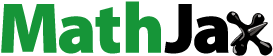
Abstract
The blending of polymers is of great interest, since the modification gives rise to diverse physical properties with the functionality of a polymer, without synthesis. Water-soluble antitumor-active poly(maleic anhydride-alt-acrylic acid) poly(MA-alt-AA) and poly(ethylene glycol) (PEG) blends were prepared by casting, and compatible properties were investigated by dilute solution viscometry. Viscosity measurements were made on ternary systems of polymer (1)/polymer (2)/solvent (H2O) and p-dioxane, at different concentrations of PEG and poly(MA-alt-AA). The interaction parameters Δβ, μ, Δk, Δb, β and α, which have been proposed, have been obtained using the viscosity data, to probe the miscibility of the polymer blends. The solid blends prepared were characterized with ATR-FTIR, 1H-NMR, DTA and TGA.
Introduction
Polymer blending has been identified as the most versatile and economical route to producing new multi-phase polymeric materials that are able to satisfy the complex demands of performance (CitationKhutoryanskiy 2003, CitationMittal 2012). The proper location of functional groups, as well as its structure, are usually responsible for the biological activity, biocompatibility and/or biodegradability of a polymer, and may have an impact on its therapeutic and/or toxic character. According to the goal, appropriate functional groups and ligands can be designed. Carboxylic groups induce therapeutic activity of many drugs; therefore, novel, biologically active, anhydride-containing polymers are expected to show considerably high biological activities because of the anionic character of the polymers formed after their hydrolysis in the medium (CitationRingsdorf 1975, CitationOttenbrite 1982). The development of various hydrophilic materials based on blends and interpolymer complexes of poly(carboxylic acid)s and non-ionic water-soluble polymers, which can be defined as drug polymers (CitationSpridon et al. 1997), is of great importance, because of their unique properties and possible applications in medicine. Several synthetic polyanions have become objects of interest to investigators for comparison of their physiological properties with those of naturally occurring polyanions (CitationFeltz and Regelson 1962, CitationMarkovsky et al. 2014) such as poly(acrylic acid), poly(methacrylic acid), poly(ethylene-co-maleic anhydride), and oxidized polysaccharides (CitationRegelson 1967, CitationTong and Cheng 2007). Subsequently, the copolymer of divinyl ether and maleic anhydride has also shown significant antitumor activity (CitationOttenbrite 1982).
The maleic anhydride (MA) copolymers as polymer drugs in medicine were described for use as antitumor agents, drug carriers, supporters for enzyme, or protein modifiers. Based on studies of the polycarboxylic acid-polysaccharide blend, it was shown that the combination of two polymers allows the preparation of very good drug carriers with the characteristics of solubility, structure, and compatibility (CitationOttenbrite 1985).
Carboxylic groups induce the therapeutic activity of many drugs, and therefore, novel and biologically active anhydride-containing polymers (CitationRegelson 1967, CitationPutnam and Kopeček 1995) are expected to show considerably high biological activity due to the anionic character of the polymers formed after their hydrolysis in the medium (CitationKaplan Can et al. 2005, Citation2006a).
Miscibility is defined as the ability to be mixed at a molecular level to produce a homogeneous mixture. The miscibility between the constituents of a polymer mixture plays a significant role in producing new polymer blends (CitationColeman et al. 1995). A combination of synthetic or natural polymers results in new functional materials, which have specific properties of synthetic components (good mechanical properties, diverse possibilities, low production expense, etc.) and biocompatibility and usage of polymers (CitationKaplan Can et al. 2006b). The carboxylic acid-containing groups exhibiting high biological activity in the poly (MA-alt-AA) component are used in the preparation of blends (CitationKavlak et al. 2004). Using compatible blended polymers is the most effective way to produce new multipurpose materials. Recent studies (CitationCascone et al. 1997) have shown that bioartificial polymeric materials can be obtained from miscible dextran/poly(acrylic acid) mixtures. Dextran/maleic acid-vinyl acetate polymeric blends have been studied, and their intermolecular interactions have been investigated by CitationBumbu et al. (2001). The compatibility of synthetic polymer blends is gaining increasing attention due to interpolymer interactions which enable the preparation of new materials with biomedical applications (CitationGaray et al. 1997). In the blending process, PEG has been more widely used due to a number of reasons; PEG has a number of other uses, apart from serving as a polymeric carrier, and is known to be non-toxic and non-immunogenic; its flexible, highly water-soluble chain provides a hydrodynamic radius, and it also shows a high degree of hydration. The low molecular weight of PEG makes it the most suitable material to be classified as an impact modifier for copolymers, due to its miscibility, biodegradability, and food contactable applications (CitationBuong et al. 2014, CitationMarkovsky et al. 2014). Many analytical and spectroscopic methods such as FTIR, DSC, DTA, DMA, TGA, 1H-NMR, XRD, and SEM, can be used for the determination of experimental and theoretical estimates of the miscibility of polymer blends (CitationAubin et al. 1983). The techniques mentioned for studying the miscibility of the polymer blends may be obscurant, prohibitive, and time consuming. Viscometry is an easy and very useful method for studying the miscibility of the polymer blends (CitationChee 1990). Using the dilute solution viscometry method, a report about both polymer–polymer interactions and polymer–solvent interactions in solution, can be confected. In addition, the dilute solution viscometry method provides information about both polymer–polymer interactions and polymer–solvent interactions in solution. Blend systems involving neutral or uncharged polymers in organic solvents, complex mixtures of polymers and microemulsions, neutral and water-soluble polymers in water, and mixtures of polyanions in aqueous solvent, can be studied by viscometry.
