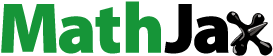
Abstract
The aim of the study was to prepare chemotherapeutic agent-loaded zinc oxide nanoparticles for the intracellular delivery of drug, for better therapeutic activity. Zinc oxide nanoparticles have inherent anticancer properties, hence it was envisaged that by loading the anticancer drug into zinc oxide nanoparticles, enhanced anticancer activity might be observed. Zinc oxide nanoparticles were prepared using zinc nitrate and sodium hydroxide. Starch was used as the stabilizing agent. The nanoparticles prepared were characterized for size, shape, entrapment efficiency, and drug release. Further, cell line studies were performed to evaluate cellular uptake and cytotoxicity profile using MCF-7 cells. A hemolysis study was performed to check the acute toxicity of the nanoparticles. The nanoparticles were found to be 476.4 ± 2.51 nm in size, with low PDI (0.312 ± 0.02) and high entrapment efficiency (> 85%). The nanoparticles were stable, and did not form aggregates on storage in the dispersed form. A cytotoxicity study demonstrated that drug-loaded zinc oxide nanoparticles exhibited higher anticancer activity as compared to either blank zinc oxide nanoparticles and doxorubicin (DOX) alone, or their mixture. A hemolytic test revealed that the prepared zinc oxide nanoparticles caused negligible hemolysis. Thus, it can be concluded that zinc oxide nanoparticles loaded with DOX resulted in better uptake of the chemotherapeutic agent, and at the same time, showed low toxicity towards normal cells.
Introduction
Cancer is a broad term given to a group of diseases all involving the unregulated growth of cells. Cancer originates in a normal cycle of cell division, when a cell can turn cancerous and divide infinitely. With over 10 million new cases per year worldwide, cancer remains a difficult disease to treat, and a significant cause for morbidity and mortality. Conventional anticancer therapies come with a number of drawbacks and side effects. The conventional therapy for cancer includes treatment with cytotoxic drugs (e.g., DOX, paclitaxel, etc.), radiation therapy, and surgical removal of tumors. Cytotoxic drugs cause severe side effects because the mechanisms which they exhibit are common to both cancer cells and normal cells. Thus, tumor cells and normal cells are both killed by anticancer drugs. The bone marrow, gastrointestinal mucosa, hair follicles, and gonads are among the tissues which are most sensitive to chemotherapy. Chemotherapeutic agents are also highly teratogenic (CitationNobili et al. 2006). Development of resistance is another major drawback of chemotherapy. Higher doses are required to kill resistant tumor cells, which further worsens the problem of unwanted side effects.
Nanotechnology provides an alternative strategy to bypass systemic toxicity and multi drug resistance, by offering a means to encapsulate or attach drugs to nanomaterials such as lipids, polymers, and solid-core nanoparticles, which have better targeting ability and are resistant to drug efflux (CitationSun et al. 2008, CitationDavis et al. 2008, CitationVeiseh et al. 2010). Nanoparticles are very small compared to body cells, and thus have high penetrating power and can interact with biomolecules both on the surface of and inside the cells (CitationHong et al. 2011). These interactions can be successfully used for the diagnosis and treatment of cancer. Nanoparticles used in biomedical applications include liposomes, polymeric micelles, dendrimers, inorganic and polymeric nanoparticles, nanorods, and quantum dots. All have been tested pre-clinically or clinically for targeted drug and gene delivery, and also as agents to enhance diagnostic imaging output, for instance, in MRI (CitationGilmore et al. 2008, CitationDeerinck 2008, CitationSon et al. 2007).
Metal nanoparticles exhibit many such physical and chemical properties which are not there in the bulk material, because of their larger surface area and greater area/volume ratio (CitationGaldiero et al. 2011). These altered properties can be lethal to the living cells, making metal nanoparticles useful for the treatment of cancer. Metal nanoparticles also bear some inherent properties which are useful for diagnosis and imaging. Iron oxide, being a superparamagnetic agent, finds its application in imaging techniques like MRI, and is thus a very good diagnostic agent. Iron oxide nanoparticles can target a chemotherapeutic agent to the tumor cells, resulting in targeted drug delivery and imaging at the same time (CitationMaeng et al. 2010, CitationSun et al. 2008, CitationKohler et al. 2006, CitationLai et al. 2010). Gold nanoparticles exhibit a phenomenon known as Surface Plasmon Resonance, which imparts them with both imaging and tumor-killing properties (CitationMukherjee et al. 2005, CitationRamanujan and Kayal 2010, CitationNam et al. 2013, CitationXiao et al. 2012). Besides these inherent properties of metal nanoparticles, they also have the ability to encapsulate, solubilize, or bind a variety of therapeutic agents such as proteins, hydrophobic and hydrophilic drugs, etc. Binding or encapsulation of therapeutic agents to or within the nanoparticles, thus solves the problems of stability, solubility, and many other difficulties which are encountered if the drug is used alone. Imaging agents can also be attached to the nanoparticles, which allow them to be used in diagnosis also (CitationKim et al. 2006, CitationMontet et al. 2006).
