Abstract
This paper aims to establish a novel and highly sensitive method to detect circulating tumor cells (CTCs) in the peripheral blood of patients with lung cancer. This therefore enables the discovery of invisible micrometastasis in the early stage of lung cancer, leading to better prognostic assessments of lung cancer and detection of the post-operative tumor recurrence and metastasis, treatment options, and evaluation of curative effects. In this research study, various lung cancer cells were mixed with adult blood samples to simulate blood samples of tumor patients. With novel test methods, CTCs in peripheral blood of lung cancer patients were calculated, after the reaction between the cells obtained from the mix and EpCAM (epithelial cell adhesion molecule) antibodies which were marked by immunomagnetic beads. The results showed that 18 out of 42 (42.9%) lung cancer patients had a positive CTCs, which increased with tumor enlargement or metastasis. CTCs were not detected in a total of 20 blood samples from healthy volunteers. This indicated that the technology of novel immunomagnetic bead-enrichment could effectively separate and identify CTCs in peripheral blood of lung cancer patients, which is of great clinical value for prognostic assessments and treatment guidance of lung cancer.
Keywords::
Introduction
The death rate of lung cancer patients stands first on the list of malignant tumors with poor prognosis. Hematogenous dissemination, an important route for lung cancer metastasis, is of significant importance in the detection of CTCs in peripheral blood of lung cancer patients. Immunomagnetic separation (IMS) is a novel immunologic technology that combines cell surface antigens with magnetic bead-connected McAbs to create an immune complex of antigen-antibody-magnetic bead. Affected by the external magnetic field, the complex takes mechanical movements to separate the target cell containing antigens from other cells, achieving cell separation with specificity (CitationHoeppener et al. 2012). The highly sensitive CellSearch system, based on IMS, can detect one signal CTC from more than 40 billion hemocytes (CitationRiethdort et al. 2007). However, the various steps involved in the operation cause significant differences in the rate of tumor cell recovery, ranging from 60% to 90% (CitationHelo et al. 2009). In addition, this technology is not fully accepted by pathologists because of its design shortages. Based on this technology, this paper integrates the EpCAM antibody with the G magnetic bead of anti-rat immunoglobulin, a new method for detecting CTCs. Via peripheral blood, this method can rapidly, swiftly, and conveniently detect the CTCs in patients with early lung cancer, so that treatment options and curative effects can be confirmed with the finding of early lung cancer micrometastasis, prognostic assessments of lung cancer, and detection of post-operative tumor recurrence and metastasis. In conclusion, this is a low-cost and high-result method, which benefits the separation and enrichment of CTCs in peripheral blood of cancer patients.
Materials and methods
Clinical samples
The test samples comprised venous blood samples of 42 lung cancer patients who had undergone only surgical treatment between August 2011 and February 2012, and samples of peripheral blood of 20 healthy volunteers, collected on an empty stomach in the morning. Among the lung cancer patients, 26 were males and 16 were females, ranging in age from 37 to 80 (68 on average). As for the healthy volunteers, 13 were males and 7 were females, ranging in age from 31 to 76 (64 on average). The samples of all 42 patients were submitted for censorship after surgery, and were pathologically diagnosed as non-small cell lung cancer. The patients with lung cancer patients were divided into different stages according to the international TNM staging system: 7 cases in stage I (4 cases of lung adenocarcinoma and 3 cases of lung squamous cell carcinoma); 9 cases in stage II (6 cases of lung adenocarcinoma and 3 cases of lung squamous cell carcinoma); 16 cases in stage III (8 cases of lung adenocarcinoma and 8 cases of lung squamous cell carcinoma); and 10 cases in stage IV (7 cases of lung adenocarcinoma and 3 cases of lung squamous cell carcinoma). A total of 42 patients were all first-visit patients, without any other history or evidence of epithelial tumor; while the healthy volunteers all underwent systematic physical examination, the results of which showed non-relevant disease history and family history of malignant tumor.
