Abstract
Quantum dots (QDs) as colloidal nanocrystalline semiconductors have exceptional photophysical properties, due to their quantum confinement effects. Depending on their sizes and chemical compositions, QDs emit different wavelengths over a broad range of the light spectrum, from visible to infrared. QDs are typically extensively used for optical applications due to their high extinction coefficient. This article reviews biomedical applications of QDs, especially the application of QDs in cell targeting, delivery, diagnostics, cancer therapy, and imaging for cancer research.
Introduction
Semiconductor nanocrystals composed of groups II–VI or III–V elements, known as quantum dots (QDs), are too small to exhibit quantum mechanical properties. QDs of elements in Groups III–V may supply a more stable alternative to QDs of elements in groups II–VI, due to the presence of a covalent bond, rather than an ionic bond, and have been reported to have lower cytotoxicity (CitationBharali et al. 2005). Alexei Ekimov discovered the QD in a glass matrix, and it was discovered in colloidal solutions by Louis E. Brus. The word “quantum dot” was coined by Mark Reed (CitationTokumasu et al. 2005). In contrast to quantum dots of type-I, which simultaneously entrap electrons and holes, blank dots of type-II exert a pull on the charge transfer of one type and repel the other (CitationJacak et al. 1998). QDs are mostly considered for optical applications, because of their high extinction coefficient (CitationLeatherdale et al. 2002). The ability to tune the size of QDs is beneficial for numerous applications, such as larger quantum dots that have a larger spectrum-shift towards red compared to the smaller dots, and reveal less marked quantum properties. On the other hand, the smaller particles offer the benefit of extra subtle quantum effects. Until recently, diverse kinds of organic dyes were being used for biological analysis. To this end, QDs have quickly filled in the role, being found to be better than traditional organic dyes on a number of counts, one of the highest benefits immediately evident being their brightness as well as their stability (CitationMichalet et al. 2005). The surface modification of QDs with aptamers, antibodies, peptides, or undersized molecules that combine with antigens present on the target cells or tissues has resulted in the improvement of sensitive and specific targeted imaging and diagnostic modalities for in vitro and in vivo applications (CitationMedintz et al. 2003, CitationChu et al. 2006, CitationYoung and Rozengurt 2006). Current research studies have shown that these nanomaterials have magnitudes that are two-photon action cross sections larger than those of the usual fluorescent probes currently in use, plus more for deep tissue imaging (CitationLarson et al. 2003).
Emission, high levels of brightness, and photo stability. QDs can be used in vitro and in vivo for multiple color imaging and targeted drug delivery. Biomedical applications necessitate high-quality water-soluble QDs; although they could be made directly in water, they mostly have thin accessible size ranges and wide size distribution (CitationWang et al. 2004, CitationZhuang et al. 2003, CitationKho et al. 2000, CitationWinter et al. 2001). Therefore, the challenge lies in creating high-quality hydrophobic QDs that are soluble in water, and furthermore, QDs that are active in bioconjugate reactions. To solve this problem, the QDs synthesized in organic solvents usually have hydrophobic surface ligands, for instance, trioctylphosphine oxide, trioctylphosphine (CitationMurray et al. 1993, CitationPeng et al. 1998, CitationYu et al. 2003), and tetradecylphosphonic acid (CitationYu et al. 2003, CitationPeng et al. 2000, CitationPeng and Peng 2001). These hydrophobic ligands could be replaced by some water-soluble bifunctional molecules in which one side connects to the surface atoms of the QDs, and the other side is hydrophilic and may also be reactive to biomolecules () (CitationAldana et al. 2001, CitationChan and Nie 1998, CitationWuister et al. 2003).
The photophysical properties of QDs, such as brightness, spectral range, long lifetimes, and their tendency to function as single-molecule probes may give good reason for the development of novel detectors that would have the temporal resolution and sensitivity of avalanche photodiodes and the 2D spatial resolution of cameras, for wide-field in vivo studies of protein dynamics and trafficking (CitationMokari et al. 2004) ().
Metabolism and toxicity
QDs are versatile new nanoparticles for in vivo biomedical application. It is important to characterize their activities in vivo, rather than rely on ex vivo measurements and theoretical considerations alone (CitationChoi et al. 2009). One obstacle to the in vivo study of QDs is the nonspecific uptake by the reticuloendothelial system (RES) including the liver, spleen, and lymph system. Particle size, surface coating and PEGgylation influence the biodistribution of QDs. The in vivo distribution and metabolism of QDs have been studied in some research studies, which have shown that QDs are generally localized in liver, kidney, spleen, and lung (CitationChoi et al. 2007, CitationInoue et al. 2007, CitationKarabanovas et al. 2008, CitationLiu et al. 2007, CitationHardman 2006). However, has been no universal conclusion about the pathway of QD clearance and its influencing factors. All engineered QDs cannot be considered to be of the same group of materials. The absorption, metabolism, distribution, excretion, and toxicity of QDs depend on manifold factors ensuing from both inherent physicochemical properties and environmental conditions; the size, charge, concentration, bioactivity of the outer coating, oxidative, photolytic, and mechanical constancy of QDs have each been identified as determining parameters in QD toxicity. Even though they propose potentially significant societal profits, for example in drug targeting and in vivo biomedical imaging, QDs may also pose risks to human health and the environment under certain situations (CitationMishra et al. 2012).