Zinc oxide is a promising theranostic (combination of therapy and diagnosis) agent for cancer treatment. Its inherent property of photoluminescence is useful in biosensing applications, and the wide band-gap semiconductor properties are useful in promoting the generation of reactive oxygen species (CitationWang et al. 2009). Several studies have been performed, which have demonstrated and confirmed the potential of zinc oxide nanoparticles in anticancer therapy, as well as in other diseases (CitationNair et al. 2009, CitationAkhtar et al. 2012, CitationLee et al. 2014, CitationPalanikumar et al. 2014, CitationSeker et al. 2014, CitationReddy et al. 2014). The nanoparticles were found to be selectively toxic against cancer cells. CitationAkhtar et al. (2012) investigated the cytotoxicity mechanism of zinc oxide using HepG2 cells. They found that the ZnO nanoparticles induce the activity of the caspase-3 enzyme, DNA fragmentation, generation of reactive oxygen species, and oxidative stress, in the HepG2 cells. CitationZhang et al. (2008) evaluated zinc oxide nanoparticles for photodynamic therapy (PDT) using OVCAR-3 ovarian cancer cells. The results of the study established that nanoparticles generated reactive oxygen species (ROS) in a concentration- and illumination-dependent manner following exposure to ultraviolet A light (365 nm), which gave proof of the concept that photoactivation of ZnO conjugates can cause targeted destruction of tumor cells. Many other such studies have confirmed the therapeutic and diagnostic properties of zinc oxide nanoparticles (CitationHong et al. 2011, CitationHanley et al. 2008, CitationGuo et al. 2008, CitationTaccola et al. 2011).
Therefore, in the present study, it has been hypothesized that by loading the anticancer drug (doxorubicin) into the ZnO nanoparticles, the cytotoxic potential of the drug might be increased, due to the synergism produced by the combination of the anticancer action of the drug as well as that of ZnO.
Materials and methods
Doxorubicin HCl was obtained as a gift sample from Sun Pharma Pvt. Ltd., Baroda, and was checked for its purity. Zinc nitrate, sodium hydroxide, and starch were purchased from CDH analytical agents. Other reagents used were of analytical grade.
Preparation of blank and drug-loaded zinc oxide nanoparticles
Method 1
Zinc oxide nanoparticles were synthesized by a reaction of zinc nitrate and sodium hydroxide, according to the process reported earlier by CitationYadav et al. (2006), with modification. Briefly, solutions of zinc nitrate and sodium hydroxide, of varying concentrations, were prepared separately in water. An aqueous solution of starch of varying concentrations was mixed with the zinc nitrate solution, and the mixture was stirred for 1 h, to ensure complete mixing of zinc nitrate and starch (). Afterwards, sodium hydroxide solution was added drop-wise to this mixture solution, with constant stirring, till the solution attained a pH of 12. Stirring was continued for 2 h after the complete addition of the sodium hydroxide solution. A white precipitate of zinc hydroxide was formed. If an excess of hydroxyl ions (from sodium hydroxide) are added, the precipitate of zinc hydroxide solubilizes due to the conversion of zinc hydroxide into zincate ions (Zn(OH)4+), which are soluble in aqueous medium. The solution mixture was kept overnight to allow settling of the precipitate. The supernatant so obtained was discarded. The white precipitate was separated by centrifugation at 3000 rpm for 5 min. The product was calcined by heating at 80°C. After complete drying, the product was ground into fine powder.
Table I. Size and size distribution of blank ZnO nanoparticles.
Preparation of drug-loaded zinc oxide nanoparticles
For the preparation of drug-loaded nanoparticles, DOX was added to the mixture of zinc nitrate and starch, and stirred for complete solubilization. Four different concentrations of DOX were tried, to assess the optimum concentration, that is, 0.05, 0.08, 0.1, and 0.12 mg/ml. Loading was found to be saturated at 0.1 mg/ml, and hence was selected as the optimum loading concentration. The rest of the steps were followed as described earlier ().