Cell culture
The ampoules were taken out from liquid nitrogen tanks and quickly put in enamel tanks with water at a temperature of 37°C to 40°C, then bottled up with constant shaking to unfreeze the ampoule as soon as possible. Disinfected by 75% ethyl alcohol, the ampoule was opened in the purification stage. Then, the epispastic cell suspension was closed in a centrifuge tube with 10 ml of culture solution, forming cell suspensions by blowing and beating. The supernatant was obtained after centrifuging at low speed (500 and 1000 r/min) for 5 min, and the above steps were repeated. Diluted with an appropriate culture solution, the ampoule was removed into a culture bottle in an incubator and further cultured with exchanged culture solution on the next day. The cells would go down in prosperity once they reached 80%, when the culture solution was drained off. Then, 5 mL of PBS was added and shaken, and drained off. The inter-cellular space was microscopically observed after adding 1–2 ml of 0.25% pancreatin for 2 to 3 min, which was drained off once obvious space was observed. Repeated blowing and beating, with the addition of RPMI-1640, to make a unicellular suspension. Usually, one bottle of cells could produce three bottles of posterity which continued to reproduce. When the posterity increased to 80%, the culture solution was drained off. Then, 5 mL of PBS was added and shaken, and then drained off. The inter-cellular space was microscopically observed after adding 1–2 ml of 0.25% pancreatin for 2 to 3 min, which was drained off once obvious space was observed. Repeated blowing and beating was carried out, with the addition of PBS, to make a unicellular suspension that would be centrifuged in the speed of 1500 rpm/min for 10 min. The unicellular suspension was kept in incubators at 4°C for 20 min, at − 20°C for 1 h, and then at − 80°C for 3 h, and finally placed in liquid nitrogen tanks.
Collection of peripheral blood samples
On the premise that patients and healthy volunteers were informed and had agreed, samples of 10 ml of peripheral blood were collected from the median cubital vein, on an empty stomach in the morning. After the immediate injection of the blood samples into a sterile EDTA-2Na anti-freezing blood collection vessel, the vessel was evenly shaken to fully mix anticoagulant and blood in case of blood coagulation. Then, the samples were placed in a refrigerator at 4°C, and processed within 6 h.
Drawing of the of CTC test equipment assembly
The novel test equipment was mainly made up of four items general laboratory apparatus: peristaltic pump (B), horizontal table and self-made enrichment and separation container (C), and vacuum pump (D) (as shown in ). Linked with a beaker (A), B pumped PBS at the speed of 10 × 10 ml/h from A to C. Linked with an empty beaker (E) that was designed to contain PBS, D pumped PBS at the speed of 10 × 10 ml/h from C. The whole process achieved the removal of parenchymal cells by injecting the PBS rinse into C, vibrating the horizontal table and pumping liquid from C. C was made of an inclined upholder, a strong magnetic patch, and a wooden box with the internal surface hydrophobically treated (its bottom was equipped with a standardized slide). Of the three parts, the inclined upholder would lead PBS at a low angle of a certain degree to wash away more white blood cells; the super magnet with a magnetic induction intensity of 4500mT applied a strong magnetic field to firmly adsorb cancer cells that had combined with EpCAM antibodies marked by immunomagnetic beads on the slide; with the exception of the bottom slide, all sides of the wooden box with the internal surface hydrophobically treated were covered with wax, so that all cells could stay on the slide.
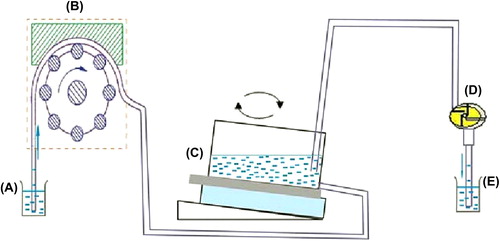
The function of the horizontal table was to slowly vibrate the liquid in C, to keep the liquid flowing around. Cells that did not combine with antibodies were suspended, to avoid the clinging of parenchymal cells on slides. Having reacted with immunomagnetic beads and EpCAM antibodies, the samples were slowly injected into C and B, the horizontal table and D were opened in sequence after 3 min. During the whole process, it was of great importance to control the speed at which D pumped PBS; the horizontal table vibrated the samples and D pumped PBS. Fast flow caused CTCs to wash away with white blood cells, while slow flow made more white blood cells cling to the slides, which affected the microscopic observation of CTCs. Therefore, we performed many experiments on the control of flow rate, to ensure the least white cells under the condition of reducing the loss of CTCs to the minimum.
