Abstract
In this study, we aimed to produce pH-sensitive microspheres for the controlled release of the nonsteroidal anti-inflammatory drug, ketorolac tromethamine (KT). For this purpose, an interpenetrating polymer network (IPN) of microspheres of poly(vinyl alcohol) (PVA)/sodium carboxymethyl cellulose (NaCMC) were prepared, based on different formulations using glutaraldehyde (GA) (0.66 M) and hydrochloric acid (HCl) (3%, v/v). The preparation conditions of the microspheres were optimized by considering the percentage of entrapment efficiency and swelling capacity of the microspheres, and their release data. The effects of PVA and NaCMC ratio on the release of KT for over a period of 6 h, at three pH values (1.2, 6.8, and 7.4), have been discussed.
Introduction
Natural carbohydrate polymers have been widely used in biomedical and pharmaceutical applications, such as the production of matrix materials (CitationRokhade et al. 2006, CitationAl-Kahtani and Sherigara 2014). In recent years, controlled release technology has focused on finding more biocompatible and nontoxic materials for medical, pharmaceutical, and agricultural applications. A search of the literature indicates that there are many research studies about controlled release using natural polymers (CitationShang et al. 2014, CitationKaity et al. 2013, CitationShu and Zhu 2002, CitationZhu and Zhuo 2001). However, natural polymers have some limitations, such as poor mechanical strength and uncontrolled water uptake (CitationAgnihotri and Aminabhavi 2005). Many studies have been reported in the literature, of efforts to overcome these disadvantages by chemical and physical alterations. Among these, the development of interpenetrating polymer network (IPN) structures has drawn attention (CitationBurugapalli et al. 2001, CitationChangez et al. 2004, CitationKosmala et al. 2000, CitationKurkuri and Aminabhavi 2004, CitationSrenivasan 1997).
Poly(vinyl alcohol) (PVA) is a well known polymer used in drug release studies, due to its desirable properties such as nontoxicity and noncarcinogenicity, and has been used in many studies because of its biocompatibility (CitationOrienti et al. 2002, CitationKim and Lee 1999, CitationKweon and Kang 1999, CitationAli et al. 2014). However, it is diffucult to prepare microspheres using this polymer, due to its poor stability and microsphere-forming ability in aqueous medium. Various researchers have studied the PVA-based IPN systems (CitationShu and Zhu 2002, CitationBurugapalli et al. 2001, CitationYue et al. 2009, CitationBanerjee et al. 2012, CitationKrishna Rao et al. 2008).
Sodium carboxymethyl cellulose (NaCMC) is a polysaccharide and a nontoxic and biodegradable polymer which is used in controlled release systems, and can be easily dissolved in aqueous solutions (CitationRokhade et al. 2006, CitationZhu et al. 2013, CitationConti et al. 2007, CitationCai et al. 2010, CitationMadhusudana Rao et al. 2012, CitationVaraprasad et al. 2011, CitationIşıklan 2006). Conti and co-workers used NaCMC and hydroxyprophylmethylcellulose (HPMC) as polymeric carriers to improve controlled release performances of matrix tablets containing a soluble drug (CitationConti et al. 2007). In vitro release studies have demonstrated that the mixture of the two cellulose derivatives enables a better control of the drug release profiles at pH levels of 4.5 and at 6.8, both in terms of rate and mechanism.
Ketorolac tromethamine () is a non-steroidal anti-inflammatory drug. The plasma half-life of KT has been reported to be around 4–6 h, and it is a relatively favorable therapeutic agent for the management of moderate to severe pain (CitationVarshosaz et al. 2011). However, gastrointestinal side effects, such as gastrointestinal bleeding and bleeding at the operative site, are commonly seen when this drug is used. The maximum duration of treatment should not exceed five days for tablets, or two days for continuous daily dosing with intravenous or intramuscular formulations. For this reason, it is important to obtain prolonged or controlled drug delivery, to improve bioavailability or stability and to target the drug to the specific site.
The present work describes the preparation and characterization of IPN microspheres for the controlled release of KT. The prepared IPN microspheres have been characterized by different techniques, to understand their drug release characteristics and chemical interactions.