Characterization of blank and drug-loaded nanoparticles
Particle size, size distribution, and zeta potential
The mean particle size and poly dispersity index (PDI) of the prepared nanoparticles were determined by using the particle size analyzer (Delsa Nano C, Beckman Coulter Counter). Briefly, 5 mg of nanoparticles were suspended in 10 ml of distilled water by applying sonication. The suspension was filtered through a syringe filter (1 μm cutoff) to remove large aggregates, and the size of the nanoparticles was determined using the particle size analyzer. The surface morphology of the nanoparticles was observed using scanning electron microscopy (SEM). The sample was coated with gold and then kept in the sampling unit as a thin film, and the photographs were taken at different magnifications using SEM (Jeol, Japan). The zeta potential of the particles is a major factor for stability of the dispersed particles. Hence, the zeta potential of the particles was determined using the Delsa Nano C Zetasizer.
Drug entrapment efficiency of nanoparticles
The entrapment efficiency of ZnO nanoparticles was determined by an indirect method. Briefly, the precipitate of zinc hydroxide was separated by centrifugation at 3000 rpm for 5 min. Drug content in the supernatant was determined by measuring absorbance, using a UV–VIS spectrophotometer (UV 1700 Pharma Spec, Shimadzu, Japan) at 480 nm (λmax of DOX). The amount of drug entrapped was calculated by subtracting the amount of drug in the supernatant from the total amount of drug added. The entrapment efficiency is calculated as follows:
In vitro drug release study of doxorubicin-loaded nanoparticles
The in vitro release study of DOX from nanoparticles was carried out over the period of 24 h, using the dialysis bag method. For this study, 50 mg of nanoparticles were weighed and put in a dialysis membrane-70 (HiMedia, LA393-10MT). This dialysis membrane was taken into 50 ml of phosphate buffer saline (pH 7.4), in a conical flask. This flask was kept in a shaking incubator and the speed of the shaker was maintained at 60 rpm, at 37°C. At specific time intervals, samples (3 ml) were withdrawn. The same volume (3 ml) of the media was replaced after each sampling, to maintain the sink condition during the test. The drug content in the sample was determined using a UV–visible spectrophotometer at 480 nm.
Stability study
The stability of the nanoparticles was checked at room temperature by dispersing 10 mg of nanoparticles in 50 ml of distilled water, by applying sonication. Any settling of the nanoparticles was checked visually, and size distribution of the nanoparticles was measured after 24 h using the particle size analyzer, to check particle aggregation.
In vitro cell line studies
Cell uptake study
Cellular uptake of the formulations by the cancer cells (MCF-7 cells) were evaluated by FACS analysis (CitationNasrolahi Shirazi et al. 2013). The cell culture was freshly prepared by sub culturing the cells using Dulbecco's Minimum Essential Medium (DMEM). After initial passage, the cells (4*104 cells/well) were seeded in 6-well culture plates. After 24 h, the cells were incubated for 1 h and 4 h with free DOX, physical mixture of DOX and ZnO nanoparticles, and DOX-loaded ZnO nanoparticles at a DOX concentration of 10 μg/mL, respectively, in the DMEM media. The media was carefully removed and cells were washed three times with ice-cold PBS, to remove the extra DOX molecules. The cells were trypsinized and suspended in 1 mL PBS, and then centrifuged at 1000 rpm for 5 min. The cell pellet was suspended in PBS (pH 7.4) before analysis, for DOX fluorescence. For gated cell populations, DOX fluorescence was analyzed in a Becton Dickinson FACSCalibur flow cytometer, for 10000 counts per sample aliquot, and the data were recorded accordingly.
Cytotoxicity study (MTT assay)
Cell viability was tested by MTT assay, based on the cleavage of the yellow tetrazolium salt MTT by metabolically active cells to form an orange formazan dye, which was quantified using an enzyme-linked immunosorbent assay (ELISA) reader (CitationNair et al. 2009). The cells were seeded in 96-well microtiter plates (2*104 cells/200 μl growth medium/well), followed by overnight incubation. The supernatants from the wells were aspirated out, and fresh aliquots of growth medium were added. After 24 h, supernatants were aspirated out and the cell monolayers in the wells were washed with 200 μl PBS (0.1 M, pH 7.4). Subsequently, the MTT reagent (150 μl, 0.8 mg/ml) was added in each well and incubated for 5 h. DMSO (100 μl) was added in each well after aspirating out the supernatant, and incubated at 37°C for 1 h. Absorbance values at two wavelengths (570 nm for soluble dye and 630 nm for cells) were recorded using the ELISA reader. Concentrations of samples showing a 50% reduction in cell viability (i.e., IC50 values) were then calculated.