In this study, after the water-soluble poly(MA-alt-AA) is synthesized via charge transfer complex copolymerization (CTC), polymeric blends were prepared with PEG, at various ratios. The miscibility behavior of poly(MA-alt-AA) with PEG was investigated with dilute solution viscosity. Solid blends were characterized with ATR-FTIR, 1H-NMR, DTA, and TGA. Dilute solution viscosity measurements were performed on ternary systems of polymer(1)/copolymer(2)/solvent (H2O), for three different ratios of PEG/Poly(MA-alt-AA) blends – 0/100, 25/75, 50/50, 75/25, and 100/0, in distilled and deionized water, at 25°C. The intrinsic viscosity and viscometric interaction parameters were experimentally measured by the classical Huggins equation. The degree of miscibility of this polymer system will be estimated on the basis of the following criteria; the sign on the values of Δβ, μ, Δk, Δb, β and α. Based on the sign convention involved in these criteria and the ATR-FTIR, the results of the 1H-NMR spectral analysis and thermal analysis will be confirmed for the compatibility/miscibility of PEG–Poly(MA-alt-AA) blends.
Theoretical
The miscibility parameters obtained by dilute solution viscometry and derived from the classical Huggins equation are given by (CitationHuggins 1942)
where ηsp is the specific viscosity of a single-solute solution, c is the mass concentration, [η] is the intrinsic viscosity, and b is related to the Huggins coefficient k by
where b is believed to reflect the binary interactions between polymer segments. The value of the Huggins coefficient is a measure of the interpenetration of polymer coils, the extent of which depends upon the segment–segment and segment–solvent interactions. This, in turn, affects the intermolecular hydrodynamic interaction and molecular dimensions. EquationEquations (1)(1)
(1) and Equation(2)
(2)
(2) can be adapted by Krigbaum and Wall to a polymer (1)/polymer (2)/solvent system(2) (CitationKrigbaum and Wall 1950).
Subscripts 1, 2 and m correspond to polymers 1, 2 and their blends, respectively. The plot of reduced specific viscosity of poly blend ηsp,m/(c1 + c2) vs total polymeric concentration (C = c1 + c2) yields a straight line, and the intercept and gradient correspond to [η] m and bm, respectively . Theoretically, bm(c1 + c2) reflects the total molecular interaction. It is composed of three terms: b11c12, b22c22 and 2b12c1c2, corresponding to polymer (1)–solvent, polymer (2)–solvent, and polymer (1)–polymer (2) interactions, respectively. Comparing it with EquationEq. (1)(1)
(1) for single component, the specific viscosity of a polyblend is
As described by Krigbaum and Wall, the information on the intermolecular interaction in binary polymer blends (polymer 1 and polymer 2) can be obtained from a comparison of the experimental interaction coefficient b12exp and the theoretical values b12id. The ideal interaction coefficient, b12id between two polymers can be expressed as
Experimental math type bmexp values were estimated by the following equation:
The terms w1 and w2 indicate the weight fraction of the components (1) and (2), and b11 and b22 are the slopes of viscosity curves for the pure components (1) and (2), respectively. The bmexp values were obtained from the slope of EquationEq. (7)(7)
(7) .
Catsiff and Hewett have proposed to define the ideal value of the interaction parameter, b12id, as the arithmetic mean value (6) (CitationCatsiff and Hewett 1962), as follows:
If ∆b > 0 or ∆b’ > 0, there exist attractive intermolecular interactions and miscibility in binary polymeric systems, whereas ∆b < 0 or ∆b’< 0 indicate phase separation.
CitationSun et al. (1992) suggested a new criterion for polymer–polymer miscibility in the blends, in terms of a thermodynamic parameter based on the classical Huggins coefficient km, by using the equation
According to the approach of Sun et al., for a ternary polymer A/polymer B/solvent system, three types of interactions might contribute to the value km. These are long range hydrodynamic interactions of pairs of single molecules, defined by km1, which is the same as km given in EquationEq. (10)(10)
(10) . The formation of double molecules is given by km2, and the intermolecular attraction or repulsion given by km3. Thus, the overall km turns out to be
The second term, km2, can be neglected at sufficiently low concentrations and in the absence of strong specific interactions that would encourage aggregation. Redefining km3 as α and rearranging the EquationEq. (11)(11)
(11) , α is given as:
Based on the term km, Sun et al. thought that the value of α can be used to determine the miscibility of polymer blends: when α is less than zero, the blend is immiscible; when α is greater than zero, it is miscible.
Many miscibility parameters have been proposed by researchers to determine polymer–polymer miscibility by the viscometric method, such as ∆k, ∆b, α, β, ∆B, and μ. The common point of all these parameters is that the miscibility parameter should appear to be greater than zero, for the observation of miscibility.
