
NIPMADM-MSNs have two parts: a cationic section, and a PMAA part. These two groups were selected for the carrier because they achieve larger drug loading and pH-dependent drug release. Accordingly, MTX is released at a mild acidic pH, but its release is prohibited considerably at the physiological pH of 7.4. In this way, the normal cells will be protected from the toxic side effects of MTX, and only the cancer cells will be targeted ().
In vitro cell assay
MTX is one of the most effective and commonly used drugs in chemotherapy for cancer, especially breast cancer. The antineoplastic drug methotrexate (MTX) is a folic acid antagonist with several-hundred-fold lower affinity for FR than folic acid (CitationWani et al. 2012, CitationPatr et al. 2005). Because of the lack of folate transporter protein, which is a heavily glycosylated protein and involved in folic acid metabolism, MDA-MB-231 is hereditarily resistant to MTX uptake. Therefore, it was demonstrated that MTX was internalized by MDA-MB-231 cells with low efficiency (CitationWu et al. 2010, CitationWorm et al. 2001). In order to achieve cell-specific drug delivery and improve the efficacy of treatment, there is a need to increase the MTX uptake by the cell lines using an appropriate nanoparticle (CitationRosenholm et al. 2010). Furthermore, the folate receptor has been explored to target therapeutics into cancer cells, due to the over expression of the receptor in cancerous cells. Binding of folic acid to the folate receptors (FR-α and FR-β) initiates receptor-mediated endocytosis and internalization of folic acid (CitationChidambaram et al. 2011). MTT assay was used to study cytotoxic effect of MTX and NIPMACIL@MSN nanocomposites, and MTX@NIPMACIL@MSNs, on MDA-MB-231 cells. As shown in , the NIPMACIL@MSN nanocomposites revealed no cytotoxicity on MDA-MB-231 cells at different concentrations after incubation for 72 h. Conversely, a significant decrease of the viability was observed, when the MDA-MB-231 cells were treated with the suspension of MTX@NIPMACIL@MSNs, in comparison with the drug-free carrier or MTX alone. Similar results were observed when this system was treated with MCF-7 cells (CitationSalehi et al. 2014a). MTX@NIPMACIL@MSNs showed higher anticancer activity after 72 h, in comparison with MTX in free form. Hence, using MTX-loaded nanocomposites, it is possible to overcome the resistance of MDA-MB-231 breast cancer cells to MTX.
Real time PCR apoptosis assay
Evaluation of Bax, Bak and Bcl-2 gene expression by real time RT-PCR
Fluorescence signals were measured over 40 PCR cycles. The cycle number (Ct) at which the signals crossed a threshold set within the logarithmic phase was recorded. For quantization, we evaluated the difference in cycle threshold (ΔCt) between the treated group and the vehicle control for each gene.
It is not clear how normal signaling pathways control apoptosis in the human breast cancer cells. Among the apoptosis regulators, the anti-apoptotic gene Bcl-2 and pro-apoptotic genes Bak and Bax have been mostly associated with ER-negative breast cancer cells (CitationKrajewski et al. 1999). The levels of Bcl-2 increased 3 ± 0.05 times in metastatic cells, and the highest expression was found in MDA-MB-231 cells. The expression of some other members of the Bcl -2 family, Bak and Bax, decreased in the metastatic cell lines (CitationReal et al. 2002). Among different members of the Bcl-2 family of apoptosis regulators, we found that the levels of Bcl-2 were higher in metastatic breast cancer cells. However, the expression of this anti-apoptotic gene can be induced by the estrogen receptor through multiple enhancer elements in breast cancer cells (CitationDong et al. 1999).
MTX was developed in the 1940s as an antagonist of DHFR (CitationCho et al. 2008, CitationFerrari 2005). MTX exerts biological effects by binding to folate receptors which are expressed mainly on cancer cells, increasing apoptosis sensitivity through both death receptors and mitochondrial pathways. Another advantage of using MTX as a chemotherapy reagent is its potential to specifically target cancer cells by the folate receptor.
Also, modified mesoporous silica nanocomposites can induce oxidative stress in a dose-dependent manner. In the cells treated with modified mesoporous silica nanocomposites, lipids and nucleic acids are targeted by induction of ROS (CitationNapierska et al. 2012, CitationAhamed 2013). MTX@NIPMACIL@MSNs have already been used for cancer cell-specific targeting and toxicity (CitationWorm et al. 2001, CitationMontalti et al. 2014).