Acute toxicity study: Hemolytic study
A hemolytic study was performed to check if hemolysis is caused by zinc oxide nanoparticles, according to the method followed by CitationBender et al. (2012). Human red blood cells (RBCs) were obtained by centrifuging whole blood from a healthy donor. The plasma and the white layer of leucocytes at the interface were discarded. The erythrocytes were washed several times with 150 mM NaCl until the supernatant was clear, and subsequently re-suspended in 150 mM NaCl, to yield the initial volume. The hemolysis assay was carried out by adding a nanoparticle dispersion to RBCs suspended in the buffer solution. The samples were thoroughly mixed by vortexing, and incubated at 37°C for 1 h under constant shaking. After removal of the cells by centrifugation, hemolysis was determined by measuring the absorbance of the supernatant at 541 nm, reflecting the amount of hemoglobin released into the supernatant. As controls, the same amounts of RBCs were suspended in distilled water and in buffer solution, in order to determine 100% lysis and theoretical 0% lysis, respectively. The percentage of hemolysis by the formulations was calculated, based on theoretical 0% lysis and 100% lysis.
Statistical analysis
All the results are expressed as mean ± standard deviation. The treated groups were compared to the controls by analysis of variance (ANOVA), thanks to the GraphPad PRISM® software (GraphPad Software Corp., San Diego, CA). A p value less than 0.05 was considered as significant.
Results and discussion
The present study was aimed at the loading of the anticancer drug, DOX, into ZnO nanoparticles, to synergize the therapeutic activity of the drug with the anticancer action of ZnO. Drug-loaded nanoparticles were prepared by the wet chemical method using zinc nitrate and sodium hydroxide as precursors. However, when nanoparticles were prepared without using starch, it was found that a major portion of the nanoparticles existed in aggregated form, as also reported earlier by different groups of researchers. Hence, starch was used as a stabilizing agent that will adsorb at the surface of nanoparticles, and thus may provide the stability to the particles. The mechanism by which starch exerts its stabilizing effect is unclear. It could be either due to its property of increasing the viscosity of the solution, or its ability to form a complex with metallic ions through its hydroxyl groups (CitationHe and Zhao 2005). The passive method was used for the loading of drug into the particles. Briefly, DOX in varying amounts was dissolved in one of the solutions of precursors, i.e., zinc nitrate solution, during the reaction, and starch at varying concentrations was also dissolved in the precursor solution. The prepared nanoparticles were optimized on the basis of size and entrapment efficiency.
Optimization and characterization of blank and drug-loaded zinc oxide nanoparticles
Determination and optimization of particle size
The particle size of nanoparticles is one of the most important characteristics, as it has a great effect on the uptake of nanoparticles by the cells. For the targeting of nanoparticles to cancer cells by the Enhanced Permeation and Retention (EPR) effect, the particles must be small enough to penetrate the tumor. Thus, the size of the nanoparticles was optimized by varying the concentrations of starch at equimolar concentrations of zinc nitrate and sodium hydroxide (0.1 M). The stirring speed and time of stirring were kept constant at 4000 rpm and 2 h after complete addition of sodium hydroxide, respectively. The results of the study showed that the minimum concentration of starch required to produce stable particles is 0.5%. Below this concentration, particles were found to aggregate. Starch solution more concentrated than 0.5% could not be prepared because of the insufficient water solubility of starch. Hence, for further studies, 0.5% starch was used for the preparation of nanoparticles. The results of the study were found to be in accordance with the studies performed earlier by researchers.
The concentration of zinc nitrate and sodium hydroxide were also optimized on the basis of particle size and PDI. The result of the study () demonstrated that the least size (412.1 ± 3.23 nm) of the nanoparticles with good PDI (0.325 ± 0.04) was obtained when 0.1 M zinc nitrate solution, 0.1 M sodium hydroxide solution and 0.5% starch solution were used (). After drug loading, the size of the nanoparticles increased from 412.1 ± 3.23 to 476.4 ± 2.51 nm (), which may be ascribed to the passive loading of drug at the surface of the nanoparticles and in the matrix of starch.
Table II. Size, size distribution, and entrapment efficiency of DOX-loaded ZnO nanoparticles.
Determination of zeta potential
The zeta potential of blank zinc oxide nanoparticles was found to be 17.11 mV. The high value of zeta potential indicates the stability of the nanoparticle dispersion. The zeta potential has a positive value because of the fact that zinc oxide nanoparticles have neutral hydroxyl groups chemisorbed onto their surface. At lower pH, protons from the environment get transferred to the particle surface, resulting in a positively charged surface (ZnOH2+). The isoelectric point of ZnO nanoparticles is found to be at a pH range of 9–10. Accordingly, ZnO nanoparticles have a strong positive surface charge under physiological conditions (CitationDegen and Kosec 2000). However, the coating of starch at the surface of the nanoparticles might be a possible reason for the lower zeta potential of the ZnO nanoparticles. Further, the zeta potential of the drug-loaded nanoparticles was found to be a high positive value of 24.67 mV, which confirms the loading of DOX into the nanoparticles. Since DOX is a weakly basic drug and has one ionizable group at physiological pH, this imparts a positive charge to the nanoparticles. Hence, a slight increase in the zeta potential of ZnO nanoparticles was observed after the loading of DOX.