For miscibility to occur, a larger, positive number will represent the phenomenon more clearly. The equations used to calculate all the above-mentioned miscibility parameters (Δk, Δb, α, β, ΔB, and μ) are summarized in . A more detailed analysis of these equations can be found in (CitationKavlak et al. 2004, CitationKaplan Can et al. 2006b), a previous work.
Table I. Summary of equations of miscibility parameters (Δb, Δk, α, β, ΔB, and μ).
Experimental methods
Materials
Acrylic acid (AA) (Fluka) was distilled before use. It has the following average characteristics: bp 141.6°C, mp 13.5°C, d420 1.0510, and nD20 1.4215. Maleic anhydride (MA) (Fluka) was purified before use by recrystallization from anhydrous benzene and by sublimation in vacuum, and it has an mp of 52.8°C. Poly(ethylene glycol) (PEG), with a molecular weight Mn of 3350 g/mol, was purchased from Aldrich. Benzoyl peroxide (BPO) (Fluka) was purified by recrystallizing twice from chloroform solution by methanol, and then it was dried under vacuum, and it has an mp of 106°C. The other reagents, including organic solvents, were purified by ordinary methods. The water used was bidistilled and deionized, with almost zero conductivity.
Synthesis of poly (maleic anhydride-alt-acrylic acid)
Copolymerization of MA with AA was carried out in p-dioxane, in the presence of benzoyl peroxide (BPO) (0.1%) as an initiator, at 70°C, under a nitrogen atmosphere for 8 h, in a Pyrex tube (). The poly (MA-alt-AA) was isolated from the reaction mixture by re-precipitation with anhydrous methanol and n-hexane, respectively. Copolymers were purified by reprecipitating twice from p-dioxane solution with n-hexane, and by washing with several portions of hexane, benzene and diethyl ether, and were dried in vacuum at 40°C to a constant weight with almost quantitative yields (CitationKaplan Can et al. 2005). The reaction mixture was cleaned and purified using ethanol and n-hexane, and then dried under moderated vacuum (Scheme 1).
Preparation of polymer blend
The powders (poly((MA-alt-AA)) and PEG) were dried in a vacuum oven to remove the free water from the samples before use. Poly(MA-alt-AA) and PEG were separately dissolved in water, to form a 1% (w/v) solution. The blends of stock solutions at different ratios (0/100, 25/75, 50/50, 75/25, and 100/0) were obtained by mixing for a certain period and then removing the solvent. The polymer blend was then purified to constant weight in a vacuum and then oven dried, and the polymer–polymer mixture was used in the experimental section as a solid phase of the study.
Experimental techniques
Viscosity studies
The viscometric behaviors of poly(MA-alt-AA) and poly(MA-alt-AA)/PEG/H2O solutions and poly(MA-alt-AA)/PEG/p-dioxane were performed using a Ubbelohde type capillary viscometer. The intrinsic viscosities of the polymer samples were determined in water at 25 ± 0.1°C, and in the concentration range of 0.1–1.0g/dL, using an Ubbelohde viscometer. The measurements of viscosity of the polymer solutions were obtained using 5 compositions, in the ratios of 0/100, 25/75, 50/50, 75/25 and 100/0; the measurements started after an equilibrium time of 10 min. The average elution times of the solutions were determined after several measurements. The values of [η] were determined from the plot of ηsp/c vs c, the concentration of polymers, using the following equation:
where ηsp is known as specific viscosity. Non-interaction of the polymer coils requires infinite dilution, and this is achieved mathematically by defining a quantity called the intrinsic viscosity, [η], according to the equation
Proton nuclear magnetic resonance (1H-NMR) spectroscopy studies
1H-NMR spectra were collected for the polymers and blends using a Bruker 400 MHz NMR spectrometer. Samples were prepared in DMSO-d6 at 25°C, and for the 1H-NMR analysis, TMS was used as an internal standard.
Attenuated total reflectance-fourier transform infrared spectroscopy studies
Attenuated Total Reflectance-Fourier Transform Infrared Spectroscopy (ATR-FTIR) spectra of poly(MA-alt.-AA), PEG, and poly(MA-alt-AA)–PEG blends, were taken with a Mattson FTIR spectrophotometer in the 4000–400 cm− 1 range, and 30 scans were taken at a resolution of 4 cm−1.
Thermal analysis studies
Differential thermal analysis (DTA) and thermogravimetric Analysis (TGA) of the structures of the copolymer, PEG and copolymer-PEG blends of different compositions, were recorded using a Shimadzu DTG-60H thermal analyzer in a dynamic nitrogen atmosphere (100 mL/min) at a heating rate of 10°C/min, with platinum crucibles as the sample vessels, and using α-Al2O3 as the reference.
Studies for determination of molecular weight
Gel Permeation Chromatography (GPC, Polymer Laboratories PL-GPC 220) was used to investigate the molecular weight distribution of the synthesized copolymer using tetrahydrofuran (THF) as the solvent. The average molecular weight of the copolymer can be given as and
, and the polydispersity index (HI) can be given as HI = 1.36 (by GPC analysis).