Submicromolar concentrations of MTX-stimulated apoptosis via death receptors or mitochondrial pathways have synergistic effects on NIPMACIL@MSNs apoptosis induction. In this way, the effects of MTX-loaded modified MSNs (MTX@NIPMACIL@MSNs) and the most effective concentration of MTX@NIPMACIL@MSNs on the Bcl-2 gene expression were investigated. In the present study, the involvement of MTX@NIPMACIL@MSNs on Bcl-2, Bax, and Bak apoptotic gene expression was studied using quantitive Real-Time PCR. After 72 h incubation of MDA-MB-231 with different concentrations of MTX@NIPMACIL@MSNs, the pro-apoptotic gene (Bax) up-regulated, while the anti-apoptotic gene (Bcl-2) down-regulated. Bak has no significant change. Decreased expression of Bcl-2 (anti-apoptotic gene) and increased expression of Bax (pro-apoptotic gene) in response to MTX@NIPMACIL@MSNs can reflect the sum of decrease in the transcriptional level as well as decrease in RNA stability of the cancerous cell ( and ). The overall decrease of the Bcl-2 protein level observed in MDA-MB-231 represents higher levels of Bcl-2 mRNA, that resulted in a decrease in the protein stability that was investigated previously (CitationSun et al. 2011, CitationAkhtar et al. 2010).
Figure 6. qRT-PCR analysis of Bcl-2, synergistic effect of MTX and MTX@NIPMACIL@MSNs, in different concentrations on Bcl-2 expression. Bcl-2 mRNA level was more abundantly down-regulated in cultured cells, in 800 μg/Ml of MTX@NIPMACIL@MSNs (4 mg/ml Silica nano composite and 0.8 mg/ml Methotrexate) concentration shows more Bcl-2 mRNA level down-regulation.
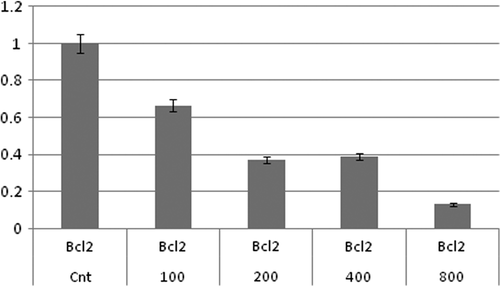
Figure 7. qRT-PCR analysis of Bax, synergistic effect of MTX and MTX@NIPMACIL@MSNs, in different concentrations on Bax expression. Bax mRNA level was more abundantly up regulated in cultured cells, in 800 μg/Ml of MTX@NIPMACIL@MSNs (4 mg/ml Silica nano composite and 0.8 mg/ml Methotrexate) concentration shows more Bax mRNA level up-regulation.
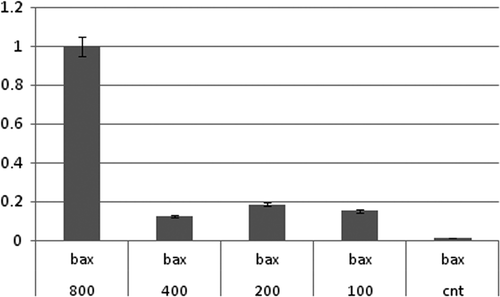
However, our findings showed that the level of Bcl-2 mRNA level is MTX concentration- dependent significant change at the transcription level of Bcl-2 and Bax was more significant in 800 μg/Ml of MTX@NIPMACIL@MSNs (4 mg/ml silica nano composite and 0.8 mg/ml methotrexate).
Down-regulation of Bcl-2 stimulated the production of oxidative stress (CitationAhmad et al. 2011, Citation2012). Oxidative stress causes tissue damage and necrosis. By using the synergistic effect of MTX and MTX@NIPMACIL@MSNs, Bcl-2 was more abundantly down-regulated in cultured cells. Therefore, down regulation of Bcl-2 transcription level has another approach, i.e. production of free radicals and damaging cancerous cells.