Experimental
Materials
NaCMC was purchased from Sigma Chemical Co (Louis, USA). PVA was supplied by Merck (Darmstadt, Germany). The molecular weight and degree of saponification of PVA were 72000 and greater than 98%, respectively. KT was received from Sigma-Aldrich (Steinheim, Germany). Glutaraldehyde (GA) (25% w/w) solution, Na2HPO4, and NaH2PO4 were all supplied from Merck (Darmstadt, Germany), and were used as received. Span-85, light liquid paraffin, hexane, Na2HPO4, NaH2PO4, and CaCl2 were supplied by Merck (Darmstadt, Germany).
Preparation of IPN microspheres
IPN microspheres of PVA and NaCMC were prepared by the emulsion-crosslinking method. Briefly, PVA (8% w/w) and NaCMC (2% w/w) were dissolved in distilled water by stirring. The required amount of KT was dispersed in this solution. The drug-loaded polymer solution was emulsified into light liquid paraffin to form a water-in-oil (W/O) emulsion, using a high speed stirrer in a beaker containing light liquid paraffin oil. Span-85 (2% (w/v)) and GA were added slowly and further stirred for a predetermined number of minutes. The microspheres formed were filtered, and washed repeatedly with n-hexane and water to remove the oil. These microspheres were dried in an oven at 40°C, and stored for further analysis (CitationKondolot Solak 2011). The preparation conditions of KT-loaded microspheres are shown in . A schematic representation of the synthesis of IPN is given in .
Table I. Formulation codes, results of percentage entrapment efficiency, and yield of the microspheres.
Fourier transform infrared spectroscopy (FTIR)
FTIR spectra of the empty microspheres, KT-loaded microspheres, and polymer samples, were obtained using a Mattson 1000 FTIR spectrometer (Welwyn Garden, England).
Differential scanning electron microscopy (DSC)
The glass transition temperature (Tg) of the microspheres was determined using a Dupont General V4.1C 2000 differential scanning calorimeter (USA). The measurements were performed over the temperature range of 0°C–300°C, at the heating rate of 10°C/min.
Scanning electron microscope (SEM)
SEM photographs were taken using the Quanta 4000 F Field Emission scanning electron microscope, to examine the morphology and surface structure of the microspheres.
X-ray diffractometry (XRD)
The X-RD patterns of KT, KT-loaded microspheres, and empty microspheres were recorded using a Rigaku Ultima-IV model diffractometer.
Swelling studies
The equilibrium swelling degree of the crosslinked empty microspheres was determined by the gravimetric measurement of the extent of their swelling in buffer solutions with pH values of 1.2, 6.8, and 7.4, at 37°C. To ensure complete equilibration, the samples were allowed to swell for 48 h. The excess surface-adhesion of microspheres was removed by blotting. The swollen microspheres were weighed with an electronic balance (XB 220 A Precisa, United States). The microspheres were then dried in an oven at 40°C, until there was no change in the dried mass of the samples. The equilibrium swelling degree (%) was calculated as follows:
where Ms and Md are the mass of the swollen microspheres and dry microspheres, respectively.
Determination of drug content of the microspheres and their entrapment efficiency
A known mass of microspheres was placed in a flask, to which water (50 mL) was then added and refluxed at the ambient temperature for 2 h, to ensure the complete extraction of KT from the microspheres. At the end of the 2-h period, the precipitated polymer was filtered and KT was analyzed with a Unico 4802 ultraviolet-visible spectrophotometer at a wavelength of 323 nm, with water as a blank. The percentage of entrapment efficiency (% EE) was then calculated as follows:
In vitro release study
In vitro drug release from the microspheres was studied in a HCL solution of pH 1.2, and a phosphate buffer solution with pH levels of 6.8 and 7.4, and incubated in a shaking water bath (Medline BS-21, Korea) at 37°C. At 2-h intervals, the pH of the medium was changed to 1.2, 6.8 and 7.4, respectively. At specific time intervals, the KT content was determined using a UV spectrophotometer at 323 nm. After analysis, the solution was added back to the dissolution media, to maintain a constant volume. From the absorbance values, the cumulative percentage of the amount of release was determined.