Morphology and drug entrapment efficiency of nanoparticles
The drug entrapment efficiency of nanoparticles was determined at different amounts of drug taken initially. The results of the study () showed that entrapment efficiency was found to be high (more than 80%) at all concentrations of DOX. However, maximum entrapment efficiency was found at the level of 0.1 mg/ml of DOX. On further increasing the amount of DOX, entrapment efficiency was found to be reduced, indicating the saturation of the surface of the nanoparticle with the drug. These results are in accordance with the study reported earlier for the entrapment of amoxicillin in ZnO nanoparticles (CitationPalanikumar et al. 2013). The morphology of the drug-loaded ZnO nanoparticles was examined using SEM. The results of the study () showed that the particles were roughly spherical in shape, which confirms the coating of particles with starch. Further, the size of the particles was also confirmed to be in the nano range (300–500 nm). Some of the nanoparticles were found in aggregated form. However, these nanoparticles de-aggregate on dispersing into water when sonication is applied.
Stability study
The stability of the nanoparticle dispersion was monitored for 24 h. The size of the nanoparticles was determined at different time intervals. The size of the nanoparticles did not change significantly even after 24 h (). This result is supported by the fact that nanoparticles bear a high value of zeta potential, which prevents aggregation and thus confers stability. Thus, it can be concluded that the particles were stable and did not form aggregates for 24 h.
Table III. Size of the nanoparticles after storage.
In vitro drug release from nanoparticles
The release of drug from the nanoparticles, as determined using the dialysis bag method, is shown in . The results of the study demonstrated fast release of drug from the nanoparticles in the initial 5 h, that is, 60% of the total drug was released in 5 h. In contrast, 100% of the drug was released (diffused) from the drug solution in the same period of time. The fast drug release from nanoparticles may be ascribed to the surface-loading (adsorption) of the drug at particle surface. The initial fast release of drug from nanoparticles may be ascribed to the fact that the drug is present (loaded) on the surface of the nanoparticles. Drug molecules absorbed at the surface of the nanoparticles are released faster as compared to embedded drug. However, further drug release was found to be sustained. In 24 h, 95% of the total drug was released, which confirmed the sustained release of the drug from the prepared nanoparticles.
Cytotoxicity studies
The aim of the study was to develop a novel formulation for better anticancer activity and less toxicity. For this, a cytotoxicity study was performed with cancer cell lines (MCF-7), whereas a hemolytic study was performed to evaluate acute toxicity of the formulation.
Hemolytic study
A hemolytic test was performed for zinc oxide nanoparticles using blood from a healthy donor. The supernatant of the RBCs having 100% hemolysis showed an absorbance value of 0.192 at 541 nm, whereas the supernatant of RBCs treated with zinc oxide nanoparticles showed an absorbance value of 0.008. The results of the study showed that zinc oxide nanoparticles caused 4.16% hemolysis. Hence, it can be suggested that zinc oxide nanoparticles are minimally toxic to the RBCs and hence to other normal body cells as well. From the study, it can be concluded that these nanoparticles could be useful for the administration of anticancer drugs with minimal toxicity.
Cell uptake study
A cell uptake study of the formulation was performed to quantitatively estimate the uptake of nanoparticles by the cancer cells. Flow cytometry was performed to estimate DOX uptake by the cancer cells (MCF-7). The mean fluorescence intensity (MFI) was estimated, which was found to be directly proportional to the cellular uptake of DOX. The results of the study revealed that the MFI of the cancer cells incubated with DOX-loaded ZnO nanoparticles was significantly higher (p < 0.05) than that of free DOX (). This may be ascribed to the greater uptake of the ZnO nanoparticles by the cancer cells. Uptake of ZnO nanoparticles by the cancer cells has already been reported earlier by CitationVimala et al. 2014. The mechanism involved in the internalization of ZnO nanoparticles is unclear. According to CitationPanariti et al. (2012), it is not possible to generalize the uptake pathway of nanoparticles, but it is reported that nanoparticles with a diameter more than 250 nm preferentially enter the cells via macropinocytosis. The surface charge of nanoparticles is also said to play an important role in the internalization, positively charged particles being internalized more quickly and in higher amounts. From the study, it can thus be concluded that ZnO nanoparticles are helpful in increasing the uptake of DOX inside the cells, and hence will show higher therapeutic activity as compared to free DOX.