Epitaxy
The controlled growth of a single crystalline material on top of a substrate is called epitaxy, which is a very essential process in semiconductor technology (CitationBen-Ari 2003). QDs are really formed when very slim semiconductor films buckle due to the stress of having a lattice structure slightly different in size from those on which the films are grown. Self-assembled dots are then used to make quantum dot lasers (CitationDerfus et al. 2004).
Application
When QDs were first created in the early 1980s, researchers forecasted their use in optics, electronics, and computing. Although the primary sensible applications of these small bits of semiconductor may really occur in biology and medicine (CitationQi and Gao 2008), the potential applications of QDs in biology and medicine were limited due to the toxic effects of semiconductor QDs, which have received enormous attention over the past few years (CitationZhang et al. 2011).
Intended for biological applications, QDs must be linked to biomolecules without changing the biological activity of the conjugated structure. A type of successful conjugation technique has been developed, including covalent and noncovalent attachment methodologies. Particular conjugation methodologies include direct adsorption on the surface of the QD, the use of inert polymer coatings, or biotin-streptavidin linkages. Present biomedical applications of QDs are paying attention to molecular imaging and sensing due to the above-mentioned optical properties. The structural properties of QDs are maybe as important as the optical properties, as it has been realized in research on drug delivery (CitationZhang et al. 2011, CitationGregor 2010). In addition, with the development of new techniques and detection methods, QDs are shown to have applications in wider fields. Zhang et al. successfully introduced Kelvin force microscopy (KFM) as a method to examine the binding of QDs with DNA, both in vitro and in vivo (CitationGregor 2010) ().
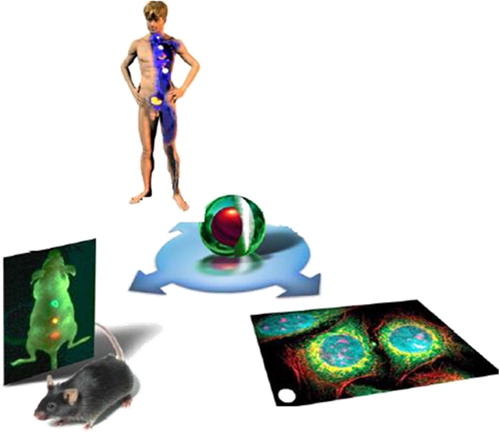
Biological imaging
The purpose of molecular imaging is to create image contrast due to the molecular difference in diverse tissues and organs. It shows the fluorescence images from Vero cells with the inclusion of silicon quantum dots transfected into the cytosol. The fluorescence illuminating the cells comes from direct band gap emission from the silicon quantum dots in Vero cells, illustrating the use of hydrophilic silicon QDs as biological fluorescence imaging agents (CitationWeng et al. 2008). QDs are extensively used as in vivo imaging agents (CitationSmith et al. 2008, CitationChoi et al. 2010, CitationPapagiannaros et al. 2010, CitationHan et al. 2001). In the treatment of cancer, a particular antibody coupled to near-IR QDs with a polymer coating is a very popular QD agent for tumor-targeted imaging (CitationSmith et al. 2008). Quantum dots have also been extensively used as in vitro imaging agents. By reducing the quantity of Cd released in the region of cells, scientists have been trying to create in vitro and in vivo testing methods for imaging nanoscale and microscale structures. The high resolution within the nanometer scale demonstrates behavior that is not beneficial for imaging DNA behavior for both biological engineering feedback and biological and chemical observation and analysis. The ability to control the emission spectra by changing the size of the QDs allows researchers to code many diverse targets by color (CitationGerion et al. 2001, CitationLi et al. 2009, CitationHyun et al. 2007).
Early studies of in vitro imaging used QDs to label cells. For instance, PbS and PbSe, capped with carboxylic groups, had been achieved in aqueous solution by replacing the ligands of label cells (CitationGac et al. 2006). It has been discovered that the cells can amass QDs in vesicles through endocytosis after washing away the overload QDs. Additionally, scientists have also verified the potential application of QDs in cell tracking by using avidin-conjugated QDs to tag cells (CitationTilley 2008). Silicon nanoparticles can be synthesized with a controlled size, in micelles and with surfaces that are defined from reactions with C = C compounds. The particles emit light in the blue region of the visible spectrum and are suitable as fluorescent markers in cell imaging. Additional research in the field of nanosized silicon is required, for both the design of synthetic protocols and to gain an improved understanding of the fundamental physics involved (CitationContag and Bachmann 2002). Self-illuminating quantum dots (QDBRET conjugates) are a group of new QDs that do not require external excitation light to fluoresce. Instead, energy comes from a bioluminescent protein through non-radiative energy transfer from the nearest bioluminescent proteins. Bioluminescence resonance energy transfer (BRET) is similar to FRET (Fluorescence Resonance Energy Transfer), except that the energy comes from a chemical reaction catalyzed by the donor enzyme rather than from absorption of excitation photons. Compared to fluorescence imaging, bioluminescence has tremendously high sensitivity for in vivo imaging purposes (CitationXing et al. 2008) ().