Results and discussion
Characterization of microspheres
In this research, the KT-loaded IPN microspheres based on PVA and NaCMC were prepared and crosslinked with GA. The FTIR spectra of pristine PVA (a), pristine NaCMC (b) and drug-loaded NaCMC/PVA (c) IPN microspheres are shown in . For NaCMC, the bands between 3500 and 3400 cm− 1 show O–H stretching vibrations. Bands at 2923 and 2853 cm− 1 show aliphatic C–H stretching vibrations. The bands found at 1125 and 1036 cm− 1 represent C–O–C stretching vibrations. Asymmetric and symmetric stretching of the carboxylate group is observed at 1634 and 1410 cm− 1.
Figure 3. FTIR spectrum of (a) pristine PVA, (b) pristine NaCMC, and (c) KT-loaded NaCMC/PVA microspheres (drug/polymer ratio: 1/8 (w/w), polymer/polymer ratio: 1/1 (w/w)).
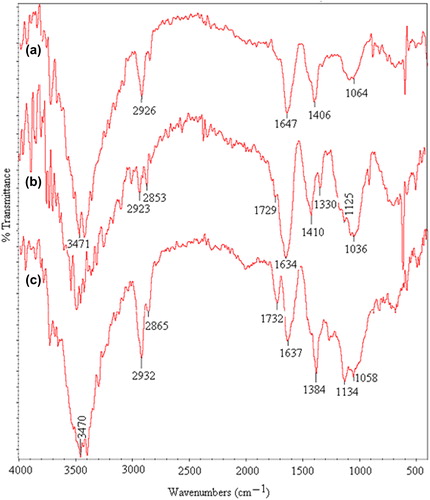
In the FTIR spectra of PVA, the bands at 3471 and 2926 cm− 1 indicate O–H and C–H stretching vibrations, respectively. The peaks that appeared for both PVA and NaCMC were observed in the spectra of the NaCMC/PVA microspheres.
The FTIR spectra of KT-loaded NaCMC/PVA microspheres (a), pristine KT (b), and placebo NaCMC/PVA microspheres (c), are shown in . For KT, a band at 3350 cm− 1 shows N–H and O–H stretching vibrations. Aliphatic C–H stretching is indicated by bands at 2926 and 2863 cm− 1, but, aliphatic C–H bending vibration is observed at 1384 cm− 1. Bands at 1592 and 1562 cm− 1 are due to carbonyl C = O stretching vibrations. A band at 898 cm− 1 indicates a mono substituted phenyl ring. In the spectra of KT-loaded microspheres, a peak at 1647 cm− 1 is due to the merging of peaks of KT observed at 1592 and 1562 cm− 1. These peaks are observed in the spectra of empty microspheres at 1637 cm− 1. This confirms the presence of KT in the KT-loaded microspheres.
Figure 4. FTIR spectrum of (a) KT-loaded microspheres (drug/polymer ratio: 1/8 (w/w), polymer/polymer ratio: 1/1 (w/w)), (b) pristine KT, and (c) placebo NaCMC/PVA microspheres (polymer/polymer ratio: 1/1 (w/w)).
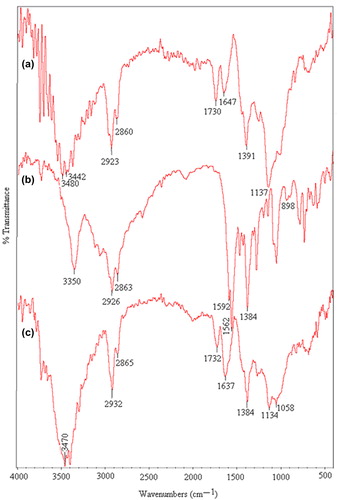
The DSC thermograms of pristine KT (a), KT-loaded NaCMC/PVA microspheres (b), and placebo NaCMC/PVA microspheres (c) are presented in . The thermogram of KT shows a sharp peak at 170°C, indicating the melting of the drug. In the case of KT-loaded microspheres, there are no peaks corresponding to KT. This indicates the amorphous dispersion of KT into the IPN matrix.