Results and discussion
ATR-FTIR spectroscopy studies of blends
FTIR spectroscopy has also been widely used by many researchers to study the formation of polymeric blends (CitationMoskala et al. 1984). The use of the FTIR spectra and the computationally calculated data is a good method for measuring interactions that occur between ethylene glycol and related copolymers when they are blended, because the experimental spectra show the actual interactions, whereas the computational ones are only for mechanical mixtures of two polymers (CitationColeman et al. 1991). The most common strong interaction in polymers, and one that is central to the properties and phase behavior of a range of important materials, is hydrogen bonding. The ATR-FTIR spectra of PEG, copolymer and blend samples, display characteristic absorption bands ( and ). The ATR-FTIR spectrum of poly(MA-alt-AA) in (monomer feed 50/50) can be seen in . In the spectrum of poly(MA-alt-AA), the broad transmission bands at 3058 cm− 1 are produced by stretching of the hydroxyl groups, multiple C–H stretching of methylene groups at 2881 cm− 1 (symmetric) and 2861 cm− 1 (asymmetric), –CH2 groups at 2930 cm− 1 (asymmetric) and 2794 cm− 1, and –COOH at 2503 cm− 1 (broad spectrum). The two bands at 1861 and 1781 cm− 1 are attributed to symmetric and asymmetric stretching of the anhydride carbonyl of copolymer respectively. The band at 1699 cm− 1 is attributed to the ester carbonyl stretching monomer units. The single bands at 1069 cm − 1 can be attributed to the asymmetrical stretching of the C–O–C anhydride ring. The bands at 1120 and 1171 cm− 1 are due to C–O stretching. The absorbance intensity is substantially diminished and new bands at 1970, 1593, and 1630 cm− 1 are observed. The band at 3058 cm− 1 is due to the C–H stretching of the aromatic ring. We observe the –C = O stretching band of maleic anhydride at 1783.6 cm− 1 (low intensity), and a band at 1695.5 cm− 1 (strong intensity), which are due to the –C = O stretching group of partially hydrolyzed maleic anhydride. Hydrogen bonding occurs between proton-accepting groups of the non-ionic polymers and non-ionized carboxylic groups of poly (carboxylic acids); complex formation also depends on the degree of hydrolysis of a poly (carboxylic acid) ().
![Figure 1. ATR-FTIR spectrum of poly(MA-alt-AA) copolymer [MA with AA was carried out in p-dioxane in the presence of benzoyl peroxide (BPO) (0.1%) as an initiator at 70°C, under a nitrogen atmosphere, for an 8 h monomer feed MA/AA →50/50].](/cms/asset/8c101a6a-e0cb-4d55-920a-a0dd353fc8a1/ianb_a_980506_f0001_b.gif)
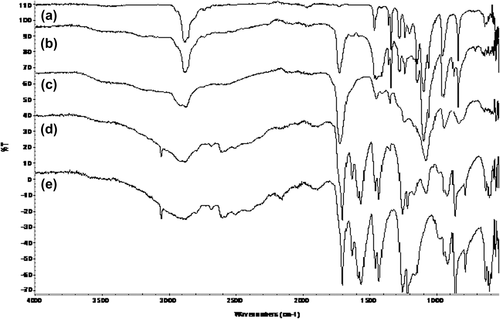
In the spectrum of PEG (), the O–H stretching is centered at 3490 cm− 1, multiple –C–H stretching of methylene at 2880 cm− 1, and –C–H bending and rocking vibrations are seen at 1467, 1413 and 962 cm− 1; moreover, –O–H bending vibrations at 1281 and –C–O bands at 1149, 1100 and 1060 cm− 1 are also seen. The ATR-FTIR spectra of poly(MA-alt-AA)/PEG blends (0/100, 25/75; 50/50, 75/25, and 100/0) are shown in . From , it can be easily seen that there are significant differences between the pure polymer and its blends. Significant changes, especially the bands at 2900, 1590, 1490, 1250 and 950 cm− 1, can be observed. The blend spectra show clear evidence of specific polymer–polymer interactions due to the band shifts. The shifts of some bands and the formation of new bands suggest that the blend structure between the PEG homopolymer and anhydride containing copolymer in 50/50 ratio is observed. This shift reveals that there is a favorable interaction between poly(MA-alt-AA)/PEG.
When the copolymer composition reaches up to the 75 wt % (maximum poly(MA-alt-AA) composition) interest of copolymer to the PEG decreases due to the carbonyl and carboxyl groups of copolymer, which are associated by intramolecular hydrogen bonding (CitationYu et al. 2007). Another proof of this behavior can be explained by the unchanged placement of the original bands at 75 wt % of the mixture containing copolymer. These results show that the PEG and poly(MA-alt-AA) are more compatible at 50/50 composition than at other blend compositions, since maximum peak displacement can be obtained (CitationKaplan Can et al. 2006b).