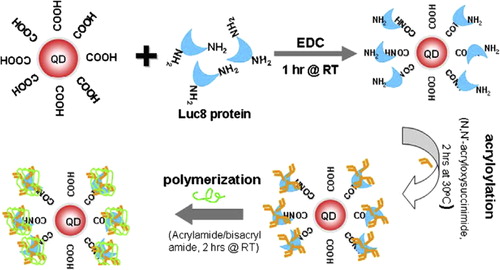
Gene technology
Gene therapy is a marvelous method to complement a deficient gene function. Even if there has been some success with the delivery of particular genes using diverse methods, such as the use of liposomes and viral vectors, most of these methods have a limited yield and also carry a risk of oncogenesis (CitationChen et al. 2006). The development of a safe and effective nonviral gene vector is a considerable challenge in the field of gene therapy. QDs have emerged as efficient FRET donors due to their wide absorption, narrow emission spectra, and high quantum yield, thereby minimizing cross-talk between the donor and acceptor (CitationPathak et al. 2001). QD-conjugated oligonucleotide sequences (attached via surface carboxylic acid groups) may be targeted to bind with DNA or mRNA (CitationGerion et al. 2002, CitationChan et al. 2005). QDs have been found to be considerably better than present methods in the cessation of gene activity. Observations show that a cell's production of a test protein dropped to 2 percent when siRNA was delivered with QDs. By contrast, the test protein was produced at 13 percent to 51 percent of standard levels when the siRNA was delivered with one of three prevalent reagents, or reaction-causing substances, which are now typically used in laboratories. In addition to their role in DNA technology, QDs perhaps find use in RNA technologies, in the detection of mRNA molecules using in situ hybridization (ISH), and in combining with siRNA in RNA intervention applications. QDs have been successfully used in the ISH method to study the expression of definite mRNA transcripts in mouse midbrain sections (CitationChoi et al. 2007).
Quantum dots as tags for drug carriers
The research of various drug nanocarriers is an imperative part of the progress of nanomedicine. The mechanism of delivery of QD/drug formulations to tumor cells is determined by the structure and properties of the nanomaterials (CitationZhang et al. 2011). The structural properties of QDs, which are perhaps equally as important, have just been realized in research on drug delivery. First, the size of QDs can be continuously tuned, from 2–10 nm, and particles smaller than 5 nm are rapidly cleared by renal filtration, Second, polymer encapsulation in general raises the size to 5–20 nm in diameter (CitationAzzazy et al. 2007). Recently, Xu et al. (2003) defined a novel process for high-throughput and multiplexed SNP (Single Nucleotide Polymorphisms) genotyping for using the Qbead system that uses quantum dots to encode microspheres employed as a platform for multiplexed assays. By mixing blends of QDs with separate emission wavelengths and intensities, exclusive spectral barcodes are formed that enable the high levels of multiplexing necessary for complex genetic analyses (CitationTabatabaei Mirakabad et al. 2014, CitationDavaran et al. 2014, CitationKouhi et al. 2014, CitationMohs et al. 2009, CitationSze 1985, CitationEbrahimnezhad et al. 2013, CitationPourhassan-Moghaddam et al. 2013, CitationAhmadi et al. 2014). The second group of QD application in traceable drug delivery is more straightforward – tagging a conventional medicine carrier with QDs, which serve as photostable fluorescent reporters. A novel approach to the enhancement of biocompatible nanoformulations that can target and treat human diseases involves the utilization of functionalized nanoparticles engineered to deliver drugs to the preferred tissues or organs (CitationMichalet et al. 2005, CitationModani et al. 2013). QDs have been tagged as both organic and inorganic drug carriers and potentially even bacteria and viruses have been used, with an explosion of activity in the field of ODN and siRNA delivery (CitationBentolila et al. 2009).
Anticancer applications
QDs, or fluorescent semiconductor nanocrystals, which are clusters of a few hundred to a few thousand atoms that emit light in rainbow hues, are among the nanomaterials in the spotlight for cancer therapy. One of the real challenges in cancer is diagnosing the state of a tumor and the potential for therapeutic treatment of that tumor. Near infrared QDs are detected by good tissue penetration and lower background, which are appropriate for the analysis of lymph node metastasis (CitationYong et al. 2009, CitationBallou et al. 2007, CitationFang et al. 2012). It has been demonstrated that the QDs injected into two model tumors rapidly move around to sentinel lymph nodes. In one study, scientists observed that four rhesus monkeys injected with cadmium-selenide QDs remained in normal health for over 90 days. Blood and biochemical parameters remained within normal ranges, and the major organs developed no abnormalities. The animals didn't lose weight. Bruchez and others expect to use the sensitivity and multiplexing facility of QDs for in vitro revealing of multiple protein or nucleic acid tumor cell markers that are changed at various stages of cancer (CitationQi and Gao 2008).