Figure 5. DSC thermograms of (a) pristine KT, (b) KT-loaded NaCMC/PVA microspheres (drug/polymer ratio: 1/8 (w/w), polymer/polymer ratio: 1/1 (w/w)), and (c) placebo NaCMC/PVA microspheres (polymer/polymer ratio: 1/1 (w/w)).
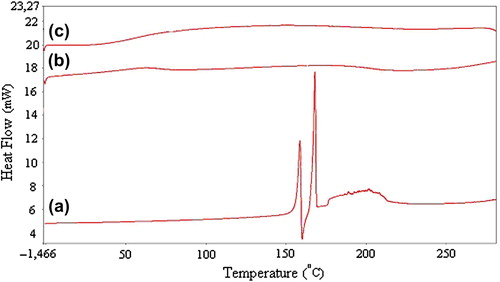
The SEM photographs of the IPN microspheres were taken at magnifications of 4000 and 8000, and are shown in and . It can be seen from the figures that the microspheres maintain a spherical form.
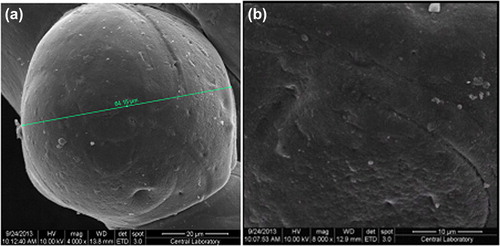
The X-ray difractograms of (a) KT-loaded IPN microspheres and (b) placebo IPN microspheres are presented in . X-RD studies are used to determine the crystallinity of the drug in the crosslinked microspheres. KT has shown the characteristic intense peaks between 2θ of 180 and 200 due to its crystalline nature (CitationRokhade et al. 2006). These peaks have disappeared in the results for the placebo and KT-loaded microspheres. In this study, the characteristic peaks of KT overlapped with the noise of the coated polymer itself. Further, the loaded drug is amorphous, which is difficult to measure at the detection limit of the crystal size in this case. This confirms that the drug is molecularly dispersed at a molecular level in the polymer matrix, and hence, no crystals are found in the drug-loaded microspheres.
Many factors, such as exposure time to crosslinking agent and the amounts of the drug and polymer, affect the % EE and yield. The results of % EE and microsphere yield (%) are shown in . As can be seen from the table, the % EE was slightly affected with the decrease in drug/polymer ratio. However by increasing the amount of NaCMC, a slight decrease in % EE is observed. By increasing the exposure time to the crosslinking agent, the % EE increased, due to the formation of a more rigid network structure at a higher amount of crosslinking.
In vitro drug release
The in vitro release of KT from the IPN microspheres is studied in gastric, input intestinal and intestinal pH conditions at 37°C. The effects of the amounts of NaCMC and PVA on the KT release are presented in . It is seen from the figure that the cumulative percentage of KT released from microspheres is increased with an increase in the NaCMC content. The results can be explained by the fact that as the NaCMC content in the polymer matrix increases, swelling of the matrix increases, due to the hydroplihic nature of NaCMC. The maximum cumulative KT release is obtained in formulation A1, as 99.6%, at the end of 6 h, with a drug/polymer ratio of 1/8. Madhusudana Rao et al. studied the preparation and characterization of pH-sensitive poly(vinyl alcohol)/sodium carboxymethyl cellulose IPN microspheres for the in vitro release studies of an anti-cancer drug (CitationMadhusudana Rao et al. 2012). They found that the percentage of cumulative release is higher at higher amounts of NaCMC present in the matrix. This can also be attributed to the fact that as the NaCMC content in the polymer matrix increases, swelling of the matrix also increases, due to the extremely hydrophilic nature of NaCMC.
Figure 8. Effect of the NaCMC/PVA ratio on KT release (*):1/1, (x):1/2, (▲):1/4, (■):1/6, (♦):1/8, (drug/polymer ratio:1/8, concentration of GA:0.66 M, exposure time to GA:30 min.).