Conclusion
In the search for cancer treatment, nanomedicine has been well known for the last two decades as a valuable tool for therapeutic applications. The promotion of nano conjugates for targeting cancer cell receptors and controlled drug delivery systems have shown to increase the therapeutic efficacy and safety of anti-cancer drugs. In summary, a highly effective drug delivery system was synthesized by modification of mesoporous silica nanoparticles. The nanocomposite had good loading capacity for MTX. The cumulative release of MTX@ NIPMACIL@MSNs showed a low leakage at a pH of 7.4, while being significantly enhanced at a pH of 5.5. Faster MTX release under mild acidic conditions is due to the decrease of the electrostatic interactions between drug and matrix. These results demonstrated that the drug release of the nanocomposite is apparently pH-responsive. Cytotoxicity assays on NIPMACIL@MSNs demonstrated no significant cytotoxicity against MDA-MB-231 cells, and hence, it could be used as a safe carrier for anticancer drugs. Furthermore, MTX@NIPMACIL@MSNs showed a remarkably enhanced efficiency in killing MDA-MB-231 cancer cells after 72 h. Evaluation of transcription level by quantitive real time RT-PCR for both the anti-apoptotic gene(Bcl-2) and the pro-apoptotic gene(Bax) demonstrated that Bax/Bcl-2 ratio increased and cell viability of cancerous cells decreased significantly when MTX was applied to modified MSNs.
Declaration of interest
The authors report no declarations of interest. The authors alone are responsible for the content and writing of the paper.
References
- Ahamed M. 2013. Silica nanoparticles-induced cytotoxicity, oxidative stress and apoptosis in cultured A431 and A549 cells. Hum Exp Toxicol. 32:186.
- Ahmad J, Ahmad M, Akhtar MJ, Alrokayan SA, Siddiqui MA, Musarrat J, Al-Khedhairy AA. 2012. Apoptosis induction by silica nanoparticles mediated through reactive oxygen species in human liver cell line HepG2. Toxicol Appl Pharmacol. 259:160–168
- Ahmad M, Akhtar MJ, Siddiqui MA, Ahmad J, Musarrat J, Al-Khedhairy AA, et al. 2011. Oxidative stress mediated apoptosis induced by nickel ferrite nanoparticles in cultured A549 cells. Toxicology. 283:101–108.
- Akhtar MJ, Ahamed M, Kumar S, Siddiqui H, Patil G, Ashquin M, Ahmad I. 2010. Nanotoxicity of pure silica mediated through oxidant generation rather than glutathione depletion in human lung epithelial cells. Toxicology. 276:95–102.
- Berry DA, Cronin KA, Plevritis SK, Fryback DG, Clarke L, Zelen M, et al. 2005. Effect of screening and adjuvant therapy on mortality from breast cancer. N Engl J Med. 353:1784.
- Chidambaram M, Manavalan R, Kathiresan K. 2011. Nanotherapeutics to overcome conventional cancer chemotherapy limitations J Pharm Pharm Sci. 14:67.
- Cho K, Wang X, Nie S, Chen ZG, Shin DM. 2008. Therapeutic nanoparticles for drug delivery in cancer. Clin Cancer Res. 14:1310.
- Dong L, WangWang F, Stoner M, Reed JC, Harigai M, Samudio I, et al. 1999. 26 Mechanisms of transcriptional activation of bcl-2 gene expression by 17beta-estradiol in breast cancer cells. J Biol Chem. 274:32099.
- Ferrari M. 2005. Cancer nanotechnology: opportunities and challenges. Nat Rev Cancer. 5:161–171.
- Gaies E, Jebabli N, Trabelsi S, Salouage I, Charfi R, Lakhal M, Klouz A. 2012. Methotrexate side effects: review article. Drug Metab Toxicol. 3:1000125.
- Ghanbari P, Mohseni M, Tabasinezhad M, Yousefi B, Saei AA, Sharifi S, et al. 2014. Inhibition of survivin restores the sensitivity of breast cancer cells to docetaxel and vinblastine. Appl Biochem Biotechnol. 6:1125.
- Gottesman MM. 2002. Mechanisms of cancer drug resistance. Annu Rev Med. 53:615.
- Hassan MS, Ansari J, Spooner D, Hussain SA. 2010. Chemotherapy for breast cancer (Review). Oncol Rep. 24:1121.
- Huang DM, Hung Y, Ko BS, Hsu SC, Chen WH, Chien CL, et al. 2005. Highly efficient cellular labeling of mesoporous nanoparticles in human mesenchymal stem cells: implication for stem cell tracking. FASEB J. 19:2014
- Krajewski S, Krajewska M, Turner BC, Pratt C, Howard B, Zapata JM, et al. 1999. Prognostic significance of apoptosis regulators in breast cancer. Relat Endocr Cancer. 6:29.
- Lin YS, Tsai CP, Huang HY, Kuo CT, Hung Y, Huang DM, et al. 2005. Effect of the chemical composition on the work function of gold substrates modified by binary self-assembled monolayers. Chem Mater. 17:4570.
- Montalti M, Prodi L, Rampazzo E, Zaccheroni N. 2014. Dye-doped silica nanoparticles as luminescent organized systems for nanomedicine. Chem Soc Rev. 43:4243–4268.