There are two methods by which QDs locate and mark tumor cells. In active targeting, QDs can be adjoining tumor-specific active binding sites so as to join themselves to tumor cells. Consequently, immunofluorescent probes are contrived with antibodies to identify these tumors (CitationDey and Rao 2011, CitationAbbasi et al. 2014, CitationEatemadi et al. 2014, CitationAlizadeh et al. 2014, CitationAbbasi et al. 2014, CitationEbrahimi et al. 2014). A QD system can discover the existence of particles of the RSV (Respiratory Syncytial Virus) in a matter of hours. It is, in addition, extra sensitive, allowing detection of the virus earlier in the route of a disease (CitationAbbasi et al. 2014, CitationDaraee et al. 2014, CitationTabatabaei Mirakabad et al. 2014, CitationDaraee et al. 2014, CitationNasrabadi et al. 2014, CitationChung et al. 2014, CitationFekri Aval et al. 2014, CitationValizadeh et al. 2014, CitationRahimzadeh et al. 2014, CitationEbrahimi et al. 2014, Citationde Lang 2012).
Quantum dots and neuroscience
QDs are seen as a novel tool of remarkable possibilities in neuroscience investigations. These nano sized materials are useful for experiments that are controlled by the limited anatomy of neuronal and glial interactions, for instance the small size of the synaptic cleft, or between an astrocyte and a neuron. In one unique linkage of quantum physics and neuroscience, QDs have been used to control brain cells, for the first time. Taking control of the brain could one day provide a non-invasive treatment for situations like depression, Alzheimer's, and epilepsy. In the near term, QDs can be used to treat blindness by activating damaged retinal cells (CitationLakowicz 1999) ().
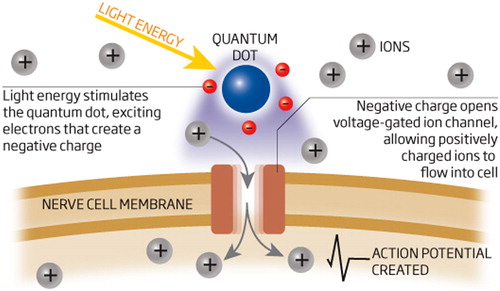
Biosensing and energy transfer
Quantum dots as strong fluorophores are companionable with ordinary biosensing techniques that apply fluorescence to generate a large measurable signal. In one research study, Medintz and coworkers used a related approach to expand a prototype FRET-based Quantum dot biosensor capable of detecting the nutrient sugar maltose in solution. The maltose-binding protein (MBP), pre-bound to an analog sugar-dye complex, was attached to water-soluble QDs, resulting in numerous MBPs linked to every QD (CitationMedintz et al. 2003, CitationAl-Ahmadi 2012).
QD blends for biological coding
The barcodes and the related readers are the most major technologies used for object detection. Since the barcode requires space to arrange the ordered data either in a 1D bar sequence or a 2D image, and therefore the barcode reader has to scan the 1D bar-sequence or register the 2D image, the systems for information retrieval are bulky and complicated (CitationResch-Genger et al. 2008).
Biomedicine is a research field where most significant and innovative advances have been carried out through the last decades. One of the most important activities in this area, which should be studied, is the large number of proteins and nucleic acids. Hence, the expansion of a fast and efficient coding method for biological molecules, which could allow high throughput analysis, would be desirable (CitationBruchez et al. 1998). Compared with the traditional organic dyes, the fluorescence properties of QDs offer unique photochemical and photophysical properties. In addition, they show longer photostability and lower photobleaching (CitationDavaran et al. 2013, CitationGhasemali et al. 2013).
Conclusion
The exceptional performance characteristics of Quantum dots (QDs), such as stability against photobleaching, high fluorescence yields, and the size-dependent luminescence features, give wide a variety of possibilities for their application in many fields. The ability to tune the size of QDs is favorable for numerous applications. Combined together, the large surface area of QDs is useful to covalently bond to biorecognition molecules, such as antibodies, peptides, nucleic acids, or small-molecule ligands, for further application as fluorescent probes.
Authors’ contributions
AA conceived of the study and participated in its design and coordination. EA and MM participated in the sequence alignment and drafted the manuscript. All authors read and approved the final manuscript.
Acknowledgments
The authors thank the Department of Medical Nanotechnology, Faculty of Advanced Medical Science of Tabriz University, for all support provided. This work is funded by a 2014 grant by the Drug Applied Research Center, Tabriz University of Medical Sciences.
Declaration of interest
The authors report no declaration of interest. The authors alone are responsible for the content and writing of the paper.
References
- Abbasi E, Akbarzadeh A, Kouhi M, Milani M. 2014. Graphene: synthesis, bio-applications, and properties. Artif Cells Nanomed Biotechnol. 1–7.
- Abbasi E, Milani M, Aval SF, Kouhi M, Akbarzadeh A, Nasrabadi HT, et al. 2014. Silver nanoparticles: Synthesis, properties, bio-applications and limitations. Crit Rev Microbiol. 1–8.