Proton nuclear magnetic resonance (1H-NMR) spectroscopy studies of blends
To determine the specific carbon or hydrogen which is affected by the amount of blending, 1H-NMR studies were performed. The NMR spectra of PEG, poly(MA-alt-AA), and poly(MA-alt-AA)/PEG blend samples at given blend compositions can be seen in . The expected specific chemical shifts for samples can be seen from the 1H-NMR spectra in DMSO-d6. The detailed characterization of poly(MA-alt-AA) copolymer was previously studied and presented by Kaplan Can et al. in 2005. In the spectra moving from PEG to the poly(MA-alt-AA), it can be easily seen that the specific chemical shifts belong to the copolymer. Also, the peak position and peak intensity can be followed depending upon the blend composition. The important peak in the 1H-NMR spectra belongs to the maleic anhydride (MA) unit, δ = 6–6.3 ppm, the –CH protons which can be easily followed, and no such band could be observed in any other composition of blends (). Aliphatic protons of –CH2 in copolymers are located between 1–1.5 ppm and at 3.0 ppm, respectively. The resonance signal at 12 ppm was attributed to the –COOH group in the partially hydrolyzed form of the copolymer. The composition PEG/poly(MA-alt-AA) (50/50) can be accepted as having maximum miscibility, since the existence of the H–bonding can be attributed to the –COOH groups of the copolymer and the carbonyl group of the PEG segments. For the pure PEG, hydrogen atoms are assigned at δ = 3.0–3.5 ppm. As the blend composition changes from PEG to the poly(MA-alt-AA), specific resonance lines of the copolymer and broadening of the peaks are observed.
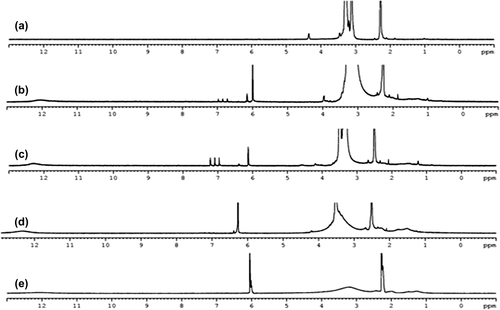
The maximum shift can be observed at blend compositions of 50/50. When the copolymer content is high, the intra molecular interaction is more dominant, and therefore the shift is less than that which would occur at the lower content of copolymer (25/75). The composition of the functional group containing polymers may reflect the H–bonding interaction between the highly reactive carbonyl and carboxylic acid groups. According to this base, the maximum compatibility can be observed at a polymer composition of 50/50 wt %. With increased functional copolymer in the blend, intramolecular H–bonding is more dominant than the intermolecular H–bonding interactions (CitationPark et al. 2005). Prepared polymer blend (50/50), estimated with increasing severity of the hydrogen bond cleavage and a 50/50 ratio of the blend, is thought to be optimum in terms of miscibility. The association of poly(carboxylic acids) and non-ionic polymers in solutions via hydrogen bonding results in the formation of novel polymeric materials (CitationZhong et al. 2012).
Investigation of the thermal properties of poly(MA-alt-AA)/PEG blends
Whether or not there was mixing of the polymeric blend composition depended on the composition and temperature of the components. This means it was achieved in a limited range of miscible blends. Interactions between the groups and the polymer segments cause a reduction of the Gibbs energy (∆G) in a miscible blend. Many methods are available to examine the miscibility of a polymer blend (CitationSwamy 2007). In miscibility studies, often a physical characteristic such as glass transition temperature was used as control. Single glass transition temperature miscibility refers to polymer–polymer miscibility (CitationUtracki 2002). Differential Thermal Analysis (DTA) allows the detection of glass transition. Thermal Gravimetric Analysis (TGA) also gives information for blend miscibility with respect to thermal stability. For the copolymer/PEG combination, experimental details for the thermal behavior of the pure polymers and their blends can be seen from the . DTA and TGA data for the following blend ratios of poly(MA-alt-AA)/PEG – (1) 0/100, (2) 25/75, (3) 50/50, (4) 75/25 and (5) 100/0 – were used. The second order transition temperature, the glass transition temperature (Tg), presents a value of 62°C for the PEG, and the blend that contained copolymer shows the higher values at 147°C, for the PEG/copolymer blend composition of 25/75. The theoretically expected Tg value of 50/50 blends was also experimentally found to be between that of the pure PEG and pure poly(MA-alt-AA). When the quantity of copolymer increases in the blend system, the chain mobility decreases due to the intra- and intermolecular interactions, especially the hydrogen bonding of the binary system. The modification in the transition temperature may be attributed to the interactions between components of the blend with the copolymer, as mentioned, and may also be due to the plasticizer role of PEG. According to the Tg results from the DTA, the results support the result obtained by viscometry that the mixture of copolymer/PEG in a ratio of 50/50 is miscible, and at ratios of 25/75, 75/25, the other blend combinations are immiscible, respectively. A miscible polymer blend will exhibit a single glass transition between the Tgs of the components, with a sharpness of the transition similar to that of the components.