Anti-proliferation study (MTT Assay)
The anti-proliferative activity of the prepared formulations was estimated by incubating cancer cells with formulations at varying concentrations of DOX for 24 h. In the case of blank ZnO nanoparticles, quantities were equivalent to the amount of ZnO nanoparticles in the drug-loaded formulations. Cell viabilities were determined by the MTT assay, and the results obtained are presented in .
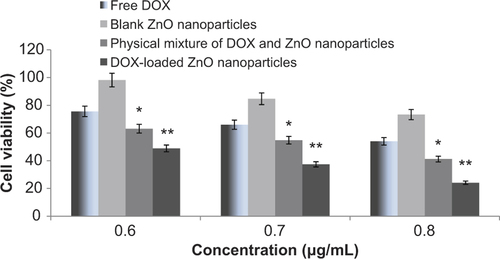
Cell viabilities were determined for 3 different concentrations (0.6 μg/mL, 0.7 μg/mL, and 0.8 μg/mL) each of free DOX, blank zinc oxide nanoparticles, mixture of DOX and blank nanoparticles, and drug-loaded nanoparticles. The results of the study clearly depict that ZnO nanoparticles have some inherent cytotoxic activity, as the cell viability was reduced to 98%, 84%, and 77% at the respective concentrations. Further, it has also been found that activity of free DOX is significantly increased (p < 0.05) when zinc oxide is co-introduced with the highly active anticancer drug DOX. This might be ascribed to the greater uptake of the DOX in the presence of ZnO nanoparticles, which showed a synergistic effect of both cytotoxic agents, i.e., ZnO nanoparticles and DOX (CitationVimala et al. 2014). However, the cytotoxicity of DOX-loaded nanoparticles was found to be significantly higher (p < 0.001) than the physical mixture of the two (at the same concentration of DOX and zinc oxide). This might be ascribed to the greater uptake of the DOX by endocytosis when loaded at the surface of the ZnO nanoparticles. Further, release of the drug from the particles might be have been controlled in the cellular environment, and showed better targeting ability as well. The results of the studies performed earlier by other research groups using different metal nanoparticles including iron oxide, gold etc., also demonstrated higher anticancer activity when anticancer drugs were loaded/conjugated to the surface of nanoparticles (methods for drug loading were different), in comparison to free drug (CitationKievit et al. 2011, CitationElbialy et al. 2014, CitationSun et al. 2014). Thus, from the present study, it can be suggested that DOX-loaded ZnO nanoparticles could be a promising carrier system for use in anticancer therapy.
Conclusion
The present study was initiated with the aim of developing a novel approach/carrier system for the delivery of anticancer drugs for better therapeutic efficacy. DOX-loaded ZnO nanoparticles were developed and characterized for various parameters. It was found that dispersion of drug-loaded ZnO nanoparticles was stable for 24 h, and released the drug in a controlled manner. The anticancer activity and efficiency of DOX-loaded nanoparticles were compared with that of DOX alone, blank ZnO nanoparticles, and a physical mixture of DOX and ZnO nanoparticles. It was found that ZnO nanoparticles exhibit some inherent anticancer activity. The mixture of ZnO nanoparticles and DOX caused higher cytotoxicity than either DOX or ZnO nanoparticles alone. This proves that the co-administration of DOX and ZnO nanoparticles produces synergistic cytotoxicity and thus higher anticancer activity. However, drug-loaded nanoparticles produced even higher cytotoxicity, i.e., the highest anticancer activity. This might be due to better targeting and retention of drug-loaded nanoparticles in the cancerous cells because of the better targeting ability of ZnO nanoparticles. Though ZnO nanoparticles have been studied as anticancer therapy earlier as well, both alone as well as in combination with the anticancer drug, this formulation proves to be better than the existing ones, as the drug is loaded onto the nanoparticles, which makes the therapy less troublesome. Moreover, as proved, it produces better cytotoxicity than ZnO nanoparticles, alone or in combination with the anticancer agent. Thus, it can be concluded that drug loading on ZnO nanoparticles is a novel and effective approach towards cancer management, with better targeting of the highly toxic chemotherapeutic drugs, thus producing fewer side effects. However, in vivo studies in animals should be performed, to better understand the mechanism and effect of the formulation developed for the treatment of cancer.
Acknowledgement
The authors want to acknowledge Sun Pharma Ltd., Baroda, for providing a gift sample of the drug. The authors also would like to thank Mr. Parveen Garg, Chairman, ISF College of Pharmacy, Moga, for providing facilities for the research.
Declaration of interest
The authors report no declarations of interest. The authors alone are responsible for the content and writing of the paper.
References
- Akhtar MJ, Ahamed M, Kumar S, Khan MMA, Ahmad J, Alrokayan SA. 2012. Zinc oxide nanoparticles selectively induce apoptosis in human cancer cells through reactive oxygen species. Int J Nanomedicine. 7:845–857.