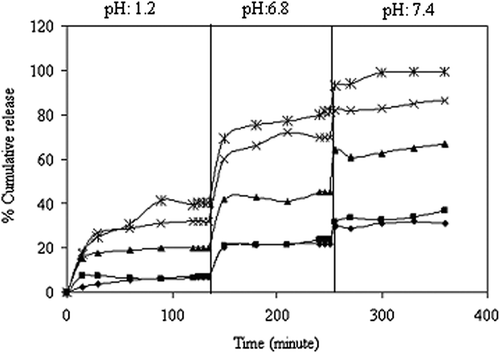
The other parameter that affects the KT release from the microspheres is the drug/polymer ratio. The effect of drug/polymer ratio on KT release is shown in . The figure shows that the cumulative release of KT from the IPN increases with a decrease in the KT/polymer ratio from 1/1 to 1/8. Lower KT content might lead to the easier penetration of liquid through the microspheres, and faster KT diffusion then occurs from the microspheres. In addition, while the KT content of the microspheres decreases, a loose structure is formed in the polymeric microspheres, through which the liquid and KT can easily penetrate and diffuse. Similar results were found in the literature (Şanlı and Kondolot Solak 2006, CitationKumbar and Aminabhavi 2003). CitationŞanlı and Kondolot Solak (2009) investigated controlled release of naproxen from sodium alginate and beads of a poly (vinyl alcohol)/sodium alginate blend, crosslinked with glutaraldehyde. They reported that the cumulative release of naproxen increases with the decrease in the naproxen/polymer ratio from 1/1 to1/4.
Figure 9. Effect of the drug/polymer ratio on KT release (*):1/8, (x):1/6, (▲):1/4, (■):1/2, (♦):1/1, (NaCMC/PVA ratio:1/1, concentration of GA:0.66 M, exposure time to GA:30 min.).
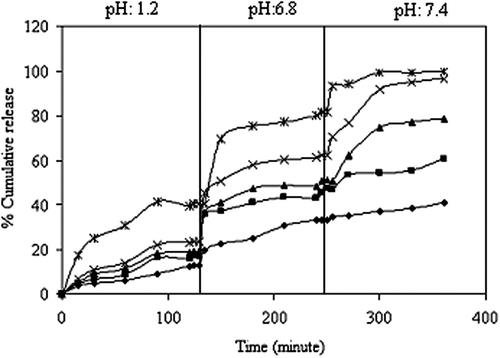
One of the most effective ways to change the release pattern of microspheres is to change the crosslinking density of the matrix by varying the times of exposure to crosslinking agent. The effect of the duration of exposure to GA on the release rate of KT has been investigated by varying the duration of exposure to GA to 30 and 45 min, respectively. The results are given in , which clearly indicates that with increasing time of exposure to GA (30–45 min), the cumulative release decreases. Due to the fact that increasing the duration of exposure to GA results in an increase in the crosslinking density of the microspheres, which gives rise to a compact network of macromolecular chains, the release becomes slower at a higher amount of GA, as expected, but becomes faster at the lower amount of GA. The maximum KT release from the 1/1 NaCMC/PVA microspheres, which are prepared with an exposure time of 30 min, is found to be 99.6%. Similar results have been reported by many workers (CitationIşıklan 2006, CitationŞanlı and Kondolot Solak 2009, CitationSoppimath and Aminabhavi 2002, CitationKumbar and Aminabhavi 2002, CitationKulkarni et al. 2000, CitationBajpai and Rajpoot 2001). Işıklan studied the controlled release of the insecticide carbaryl from beads of sodium alginate, sodium alginate/gelatin and sodium alginate/sodium carboxymethyl cellulose blends, crosslinked with glutaraldehyde. The author reported that by increasing the time of exposure to glutaraldehyde (5–15 min), the release rate of carbaryl decreases (CitationIşıklan 2006).
Figure 10. Effect of the exposure time on KT release (♦): 30 min., (■): 45 min. (NaCMC/PVA ratio: 1/1, drug/polymer ratio:1/8, concentration of GA:0.66 M).