- Ahmadi A, Shirazi H, Pourbagher N, Akbarzadeh A, Omidfar K. 2014. An electrochemical immunosensor for digoxin using core-shell gold coated magnetic nanoparticles as labels. Mol Biol Rep. 41:1659–1668.
- Al-Ahmadi A. 2012. Quantum Dots - A Variety of New Applications. InTech, Chapters 6, Quantum Dots as a Light Indicator for Emitting Diodes and Biological Coding, Irati Ugarte, Ivan Castelló, Emilio Palomares and Roberto Pacios.
- Aldana J, Wang YA, Peng X. 2001. Photochemical instability of CdSe nanocrystals coated by hydrophilic thiols J Am Chem Soc. 123:8844–8850.
- Alizadeh E, Zarghami N, Eslaminejad MB, Akbarzadeh A, Barzegar A, Mohammadi SA. 2014. The effect of dimethyl sulfoxide (DMSO) on hepatic differentiation of mesenchymal stem cells. Artif Cells Nanomed Biotechnol. 1–8.
- Azzazy HM, Mansour MM, Kazmierczak SC. 2007. From diagnostics to therapy: prospects of quantum dots. Clin Biochem. 40:917–927.
- Ballou B, Ernst LA, Andreko S, Harper T, Fitzpatrick JA, Waggoner AS, Bruchez MP. 2007. Sentinel lymph node imaging using quantum dots in mouse tumor models. Bioconjug Chem. 18:389–396.
- Ben-Ari ET. 2003. Nanoscale quantum dots hold promise for cancer applications, JNCI J Natl Cancer Inst. 95:502–504.
- Bentolila LA, Ebenstein Y, Weiss S. 2009. Quantum dots for in vivo small-animal imaging. J Nucl Med. 50:493–496.
- Bharali DJ, Lucey DW, Jayakumar H, Pudavar HE, Prasad PN. 2005;Folate-receptor-mediated delivery of InP quantum dots for bioimaging using confocal and two-photon microscopy. J Am Chem Soc. 127:11364–11371.
- Bruchez Jr, Moronne M M, Gin P, Weiss S, Alivisatos AP. 1998. Semiconductor nanocrystals as fluorescent biological labels . Science. 281:2013–2016.
- Chan PM, Yuen T, Ruf F, Gonzalez-Maeso J Sealfon SC. 2005. Method for multiplex cellular detection of mRNAs using quantum dot fluorescent in situ hybridization. Nucleic Acids Res. 33.
- Chan WCW, Nie S. 1998. Quantum dot bioconjugates for ultrasensitive nonisotopic detection Science. 281:2016–2018.
- Chen HH, Ho YP, Wang TH, Leong KW. 2006. Intracellular Trafficking of QD-FRETNanoparticles for Gene Delivery,Biomedical Engineering, Johns Hopkins University, Baltimore,,Durham, NC., Molecular Therapy Volume.13.
- Choi HS, Ipe BI, Misra P, Lee JH, Bawendi MG, Frangioni JV. 2009. Tissue- and organ-selective biodistribution of NIR fluorescent quantum dots. Nano Lett. 9:2354–2359.
- Choi HS, Liu W, Misra P, Tanaka E, Zimmer JP, Itty Ipe B, et al. 2007. Renal clearance of quantum dots. Nat Biotechnol. 25:1165–1170.
- Choi HS, Liu WH, Liu FB Nasr K, Misra P, Bawendi MG, Frangioni JV. 2010. Design considerations for tumour-targeted nanoparticles. Nat Nanotechnol. 5:42–47.
- Chu TC, Shieh F, Lavery LA, Levy M, Richards-Kortum R, Korgel BA, Ellington AD. 2006. Biosens Bioelectron.21:1859.
- Chung JH, Kim YK, Kim KH, Kwon TY, Vaezmomeni SZ, Samiei M, et al. 2014. Synthesis, characterization, biocompatibility of hydroxyapatite-natural polymers nanocomposites for dentistry applications. Artif Cells Nanomed Biotechnol. 1–8.
- Contag CH, Bachmann MH. 2002. Advances in in vivo bioluminescence imaging of gene expression, Annu Rev Biomed Eng. 4: 235–260.
- Daraee H, Eatemadi A, Abbasi E, Aval SF, Kouhi M, Akbarzadeh A. 2014. Application of gold nanoparticles in biomedical and drug delivery. Artif Cells Nanomed Biotechnol. 1–13.
- Daraee H, Etemadi A, Kouhi M, Alimirzalu S, Akbarzadeh A. 2014. Application of liposomes in medicine and drug delivery. Artif Cells Nanomed Biotechnol. 1–11.
- Davaran S, Akbarzadeh A, Nejati-Koshki K, Alimohammadi S, Ghamari MF, Soghrati MM, Rezaei A, Khandaghi AA. 2013. In vitro studies of NIPAAM-MAA-VP copolymer-coated magnetic nanoparticles for controlled anticancer drug release, J Encapsul Adsorpt Sci. 3:108–115.