- Bender EA, Adorne MD, Colomé LM, Abdalla DSP, Guterres SS, Pohlmann AR. 2012. Hemocompatibility of poly(ϵ-caprolactone) lipid-core nanocapsules stabilized with polysorbate 80-lecithin and uncoated or coated with chitosan. Int J Pharm. 426:271–279.
- Davis ME, Chen ZG, Shin DM. 2008. Nanoparticle therapeutics: an emerging treatment modality for cancer. Nat Rev Drug Discov. 7:771–782.
- Deerinck TJ. 2008. The application of fluorescent quantum dots to confocal, multiphoton, and electron microscopic imaging. Toxicol Pathol. 36:112–116.
- Degen A, Kosec M. 2000. Effect of pH and impurities on the surface charge of zinc oxide in aqueous Solution. J Eur Ceramic Soc. 20:667–673.
- Elbialy NS, Fathy MM, Khalil WM. 2014. Preparation and characterization of magnetic gold nanoparticles to be used as doxorubicin nanocarriers. Phys Med. 30:843–848.
- Galdiero S, Falanga A, Vitiello M, Cantisani M, Marra V, Galdiero M. 2011. Silver nanoparticles as potential antiviral agents. Molecules. 16:8894–918.
- Gilmore JL, Yi X, Quan L, Kabanov AV. 2008. Novel nanomaterials for clinical neuroscienceNovel Nanomaterials for Clinical Neuroscience. J Neuroimmune Pharmacol. 3:83–94.
- Guo D, Wua C, Jiang H, Li Q, Wanga X, Chen B. 2008. Synergistic cytotoxic effect of different sized ZnO nanoparticles and daunorubicin against leukemia cancer cells under UV irradiation. J Photochem Photobiol B. 93:119–126.
- Hanley C, Layne J, Punnoose A, Reddy KM, Coombs I, Coombs A, et al. 2008. Preferential killing of cancer cells and activated human T cells using ZnO nanoparticles. Nanotechnology. 19:295103.
- He F, Zhao D. 2005. Preparation and characterization of a new class of starch-stabilized bimetallic nanoparticles for degradation of chlorinated hydrocarbons in water. Environ Sci Technol. 39:3314–3320.
- Hong H, Shi J, Yang Y, Zhang Y, Engle JW, Nickles RJ, et al. 2011. Cancer-targeted optical imaging with fluorescent zinc oxide nanowires. Nano Lett. 11:3744–3750.
- Kievit FM, Wang FY, Fang C, Mok H, Wang K, Silber JR, et al. 2011. Doxorubicin loaded iron oxide nanoparticles overcome multidrug resistance in cancer in vitro. J Control Release. 152:76–83.
- Kim K, Lee M, Park H, Kim JH, Kim S, Chung H, et al. 2006. Cell- permeable and biocompatible polymeric nanoparticles for apoptosis imaging. J Am Chem Soc. 128:3490–3491.
- Kohler N, Sun C, Fichtenholtz A, Gunn J, Fang C, Zhang M. 2006. Methotrexate-immobilized poly(ethylene glycol) magnetic nanoparticles for MR imaging and drug delivery. Small. 2:785–792.
- Lai JR, Chang YW, Yen HC, Yuan NY, Liao MY, Hsu CY, et al. 2010. Multifunctional doxorubicin/superparamagnetic iron oxide- encapsulated Pluronic F127 micelles used for chemotherapy/magnetic resonance imaging. J Appl Phys. 107:09B318.
- Lee JH, Kim YG, Cho MH, Lee J. 2014. ZnO nanoparticles inhibit Pseudomonas aeruginosa biofilm formation and virulence factor production. Microbiol Res. 169:888–896.
- Maeng JH, Lee DH, Jung KH, Bae YH, Park IS, Jeong S, et al. 2010. Multifunctional doxorubicin loaded superparamagnetic iron oxide nanoparticles for chemotherapy and magnetic resonance imaging in liver cancer. Biomaterials. 31:4995–5006.
- Montet X, Weissleder R, Josephson L. 2006. Imaging pancreatic cancer with a peptide-nanoparticle conjugate targeted to normal pancreas. Bioconjug Chem. 17:905–911.
- Mukherjee P, Bhattacharya R, Wang P, Wang L, Basu S, Nagy JA, et al. 2005. Antiangiogenic properties of gold nanoparticles. Clin Cancer Res. 11:3530–3534.