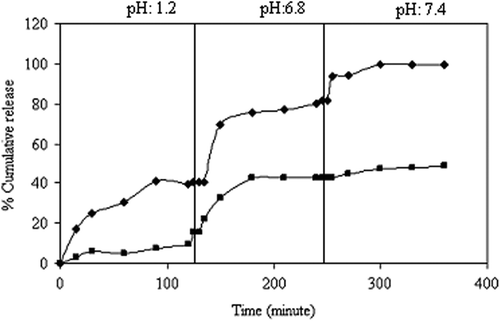
KT-release results are also supported by the measurements of swelling. Equilibrium swelling experiments are performed in distilled water and in buffer solutions of different pH values (pH of 1.2, 6.8 and 7.4) for various empty microsphere formulations, and are presented in . As it seen from the , a decrease in pH values decreases the percentage of swelling.
Table II. Results of swelling study of empty IPN microspheres.
Table III. Results of k, n and r calculated with equation (3).
Analysis of the kinetic results
The event of solvent sorption by a microsphere depends mechanistically on the diffusion of water molecules into the gel matrix and subsequent relaxation of the macromoleculer chains of the microsphere (CitationBajpai and Sharma 2005). The release data of all the systems have been further substantiated by fitting the fraction release data Mt/M∞ to an empirical equation proposed by CitationPeppas (1985).
where Mt is the amount of NS released at time t and M∞ is the drug released at equilibrium time; k, a constant characteristic of the drug-polymer system; and n, the diffusional exponent which suggests the nature of the release mechanism. Fickian release is defined by initial t1/2 time dependence of the fractional release for slabs, cylinders and spheres. Analogously, Case-II transport is defined by an initial linear time dependence of the fractional release for all geometries (CitationRitger and Peppas 1987). A value of n equal to 0.5 indicates the Fickian transport (mechanism), while if the value of n is 1, it is an example of Case II or non-Fickian transport (swelling controlled) (CitationRamesh Babu et al. 2006). The intermediary values ranging between 0.5 and 1.0 are indicative of anomalous transport. The least squares estimations of the fractional release data, along with the estimated correlation coefficient values, r, are presented in . From these data, the n value was found to range from 0.059 to 0.178, indicating that the release of KT from the microspheres slightly deviates from the model of Fickian transport.
Conclusions
The biocompatible and naturally available polymers NaCMC and PVA were used to prepare IPN microspheres for the controlled release of KT. FTIR, DSC, SEM and XRD have been used to confirm the formation of IPN. The release studies have been supported with swelling studies. The KT release from IPN microspheres increases with a decrease in drug/polymer ratio. On the other hand, drug release decreases as the amount of NaCMC increases. As the duration of exposure to crosslinking agent increases, the release rate of KT decreases, whereas the entrapment efficiency increases. It is also observed that release of KT is much higher at high pH values,compared to low pH values, showing that the release system is interesting as a controlled release system for colon-specific drug delivery. The highest KT release has been found to be 99.6%, for the NaCMC/PVA ratio of 1/1 and a drug/polymer ratio of 1/8. The swelling measurements of the microspheres supported the release results. The release mechanism was found to follow a Fickian-type behavior.
Acknowledgement
The authors are grateful to the Gazi University Scientific Research Foundation for support of this study.
Declaration of interest
The authors report no declarations of interest. The authors alone are responsible for the content and writing of the paper.
References
- Agnihotri SA, Aminabhavi TM. 2005. Development of novel interpenetrating network gellan gum-poly (vinyl alcohol) hydrogel microspheres for the controlled release of carvedilol. Drug Dev Ind Pharm. 31:491–503.
- Ali L, Ahmad M, Usman M, Yousuf M. 2014. Controlled release of highly water-soluble antidepressant from hybrid copolymer poşy vinyl alcohol hydrogels. Polym Bull. 71:31–46.
- Al-Kahtani AA, Sherigara BS. 2014. Semi-interpenetrating network of acrylamide-grafted-sodium alginate microspheres for controlled release of diclofenac sodium, preparation and characterization. Colloids Surf B Biointerfaces. 115:132–138.