Table II. DTA and TGA data for the pure polymers and their blends ratios of poly(MA-alt-AA)/PEG; 0/100, 25/75, 50/50, 75/25 and 100/0.
and show the percentage of weight loss as a function of temperature for poly(MA-alt-AA)/PEG, and their different blend compositions, with heating rate of 10°C/min at the temperature range from room temperature to 800°C. It is evident from that the initial weight loss for all the samples starts at around 100 °C, with a maximum of 3.3% loss of weight. This may correspond to the loss of bound water from pure polymers and their blends, especially in the 50/50 composition of copolymer (CitationQin et al. 2002). The second weight loss starts at around 200°C and continues up to 400°C melting temperature, Tm, during which there was a 40.5% loss in weight. The third weight loss was observed in the range from 450–500°C, which may correspond to the structural decomposition of the blend, during which there was 64.0% weight loss (). For the thermal stability, degradation shapes and steps depend on the copolymer, structure, and interaction between PEG and copolymer. Using data obtained from DTA and TGA, results show that the ratio of 50/50 is compatible with most of the blends, whereas blends in the ratio of 75/25 show properties peculiar to homopolymers.
Investigation of viscosity properties and assessment of miscibility parameters of blends
The viscometric method is used primarily for the investigation of miscibility of the polymer blend of the pure polymers, and the blend and flow time of the solvent. Before a discussion of data on poly(MA-alt-AA)/PEG mixtures, it is necessary to characterize the viscometric behavior of each polymer separately in aqueous solutions. By measuring the dynamic viscosity of pure polymer, blends in solvent at 25°C ± 0.1, ηrel, ηsp, [η] were calculated according to Huggins equation values. In aqueous solution, the polymers and blends showed the polyelectrolyte behavior as observed in a polar solvent system () (CitationKaplan Can et al. 2005). Considering the behavior of prepared polymer and polymer blends in water, the results show increased viscosity at low concentrations. This polyelectrolyte behavior can be seen and slightly eliminated by using p-dioxane as a non-polar solvent (). In results of viscosity experiments in polymer (1) and polymer (2), and use of solvent and different regions of the linear regression (LR) as a result, values of intrinsic viscosity [η], calculated using the Huggins equation and Huggins constant, kh, are presented in . According to , intrinsic viscosity values for polymeric blends vary between those of pure polymers, indicating the existence of intermolecular interactions between the unlike polymer segments when the pure polymers are mixed. Based on the experimentally observed values [η] for the binary (polymer/water) and ternary (polymer 1/polymer 2/water) systems, the parameters of the compatibility criteria proposed by Krigbaum and Wall, Sun et al., Catsiff and Hewitt and Chee were computed by related equations (CitationKavlak et al. 2004). The miscibility parameters Δb, μ, α, ΔB and Δk, calculated according to the weight fraction of poly(MA-alt-AA)/PEG, are illustrated in . For the sake of simplicity, and to compare the miscibility of polymer blends, miscibility parameter values are depicted as a function of composition. Generally, the values of parameters ΔB and μ obtained for the blends studied are more negative than the other miscibility parameters. In spite of this contradiction, a common trend is observed with all miscibility parameters (CitationKaplan Can et al. 2006b). For the blend system studied, the miscibility of copolymer and PEG shows a tendency that the copolymer composition increases. Poly(MA-alt-AA)/PEG blend systems having composition 25/75 have negative values of all miscibility parameters (Δk, Δb, α, β, ΔB, and μ) at same temperature and casting solvent studied, suggesting that the blend system may exhibit phase separation, and hence, immiscibility. The possible reason for the disagreement between these four methods of determining (∆k, μ, ∆b, and α) is probably the fact that viscometric criteria do not take into account interactions between the polymer and solvent, but attribute the miscibility of two polymers only to the specific interactions between the unlike polymer molecules.
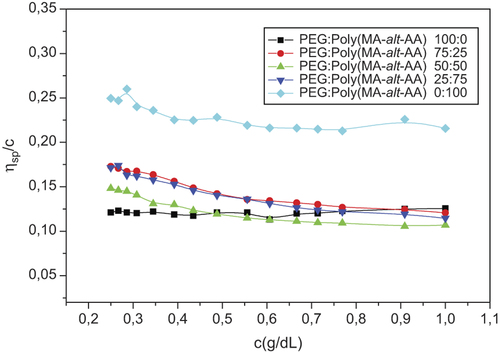
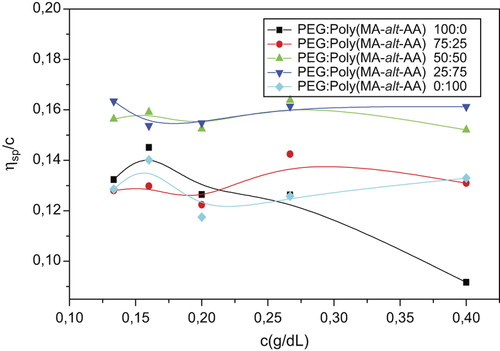
Table III. Huggins constant, kH, and intrinsic viscosity [η], calculated according to the Huggins equation, with different mixing ratios of poly(MA-alt-AA):PEG at 25°C ± 0.1 in water.
Table IV. Calculated intrinsic viscosity, [η], and Huggins constant, kH, according to the Huggins equation in the viscosity experiments of poly (MA-alt-AA)/PEG, with different mixing ratio at 25°C ± 0.1 in water.