- Davaran S, Rezaei A, Alimohammadi S, Khandaghi AA, Nejati-Koshki K, Nasrabadi HT, Akbarzadeh A. 2014. Synthesis and physicochemical characterization of biodegradable star-shaped poly lactide- co-glycolide– β -cyclodextrin copolymer nanoparticles containing albumin. Adv Nanopart. 3:14–22.
- de Lang C. 2012. Quantum dots control brain cells for the first time by Catherine de Lange Magazine issue 2852.
- Derfus AM, Chan WCW, Bhatia SN. 2004. Probing the cytotoxicity of semiconductorc quantum dots. Nano Lett. 4:11–8.
- Dey NS, Rao M. 2011. Quantum dot: novel carrier for drug delivery. Int J Res Pharm Biomed Sci. 2.
- Eatemadi A, Daraee H, Zarghami N, Yar HM, Akbarzadeh A, Hanifehpour Y. 2014. Nanofiber; synthesis and biomedical applications. Artif Cells Nanomed Biotechnol. 1–11.
- Ebrahimi E, Abbasi E, Akbarzadeh A, Khandaghi AA, Davaran S. 2014. Novel drug delivery system based on doxorubicin-encapsulated magnetic nanoparticles modified with PLGA-PEG1000 copolymer. Artif Cells Nanomed Biotechnol. 1–8.
- Ebrahimi E, Khandaghi AA, Valipour F, Babaie S, Asghari F, Motaali S, et al. 2014. In vitro study and characterization of doxorubicin-loaded magnetic nanoparticles modifi ed with biodegradable copolymers. Artif Cells Nanomed Biotechnol. Early Online:1–9.
- Ebrahimnezhad Z, Zarghami N, Keyhani M, Amirsaadat S, Akbarzadeh A, Rahmati M, et al. 2013. Inhibition of hTERT Gene Expression by Silibinin-Loaded PLGA-PEG-Fe3O4 in T47D breast cancer cell line. BioImpacts. 3:67–74.
- Fang M, Peng C-W, Pang D-W, Li Y. 2012. Quantum dots for cancer research: current status, remaining issues, and future perspectives. Cancer Biol Med. 9:151–163.
- Fekri Aval S, Akbarzadeh A, Yamchi MR, Zarghami F, Nejati-Koshki K, Zarghami N. 2014. Gene silencing effect of SiRNA-magnetic modified with biodegradable copolymer nanoparticles on hTERT gene expression in lung cancer cell line. Artif Cells Nanomed Biotechnol. 1–6.
- Gac SL, Vermes I, Berg AVD. 2006. Quantum dots based probes conjugated to annexin V for photostable apoptosis detection and imaging. Nano Lett. 6:1863–1869.
- Gerion D, Parak WJ, Williams SC, Zanchet D, Micheel CM, Alivisatos AP. 2002. Sorting fluorescent nanocrystals with DNA. J Am Chem Soc. 124:7070–4.
- Gerion D, Pinaud F, Williams SC. 2001. Synthesis and properties of biocompatible water-soluble silica-coated CdSe/ZnS semiconductor quantum dots. J Phys Chem B. 105:8861–8871.
- Ghasemali S, Nejati-Koshki K, Akbarzadeh A, Tafsiri E, Zarghami N, Rahmati-Yamchi M, et al. 2013.,Study of inhibitory effect of β- cyclodextrin-helenalincomplex on HTERT gene expression in T47D breast cancer cell line by real timequantitative PCR (q-PCR). Asian Pac J Cancer Prev. 14:6949–6953.
- Gregor PC. 2010. Drummen, Quantum Dots—From Synthesis to Applications in Biomedicine and Life Sciences. Int J Mol Sci. 11:154–163
- Han M, Gao X, Su LZ. 2001. Quantum-dot-tagged microbeads for multiplexed optical coding of biomolecules. Nat Biotechnol. 19: 631–635.
- Hardman R. 2006. A toxicologic review of quantum dots: toxicity depends on physicochemical and environmental factors. Environ Health Perspect. 114:165–172.
- Hoshino A, Manabe N, Fujioka K, Hanada S, Yasuhara M, Kondo A, Yamamoto K. 2008. GFP expression by intracellular gene delivery of GFP-coding fragments using nanocrystal quantum dots. Nanotechnology.
- Hyun BR, Chen HY, Rey DA, Wise FW, Batt CA. 2007. Near-infrared fluorescence imaging with water-soluble lead salt quantum dots. J Phys Chem B. 111:5726–5730.
- Inoue Y, Izawa K, Yoshikawa K, Yamada H, Tojo A, Ohtomo K. 2007. In vivo fluorescence imaging of the reticuloendothelial system using quantum dots in combination with bioluminescent tumour monitoring. Eur J Nucl Med Mol Imaging. 34:2048–2056.
- Jacak L, Hawrylak P, W´ojs A .1998. Quantum Dots. Berlin Heidelberg: Springer-Verlag.