- Mukerjee A, Ranjan AP, Vishwanatha JK. 2012. Combinatorial nanoparticles for cancer diagnosis and therapy. Curr Med Chem. 19:3714.
- Napierska D, Rabolli V, Thomassen LC, Dinsdale D, Princen C, Gonzalez L, et al. 2012.Oxidative stress induced by pure and iron-doped amorphous silica nanoparticles in subtoxicconditions. Chem Res Toxicol. 25:828.
- Patr AK, Kukowska-Latallo JF, Baker JR. 2005. Targeted drug delivery with dendrimers: comparison of the release kinetics of covalently conjugated drug and non-covalent drug inclusion complex. Adv Drug Deliv Rev. 57:2203.
- Pfaffl MW. 2002. Relative expression software tool (REST) for group wise comparison and statistical analysis of relative expression results in real-time PCR. Nucleic Acid Res. 30:e36.
- Radu DR, Lai CY, Jeftinija K, Rowe EW, Jeftinija S, Lin VSY. 2004. A polyamidoaminedendrimer-capped mesoporous silica nanosphere-based gene transfection reagent. Chem Soc.126:13216.
- Rani D, Somasundaram VH, Nair S, Koyakutty M. 2012. Advances in cancer nanomedicine. J Indian Inst Sci. 99:188–218.
- Rasouli S, Davaran S, Rasouli F, Mahkam M, Salehi R. 2014a. Positively charged functionalized silica nanoparticles as nontoxic carriers for triggered anticancer drug release. Des Monomers Polym.17:227.
- Rasouli S, Davaran S, Rasouli F, Mahkam M, Salehi R. 2014b. Synthesis, characterization and pH-controllable methotrexate release from biocompatible polymer/silica nanocomposite for anticancer drug delivery. Drug Deliv. 21:155.
- Real PJ, Sierra A, De Juan A, Segovia JC, Lopez-Vega JM, Fernandez-Luna JL. 2002. Resistance to chemotherapy via Stat3-dependent overexpression of Bcl-2 in metastatic breast cancer cells. Oncogene. 21:7611.
- Rosenholm JM, Peuhu E, Bate-Eya LT, Eriksson JE, Sahlgren C, Lindén M. 2010. Cancer-cell-specific induction of apoptosis using mesoporous silica nanoparticles as drug-delivery vectors. Small. 6:1234.
- Salehi R, Hamishehkar H, Eskandani M, Mahkam M, Davaran S. 2014a. Development of dual responsive nanocomposite for simultaneous delivery of anticancer drugs. J Drug Target. 22:327.
- Salehi R, Irani M, Eskandani M, Nowruzi K, Davaran S, Haririan I. 2014b. Controlled release, and antitumor activity of doxorubicin hydrochloride from pH-sensitive P(NIPAAm-MAA-VP) nanofibrous scaffolds prepared by green electrospinning. Int J Polym Mater Polym BioMater. 63:609–619.
- Salehi R, Irani M, Rashidi MR, Aroujalian A, Raisi A, Eskandani M, et al. 2013. Stimuli-responsive nanofibers prepared from poly(N-isopropylacrylamide-acrylamidevinylpyrrolidone) by electrospinning as an anticancer drug delivery. Des Monomers Polym. 16:515.
- Samadi N, Bekele R, Capatos D, Venkatraman G, Sariahmetoglu M, Brindley DN. 2011. Regulation of lysophosphatidate signaling by autotaxin and lipid phosphate phosphatases with respect to tumor progression, angiogenesis, metastasis and chemo-resistance. Biochemie. 93:61.
- Sun L, Li Y, Liu X, Jin M, Zhang L, Du Z, et al. 2011. Cytotoxicity and mitochondrial damage caused by silica nanoparticles. Toxicol In Vitro 25:1619.
- Wani A, Muthuswamy E, Savithra GH, Mao G, Brock S, Oupický D. 2012. Surface functionalization of mesoporous silica nanoparticles controls loading and release behavior of mitoxantrone. Pharm Res. 29:2407.
- Worm J, Kirkin AF, Dzhandzhugazyan KN, Guldberg P. 2001. Methylation-dependent silencing of the reduced folate carrier gene in inherently methotrexate-resistant human breast cancer cells. J Cells Biol Chem. 276:39990.
- Wu Z, Shah A, Patel N, Yuan X. 2010. Development of methotrexate prolineprodrug to overcome resistance by MDA-MB-231. Bioorg Med Chem Lett. 20:5108.