According to studies on ternary systems of poly(MA-alt-AA)/PEG/H2O blends, polymers prefer to interact with H2O molecules individually, since H2O is a small molecule compared with PEG and poly (MA-alt-AA), and it also has two hydrogen bonding units. For the blend solutions composed of H-bond interacting groups, being non-ionic, they do not possess sufficient contrast homogeneously in the coils of the solution. The H-bonding between the unlike chains not only forms interchain connections but also influences the chain conformation, that is, it causes the interaction pairing between unlike segments, and thus the chains lose their solvation ability (). Namely, in addition to the H-bond interaction and H-bond formation between the PEG and poly(MA-alt-AA) molecules in aqueous solutions, there is the possibility of the hydrogen bond formation between the water and each of the polymers (CitationKaplan Can et al. 2005).
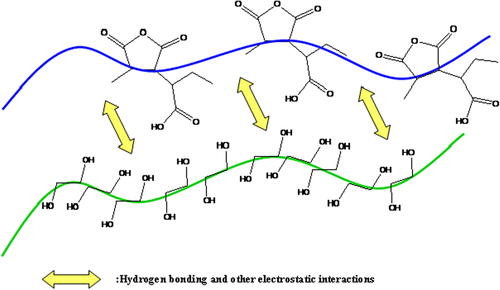
The H-bond interactions should be responsible for the miscibility of the PEG and poly(MA-alt-AA). It can be proposed that favorable interactions occur between –OH groups of the poly (MA-alt-AA) and –OH groups and etheric oxygen of PEG. Under the conditions where immiscibility is exhibited between the PEG and poly (MA-alt-AA), H-bonding interaction between the two polymers should be strong enough to overcome the intra- and intermolecular H-bonding between the polymer and solvent (CitationKavlak et al. 2004).
Conclusions
Water-soluble antitumor-active poly(maleic anhydride-alt-acrylic acid) (poly(MA-alt-AA) and poly(ethylene glycol) (PEG) polymer blends were prepared by casting from water at room temperature, and their compatibility properties were investigated by dilute solution viscometry and in solid state, by spectroscopic methods such ATR-FTIR, 1H-NMR, and thermal analysis methods such as DTA and TGA. Using the viscosity data, interaction parameters (Δk, Δb, α, β, ΔB, and μ) were computed. These values revealed that the blend is miscible when PEG content is 50/50 and 25/75 in the ternary polymer blend system. According to the parameters of miscibility in the viscosity based test, the miscibility of the blends with 50/50 and 25/75 ratio theoretically prepared in the blender can be obtained. Under this critical concentration, the blends were found to be immiscible and undergo phase separation. From our theoretical and experimental observations it is clear that all miscibility parameters show the tendency for miscibility. Viscometric methods can be used to obtain results with a fairly good accuracy in determining miscibility/compatibility of two polymers. All other methods used also strongly support specific interactions between the copolymer and PEG in the blend system. The association of poly(carboxylic acids) and non-ionic polymers (PEG) in solutions via hydrogen bonding results in the formation of novel polymeric materials. These materials can potentially be used and hold promise for the design of novel polymer-drug conjugates, solubilization of poorly antitumor soluble drugs, mucoadhesive dosage forms, development of solid drug dispersions, and encapsulation technologies.
Declaration of interest
The authors of the manuscript solemnly declare that no scientific and/or financial conflicts of interest exist with other people or institutions.
References
- Aubin M, Bédard Y, Morrissette MF, Prud’Homme RE. 1983. Miscible blends prepared from two crystalline polymers. J Polym Sci Polym Phy Ed. 21:233–240.
- Bumbu GG, Vasile C, Chitanu GC, Carpov A. 2001. On the compatibility of polysaccharide/maleic copolymer blends I. Thermal behaviour of dextran containing blends. Polym Deg Stab. 72:99–108.
- Buong WC, Ibrahim NA, Wan MZ, Mohd ZH. 2014. Poly(lactic acid)/poly(ethylene glycol) polymer nanocomposites: effects of graphene nanoplatelets. Polymers. 6:93–104.
- Cascone MG, Polacco G, Lazzeri L, Barbani N. 1997. Dextran/poly (acrylic acid) mixtures as miscible blends. J Appl Polym Sci. 66:2089–2094.
- Catsiff EH, Hewett WA. The interaction of two dissimilar polymers in solution. 1962. J Appl Polym Sci. 6:S30–S32.
- Chee KK. Determination of polymer-polymer miscibility by viscometry. 1990. Eur Polym J. 26:423–426.
- Coleman MM, Graft JF, Painter PC. 1991. Specific Interaction and the Miscibility of Polymer Blends. Lancaster, PA: Technomic Publishing.
- Coleman MM, Painter PC, Graf JF. 1995. Specific Interactions and the Miscibility of Polymer Blends. Boca Raton, FL: CRC Press.
- Feltz ET, Regelson W. 1962. Ethylene maleic anhydride copolymers as viral inhibitors. Nature. 196:642–645.