- Karabanovas V, Zakarevicius E, Sukackaite A, Streckyte G, Rotomskis R. 2008. Examination of the stability of hydrophobic (CdSe)ZnS quantum dots in the digestive tract of rats. Photochem Photobiol Sci. 7:725–729.
- Kho R, Torres-Martinez CL, Mehra RK. 2000. A simple colloidal synthesis for gram-quantity production of water-soluble ZnS nanocrystal powders J. Colloid Interface Sci. 227:561–566.
- Kouhi M, Vahedi A, Akbarzadeh A, Hanifehpour Y, Joo SW. 2014. Investigation of quadratic electro-optic effects and electro absorption process in GaN/AlGaN spherical quantum dot. Nanoscale Res Lett. 9:131–136.
- K-Yong T, Roy I, Ding H, Bergey EJ, Prasad PN. 2009. Biocompatible near-infrared quantum dots as ultrasensitive probes for long-term in vivo imaging applications. Small. 5:1997–2004.
- Lakowicz JR. 1999. Principles of Fluorescence Spectroscopy. Kluwer Academic/Plenum Publishers.
- Larson DR, Zipfel WR, Williams RM, Clark SW, Bruchez MP, Wise FW, Webb WW 2003. Water-soluble quantum dots for multiphoton fluorescence imaging in vivo Science. 300:1434–1436.
- Leatherdale CA, Woo WK, Mikulec FV, Bawendi MG. 2002. On the absorption cross section of CdSe nanocrystal quantum dots. J Phys Chem B. 106.
- Li L, Daou TJ, Texier I, Chi TTK, Liem NQ, Reiss P. 2009. Highly luminescent cuins 2/ZnS core-shell nanocrystals: cadmium-free quantum dots for in vivo imaging. Chem Mater. 21:2422–2429.
- Liu W, Choi HS, Zimmer JP, Tanaka E, Frangioni JV, Bawendi M. 2007. Compact cysteine-coated CdSe(ZnCdS) quantum dots for in vivo applications. J Am Chem Soc. 129:14530–14531.
- Medintz IL, Clapp AR, Mattoussi H, Goldman ER, Fisher B, Mauro JM. 2003. Self-assembled nanoscale biosensors based on quantum dot FRET donors. Nat Mater. 2:630–638.
- Michalet X, Pinaud FF, Bentolila LA, Tsay JM, Doose S, Li JJ, et al. 2005. Quantum dots for live cells, in vivo imaging, and diagnostics Science. 307:538–544.
- Mishra S, Tripathy P, Sinha SP. 2012. Advancements in the field of quantum dots. Int J Adv Res Technol. 1:1–5.
- Modani S, Kharwade M, Nijhawan M. 2013. Quantum dots: a novelty of medical field with multiple applications. Int J Curr Pharm Res. 5:55–59.
- Mohs AM, Duan HW, Kairdolf BA, Smith AM, Nie SM. 2009. Proton- resistant quantum dots: stability in gastrointestinal fluids and implications for oral delivery of nanoparticle agents. Nano Res. 2:500–508.
- Mokari T, Rothenberg E, Popov I, Costi R, Banin U. 2004. Science. 304:1787. [PubMed: 15205530].
- Murray CB, Norris DJ, Bawendi MG. 1993. Synthesis and characterization of nearly monodisperse CdE (E = sulfur, selenium, tellurium) semiconductor nanocrystallites J Am Chem Soc. 115: 8706–8715.
- Nasrabadi HT, Abbasi E, Davaran S, Kouhi M, Akbarzadeh A. 2014. Bimetallic nanoparticles: preparation, properties, and biomedical applications. Artif Cells Nanomed Biotechnol. 1–5.
- Papagiannaros A, Upponi J, Hartner W, Mongayt D, Levchenko T, Torchilin V. 2010. Quantum dot loaded immunomicelles for tumor imaging. BMC Med Imaging.10:article 22.
- Pathak S, Choi SK, Arnheim N, Thompson ME. 2001. Hydroxylated quantum dots as luminescent probes for in situ hybridization. J Am Chem Soc. 123:4103–4.
- Peng X, Manna U, Yang W, Wickham J, Scher E, Kadavanich A, Allvisatos AP. 2000. Shape control of CdSe nanocrystals Nature (London). 404:59–61.
- Peng X, Wickham J, Alivisatos AP. 1998. Kinetics of II–VI and III–V colloidal semiconductor nanocrystal growth: ‘‘focusing’’ of size distributions, J Am Chem Soc. 120:5343–5344.
- Peng ZA, Peng X. 2001. Mechanisms of the shape evolution of CdSe nanocrystals , J Am Chem Soc. 1389–1395.
- Pourhassan-Moghaddam M, Rahmati-Yamchi M, Akbarzadeh A, Daraee H, Nejati-Koshki K, Hanifehpour Y, Joo SW. 2013. Protein detection through different platforms of immuno-loop-mediated isothermal amplification. Nanoscale Res Lett. 8:485.
- Qi L, Gao X. 2008. Emerging application of quantum dots for drug delivery and therapy. Expert Opin Drug Deliv. 5.