- Bajpai AK, Rajpoot M. 2001. Release and diffusion of sulfamethoxazole through acrylamide-based hydrogel. J Appl Polym Sci. 81: 1238–1247.
- Bajpai AK, Sharma M. 2005. Preparation and characterization of binary grafted polymeric blends of poly(vinyl alcohol) and gelatin and evaluation of their water uptake potential. J Macromol Sci Pure Appl Chem. 42:663–682.
- Banerjee S, Sidiqui L, Bhattacharya SS, Kaity S, Ghosh A, Chattopadhyay P, et al. 2012. Interpenetrating polymer network (IPN) hydrogel microspheres for oral controlled release application. Int J Biol Macromols. 50:198–206.
- Burugapalli K, Bhatia D, Koul V, Choudhary V. 2001. Interpenetrating polymer networks based on poly (acrylic acid) and gelatin: swelling and thermal behavior. J Appl Polym Sci. 82:217–227.
- Cai X, Luan Y, Dong Q, Shao W, Li Z, Zhao Z. 2010. Sustained release of 5-fluorouracil by incorporation into sodium carboxymethylcellulose sub-micron fibers. Int J Pharmaceut. 419:240–246.
- Changez M, Koul V, Burugapalli K, Dinda AK. 2004. Studies on biodegradation and release of gentamicin sulphate from interpenetrating network hydrogels based on poly (acrylic acid) and gelatin: In vitro and in vivo. Biomaterials. 25:139–146.
- Conti S, Maggi L, Segale L, Ochoa Machista E, Conte U, Grenier P, Vergnault G. 2007. Matrices containing NaCMC and HPMC: 1. Dissolution performance characterization. Int J Pharmaceut. 333:136–142.
- Işıklan N. 2006. Controlled release of insectiside carbaryl from sodium alginate, sodium alginate/sodium carboxymethylcellulose blend beads crosslinked with glutaraldehyde. J Appl Polym Sci. 99: 1310–1319.
- Kaity S, Isaac J, Ghosh A. 2013. Interpenetrating polymer network of locoust bean gum-poly (vinyl alcohol) for controlled release drug delivery. Carbohydr Polym. 94:456–467.
- Kim SY, Lee YM. 1999. Drug release behavior of electrical responsive poly(vinyl alcohol)/poly(acrylic acid) IPN hydrogels under an electric stimulus. J Appl Polym Sci. 74:1752–1761.
- Kondolot Solak E. 2011. Preparation and characterization of IPN microspheres for controlled delivery of naproxen. J Biomater Nanobiotech. 2:445–453.
- Kosmala JD, Henthorn DB, Peppas LM. 2000. Preparation of interpenetrating networks of gelatin and dextran as degradable biomaterials. Biomaterials. 21:2019–2023.
- Krishna Rao KSV, Kiran Kumar ABV, Madhusudhan Rao K, Subha MCS, Lee Y III. 2008. Semi-IPN hydrogels based on Poly(vinyl alcohol) for controlled release studies of chemotherapeutic agent and their swelling characteristics. Polym Bull. 61:81–90.
- Kulkarni AR, Soppimath KS, Aminabhavi TM, Dave AM, Mehta MH. 2000. Glutaraldehyde crosslinked sodium alginate beads containing liquid pestiside for soil application. J Control Release. 63:97–105.
- Kumbar SG, Aminabhavi TM. 2002. Preparation and characterization of interpenetrating network beads of poly(vinyl alcohol)-grafted-poly(acrylamide) with sodium alginate and their controlled release characteristics for cypermethrin pesticide. J Appl Polym Sci. 84: 552–560.
- Kumbar SG, Aminabhavi TM. 2003. Synthesis and characterization of modified chitosan microspheres: Effect of the grafting ratio on the controlled release of nifedipine through microspheres. J Appl Polym Sci. 89:2940–2949.
- Kurkuri MD, Aminabhavi TM. 2004. Poly (vinyl alcohol) and poly (acrylic acid) sequential interpenetrating network pH sensitive microspheres for the delivery of diclofenac sodium to the intestine. J Control Release. 96:9–20.