- Nair S, Sasidharan A, Rani VVD, Menon D, Nair S, Manzoor K, Raina S. 2009. Role of size scale of ZnO nanoparticles and microparticles on toxicity toward bacteria and osteoblast cancer cells. J Mater Sci. 20:S235–S241
- Nam J, La WG, Hwang S, Ha YS, Park N, Won N, et al. 2013. pH- responsive assembly of gold nanoparticles and “spatiotemporally concerted” drug release for synergistic cancer therapy. ACS Nano. 7:3388–3402.
- Nasrolahi Shirazi A, Tiwari RK, Oh D, Sullivan B, McCaffrey K, Mandal D, Parang K. 2013. Surface decorated gold nanoparticles by linear and cyclic peptides as molecular transporters. Mol Pharm. 10:3137–3151.
- Nobili S, Landini I, Giglioni B, Mini E. 2006. Pharmacological strategies for overcoming multidrug resistance. Curr Drug Targets. 7:861–879.
- Palanikumar L, Ramasamy S, Hariharan G, Balachandran C. 2013. Influence of particle size of nano zinc oxide on the controlled delivery of Amoxicillin. Applied Nanoscience. 3:441–451.
- Palanikumar L, Ramasamy SN, Balachandran C. 2014. Size-dependent antimicrobial response of zinc oxide nanoparticles. IET Nanobiotechnol. 8:111–117.
- Panariti A, Miserocchi G, Rivolta I. 2012. The effect of nanoparticle uptake on cellular behavior: disrupting or enabling functions? Nanotechnol Sci Appl. 5:87–100.
- Ramanujan RV, Kayal S. 2010. Anti-cancer drug loaded iron–gold core–shell nanoparticles (Fe@Au) for magnetic drug targeting. J NanosCNanotechnol. 10:1–13.
- Reddy LS, Nisha MM, Joice M, Shilpa PN. 2014. Antimicrobial activity of zinc oxide (ZnO) nanoparticle against Klebsiella pneumoniae. Pharm Biol. 52:1388–1397.
- Seker S, Eser Elçin A, Yumak T, Sınağ A, Murat Elçin Y. 2014. In Vitro cytotoxicity of hydrothermally synthesized ZnO nanoparticles on human periodontal ligament fibroblast and mouse dermal fibroblast cells. Toxicol In Vitro. 28:1349–1358.
- Son SJ, Bai X, Lee SB. 2007. Inorganic hollow nanoparticles and nanotubes in nanomedicine Part 1. Dru.g/gene delivery applications. Drug Discov Today 12:650–656.
- Sun C, Fang C, Stephen Z, Veiseh O, Hansen S, Lee D, et al. 2008. Tumor-targeted drug delivery and MRI contrast enhancement by chlorotoxin-conjugated iron oxide nanoparticles. Nanomedicine. 3:495–505.
- Sun TM, Wang YC, Wang F, Du JZ, Mao CQ, Sun CY, et al. 2014. Cancer stem cell therapy using doxorubicin conjugated to gold nanoparticles via hydrazone bonds. Biomaterials. 35:836–845.
- Taccola L, Raffa V, Riggio C, Vittorio O, Iorio MC, Vanacore R, et al. 2011. Zinc oxide nanoparticles as selective killers of proliferating cells. Int J Nanomedicine. 6:1129–1140.
- Veiseh O, Kievit FM, Fang C, Mu N, Jana S, Leung MC, et al. 2010. Chlorotoxin bound magnetic nanovector tailored for cancer cell targeting, imaging, and siRNA delivery. Biomaterials. 31:8032–8042.
- Vimala K, Sundarraj S, Paulpandi M, Vengatesan S, Kannan S. 2014. Green synthesized doxorubicin loaded zinc oxide nanoparticlesregulates the Bax and Bcl-2 expression in breast and colon carcinoma. Process Biochem. 49 (1)::160–172.
- Wang H, Wingett D, Engelhard MH, Feris K, Reddy KM, Turner P, et al. 2009. Fluorescent dye encapsulated ZnO particles with cell specific toxicity for potential use in biomedical applications. J Mater Sci Mater Med. 20:11–22.
- Xiao Y, Hong H, Matson VZ, Javadi A, Xu W, Yang Y, et al. 2012. Gold nanorods conjugated with doxorubicin and cRGD for combined anticancer drug delivery and PET imaging. Theranostics. 2:757–768.
- Yadav A, Prasad V, Kathe AA, Raj S, Yadav D, Sundaramoorthy C, Vigneshwaran N. 2006. Functional finishing in cotton fabrics using zinc oxide nanoparticles. Bull Mater Sci. 29:641–645.
- Zhang Y, Wei C, Wang S, Liu Y, Pope C. 2008. Phototoxicity of zinc oxide nanoparticle conjugatesin human ovarian cancer NIH: OVCAR-3 cells. J Biomed Nanotechnol. 4:432–438.