- Rahimzadeh A, Tabatabaei Mirakabad FS, Movassaghpour A, Shamsasenjan K, Kariminekoo S, Talebi M, et al. 2014. Biotechnological and biomedical applications of mesenchymal stem cells as a therapeutic system. Artif Cells Nanomed Biotechnol. Early Online:1–12.
- Resch-Genger U, Grabolle M, Cavaliere-Jaricot S, Nitschke R, Nann T. 2008. Quantum dots versus organic dyes as fluorescent labels . Nat Methods. 5:763–775.
- Smith AM, Duan H, Mohs AM. 2008. Bioconjugated quantum dots for in vivo molecular and cellular imaging. Drug Deliv. 60:1226–1240.
- Smith RR, Cheng Z, De A, Koh AL, Sinclair R, Gambhir SS. 2008. Real-time intravital imaging of RGD-quantum dot binding to luminal endothelium in mouse tumor neovasculature. Nano Lett. 8:2599–2606.
- Sze SM. 1985. Semiconductor Devices Physics and Technology.
- Tabatabaei Mirakabad FS, Akbarzadeh A, Milani M, Zarghami N, Taheri-Anganeh M, Zeighamian V, et al. 2014. A Comparison between the cytotoxic effects of pure curcumin and curcumin-loaded PLGA-PEG nanoparticles on the MCF-7 human breast cancer cell line. Artif Cells Nanomed Biotechnol. 1–8.
- Tabatabaei Mirakabad FS, Akbarzadeh A, Zarghami N, Zeighamian V, Rahimzadeh A, Alimohammadi S. 2014. PLGA-based nanoparticles as cancer drug delivery systems. APJCP. Asian Pac J Cancer Prev. 15:517–535.
- Tilley RD, Warner JH, Yamamoto K, Matsui I, Fujimori H. 2005. Chem. Comm. 1833–1835.
- Tilley RD. 2008. Synthesis and applications of nanoparticles and quantum dots. Chem New Zealand. 146–150.
- Tokumasu F, Fairhurst RM, Ostera GR, Brittain NJ, Hwang J, Wellems TE, Dvorak JA.2005. Band 3 modifications in Plasmodium falciparum-infected AA and CC erythrocytes assayed by autocorrelation analysis using quantum dots. J Cell Sci. 118:1091–1098.
- Valizadeh A, Bakhtiary M, Akbarzadeh A, Salehi R, Frakhani SM, Ebrahimi O, et al. 2014. Preparation and characterization of novel electrospun poly(e-caprolactone)-based nanofibrous scaffolds. Artif Cells Nanomed Biotechnol. Early Online: 1–6.
- Wang Y, Tang Z, Correa-Duarte MA, Pastoriza-Santos I, Giersig M, Kotov NA, Liz-Marzan LM. 2004. Mechanism of strong luminescence photoactivation of citrate-stabilized water-soluble nanoparticles with CdSe cores, J Phys Chem B. 108:15461–15469.
- Warner JH, Hoshino A, Yamamoto K, Tilley RD. 2005. Angew Chem Int Ed. 44:4550–4554.
- Weng KC, Noble CO, Papahadjopoulos-Sternberg B, Chen FF, Drummond DC, Kirpotin DB, et al. 2008. Targeted tumor cell internalization and imaging of multifunctional quantum dot-conjugated immunoliposomes in vitro and in vivo. Nano Lett. 8:2851–2857.
- Winter JO, Liu TY, Korgel BA, Schmidt CE. 2001. Recognition molecule directed interfacing between semiconductor quantum dots and nerve cells Adv Mater. 13:1673–1677.
- Wuister SF, Swart I, van Driel F, Hickey SG, de Mello Donega C. 2003. Highly luminescent water-soluble CdTe quantum dots Nano Lett. 3:503–507.
- Xing Y, So MK, Koh AL, Sinclair R, Rao J. 2008. Improved QD- BRET conjugates for detection and imaging. Biochem Biophys Res Commun. 372: 388–394.
- Young SH, Rozengurt E. 2006. Am J Physiol Cell Physiol. 290:C728.
- Yu WW, Qu L, Guo W, Peng X. 2003. Experimental determination of the extinction coefficient of CdTe, CdSe and CdS nanocrystals Chem Mater. 15:2854–2860 [Correction, Chem. Mater. 16 (2004) 560].
- Yu WW, Wang YA, Peng X. 2003. Formation and stability of size-, shape-, and structure-controlled CdTe nanocrystals: ligand effects on monomers and nanocrystals Chem Mater. 15:4300–4308.
- Zhang W, Yao Y, Chen YS. 2011. Imaging and quantifying the morphology and nanoelectrical properties of quantum dot nanoparticles interacting with DNA. J Phys Chem C. 115:599–606.
- Zhuang J, Zhang X, Wang G, Li D, Yang W, Li T. 2003. Synthesis of water-soluble ZnS:Mn2 + nanocrystals by using mercaptopropionic acid as stabilizer J Mater Chem. 13:1853–1857.