- Garay TM, Llamas CM, Iglesias E. 1997. Study of polymer-polymer complexes and blends of poly (N-isopropylacrylamide) with poly (carboxylic acid): 1. Poly (acrylic acid) and poly (methacrylic acid). Polymer. 38:5091–5096.
- Huggins ML. 1942. The viscosity of dilute solutions of long-chain molecules. IV. Dependence on concentration, J Am Chem Soc. 64:2716–2718.
- Kaplan Can H, Doğan AL, Rzaev ZMO, Hasegeli Üner A, Güner A. 2005. Synthesis and antitumor activity of poly (3, 4-dihydro-2H-pyran-co-maleic anhydride-co-vinyl acetate). J Appl Polym Sci. 96:2352–2359.
- Kaplan Can H, Doğan AL, Rzaev ZMO, Hasegeli Üner A, Güner A. 2006a. Synthesis, characterization, and antitumor activity of poly (maleic anhydride-co-vinyl acetate-co-acrylic acid). J Appl Polym Sci. 100:3425–3432.
- Kaplan Can H, Barsbay M, Güner A, Rzaev ZMO. 2006b. Experimental and theoretical approaches to investigating the miscibility of anhydride-containing copolymers and dextran. J Appl Polym Sci. 102: 2132–2141.
- Kavlak S, Kaplan Can H, Güner A. 2004. Miscibility studies on poly (ethylene glycol)/dextran blends in aqueous solutions by dilute solution viscometry. J Appl Polym Sci. 94:453–460.
- Khutoryanskiy VV, Cascone MG, Lazzeri L, Nurkeeva ZS, Mun GA, Mangazbaeva RA. 2003. Phase behaviour of methylcellulose–poly (acrylic acid) blends and preparation of related hydrophilic films. Polym Int. 52:62–67.
- Krigbaum WR, Wall FT. 1950. Viscosities of binary polymeric mixtures. J Polym Sci. 5:505–514.
- Markovsky E, Baabur-Cohen H, Satchi-Fainaro R. 2014. Anticancer polymeric nanomedicine bearing synergistic drug combination is superior to a mixture of individually-conjugated drugs. J Control Release. 187:145–157.
- Mittal V. 2012. Functional Polymer Blends: Synthesis, Properties and Performance USA: Taylor and Francis (CRC Press).
- Moskala EJ, Howe SE, Painter PC, Coleman MM. 1984. On the role of intermolecular hydrogen bonding in miscible polymer blends. Macromolecules. 17:1671–1678.
- Ottenbrite RM. 1982. The Antitumor and Antiviral Effects of Polycarboxylic Acid Polymers. ACS Symposium Series, Vol 186, Chapter 16,Washington, D.C.: American Chemical Society. 205–220.
- Ottenbrite RM. 1985. Antitumor activity of polycarboxylic acid polymers. J Macromol Sci Chem. 22:819–832.
- Park Y, Veytsman B, Coleman MM, Painter PC. 2005. The miscibility of hydrogen-bonded polymer blends: Two self-associating polymers. Macromolecules. 38:3703–3707.
- Putnam D, Kopeček J. 1995. Polymer conjugates with anticancer activity. Adv Poly Sci. 122:55–123.
- Qin CQ, Du YM, Xiao L, Li Z, Gao XH. 2002. Enzymic preparation of water-soluble chitosan and their antitumor activity Int J Biol Macromol. 31:111–117.
- Regelson W. 1967. Prevention and treatment of Friend leukemia virus (FLV) infection by interferon-inducing synthetic polyanions. The Reticuloendothelial System and Atherosclerosis, vol 1. New York: Springer, pp.315–332.
- Ringsdorf H. 1975. Structure and properties of pharmacologically active polymers. J Polym Sci Polym Sym. 51:135–153.
- Spridon D, Panaitescu L, Ursu D, Uglea CV, Popa I, Ottenbrite RM. 1997. Synthesis and biocompatibility of maleic anhydride copolymers: 1. Maleic anhydride–vinyl acetate, maleic anhydride–methyl methacrylate and maleic anhydride–styrene. Polym Inter. 43:175–181.
- Sun Z, Wang W, Feng Z. 1992. Criterion of polymer-polymer miscibility determined by viscometry. Eur Polym J. 28:1259–1261.
- Swamy TM. 2007. Studies on miscibility of polyacrylamide/polyethylene glycol blends. J Appl Polym Sci. 104:2048–2053.
- Tong R, Cheng J. 2007. Anticancer polymeric nanomedicines. J Macromol Sci Polym Rev. 47:345–381.
- Utracki LA. 2002.Polymer Blends Handbook, vol 1 London: Kluwer Academic Publishers.
- Yu WW, Chang E, Falkner JC, Zhang J, Al-Somali AM, Sayes CM, et al. 2007. Forming biocompatible and nonaggregated nanocrystals in water using amphiphilic polymers. J Am Chem Soc. 129:2871–2879.
- Zhong T, Deng C, Gao Y, Chen M, Zuo B. 2012. Studies of in situ- forming hydrogels by blending PLA-PEG-PLA copolymer with silk fibroin solution. J Biomed Mater Res. 100:1983–1989.