- Kweon DK, Kang DW. 1999. Drug –release behavior of chitosan- g-poly(vinyl alcohol) copolymer matrix. J Appl Polym Sci. 74:458–464.
- Madhusudana Rao K, Mallikarjuna B, Krishna Rao KSV, Prabhabkar MN, Chowdoji Rao K, Subha MCS. 2012. Preparation and characterization of pH sensitive poly(vinyl alcohol)/sodium carboxymethyl cellulose IPN microspheres for in vitro release studies of an anti-cancer drug. Polym Bull. 68:1905–1919.
- Orienti I, Trere R, Luppi B, Bigucci F, Cerchiara T, Zuccari G, Zecchi V. 2002. Hydrogels formed by crosslinked poly(vinyl alcohol) as sustained drug delivery systems. Arc Pharm. 335:89–93.
- Peppas NA. 1985. Analysis of Fickian and non-Fickian drug release from polymer. Pharm Acta Helv. 60:110–111.
- Ramesh Babu V, Krishna Rao KSV, Sairam M, Vijaya Kumar Naidu B, Hosamani KM, Aminabhavi TM. 2006. pH-Sensitive interpenetrating network microgels of sodium alginate-acrylic acid for the controlled release of ibuprofen. J Appl Polym Sci. 99:2671–2678.
- Ritger PL, Peppas NA. 1987. A simple equation for description of solute release II Fickian and anomalous release from swellable devices. J Control Release. 5:37–42.
- Rokhade AP, Agnihotri SA, Patil SA, Mallikarjuna NN, Kulkarni PV, Aminabhavi TM. 2006. Semi-interpenetrating polymer network microspheres of gelatin and sodium carboxymethyl cellulose for controlled release of ketorolac tromethamine. Carbohydr Polym. 65:243–252.
- Shang Y, Ding F, Xiao L, Deng H, Du Y, Shi X. 2014. Chitin-based fast responsive pH sensitive microspheres for controlled drug release. Carbohydr Polym. 102:413–418.
- Shu XZ, Zhu K. 2002. The release behavior of brilliant blue from calcium–alginate gel beads coated by chitosan: the preparation method effect. Eur J Pharm Biopharm. 53:193–201.
- Soppimath KS, Aminabhavi TM. 2002. Water transport and drug release study from cross-linked polyacrylamide grafted guar gum hydrogel microspheres for the controlled release application. Eur J Pharm Biopharm 53:87–98.
- Srenivasan K. 1997. On the restriction of the release of water-soluble component from polyvinyl alcohol film by blending β-cyclodextrin. J Appl Polym Sci. 65:1829–1832.
- Şanlı O, Kondolot Solak E. 2009. Controlled release of naproxen from sodium alginate and poly (vinyl alcohol)/sodium alginate blend beads crosslinked with glutaraldehyde. J Appl Polym Sci. 112:2057–2065.
- Varaprasad K, Vimila K, Ravindra S, Reddy NN, Raju KM. 2011. Sodium carboxymethyl cellulose-based poly (acrylamide-co-2 acrylamido-2-methyl-1-propane sulfonic acid) hydrogels for in vitro drug release studies of ranitidine hydrochloride an anti-ulcer drug. Polym-Plast Technol Eng. 50:1199–1207.
- Varshosaz J, Hajhashemi V, Soltanzadeh S. 2011. Lipid nanocapsule-based gels for enhancement of transdermal delivery of ketorolac tromethamine. J Drug Deliv. 571272:7.
- Yue Y, Shang X, Wang P. 2009. Fabrication and characterization of microstructured and pH sensitive interpenetrating networks hydrogel films and application in drug delivery field. Eur Polym J. 45:309–315.
- Zhu K, Ye T, Liu J, Peng Z, Xu S, Lei J, et al. 2013. Nanogels fabricated by lysozyme and sodium carboxymethyl cellulose for 5-fluorouracil controlled release. Int J Pharmaceut. 441:721–727.
- Zhu Z, Zhuo R. 2001. Slow release behavior of starch-g-poly(vinyl alcohol) matrix for 2,4,5-trichlorophenoxyacetic acid herbicide. Eur Polym J. 37:1913–1919.