Establishment of CTC detection method
We calculated A549 cell strains that were cultured in vitro after trypsinization, and obtained about 100 cancer cells through gradient dilution. The solution of the addition of 2 ml peripheral blood samples from healthy volunteers was intended to be centrifuged for 7 min. With the removal of the supernatant, 0.72% ammonium chloride solution at five times the volume was added, overturned, and incubated. The solution obtained after the above steps was centrifuged for 10 min. The supernatant was then removed and the splitting was repeated once more. A total of 100ul of phosphate buffer was used to suspend the remaining cell sediments that were incubated under 4°C in the horizontal table for 30 min, with the addition of 5ul magnetic marked EpCAM antibodies. After immunoreaction, the cells were completely transmitted to the enrichment and separation container with a slide-enclosed bottom. The container was stacked on a strong magnetic patch which equaled the slide in size, and fixed on the horizontal table. The slide was HE-dyed (hematoxylin and eosin) by washing with 50 ml of phosphate buffer, and placed under a light microscope to count the number of cancer cells. In this process, the run-off of cancer cells would be reduced by adjusting the container's angle level, the table's vibrational frequency, and the rinse liquid's flow rate.
HE dyeing
The cells obtained from the slide were recognized and identified by HE dyeing. The process of HE dyeing was performed as follows: the slide was soaked for 15 min with 95% ethyl alcohol and for 3 min with distilled water; soaked in tap water for 10 min after a 10-min hematoxylin dyeing process, and washed up with distilled water. They were washed with distilled water again after soaking in warm water at 50°C for 5 min, dehydrated with 95% ethanol, and 5 s later, dyed with eosin dye for 2 min. After washing twice with 75% ethanol, the cells on the slide were dehydrated for 2 min with 95% ethanol. Then, using 95% fresh ethanol, they were dehydrated for 2 min, and then cleared with xylene for 5 min, and finally, cleared with fresh xylene for another 5 min. The slide, which was mounted with neutral gum, was observed under a light microscope.
Measurement of cell recovery
In order to study the sensitivity of this novel method of detecting CTCs, 5 healthy volunteers were selected, for the collection of 10 ml of peripheral blood samples from each person. The blood samples were collected in sterile EDTA-2Na anti-freezing pipes and divided into 5 pipes with 2 ml each. Different numbers of A549 (50, 100, 200, 500, and 1000) were mixed respectively into the five pipes. Then, the peripheral blood samples were enriched by mixing known numbers of cancer cells based on the above-mentioned system. After that, the samples were dyed with HE and the number of captured cancer cells were counted under the microscope; each experiment was repeated five times. Clinical sample test: cancer cells were tested by applying established separation/ identification system after the regular process of collecting peripheral blood samples of 42 patients with lung cancer.
Statistical analysis
All data were subjected to the chi-square test and analyzed by the statistical software SPSS13.0, and the result was considered to be of statistical significance if p < 0.05.
Result
Authentication of CTCs
The result of HE dyeing changed once A549 cells reacted with the EpCAM antibodies and the immunomagnetic bead. presents the results of HE dyeing before and after the reaction of A549 cells with the immunomagnetic antibodies. The original color of A549 cytoplasm was ingrain blue, and A549's complete karyon was visible; however, the cytoplasm presented as pink or an understain of lavender after immunomagnetic enrichment and separation. Besides, the nucleus was out of shape with uneven dyeing, in granular or dotted form, performing as a large nucleus or multi-nucleus.
Optimization of operative equipment and effects on cell separation
In order to enhance the recovery of A549 as well as to lower the number of white cells, we optimized the equipment. The speed of the peristaltic pump was the same as that of the vacuum pump, both at 10 × 10 ml/h, so that PBS ran in and out of the wooden box at a balanced speed without impacting the cells. The speed of the horizontal table varied between 20, 40, 60 and 80 rpm/min; while the angle of the self-made enrichment and separation container differed, at 5 degrees, 15 degrees and 20 degrees. To maintain optimal device parameters, 100 A549 cells were mixed into 2 ml samples of peripheral blood of healthy volunteers, to calculate captured cancer cells under different speeds of the horizontal table and at different angles the of enrichment and separation container (refer ). With speed at 60 rpm/min or 80 rpm/min and at a container angle of 25 degrees, very few CTCs were detected, because the CTCs were washed away with white cells as the speed was too high and the angle too steep. More cells remained in the slide under the other four conditions, namely a speed of 20 rpm/min with a 5 degree angle, 20 rpm/min with a 15 degree angle, 40 rpm/min with a 5 degree angle, and 40 rpm/min with a 15 degree angle. The remaining cells were HE dyed for identification. Results showed that white cells were much more in number with the first three parameters than with the fourth parameter (), which was of no use in CTC observation and identification. With comprehensive analysis of results from and , the speed of the horizontal table was set as 40 rpm/min and the angle of incline as 15 degrees. This setting reduced the white cells without affecting the capture of CTCs, which was convenient for microscope observation and recognition.
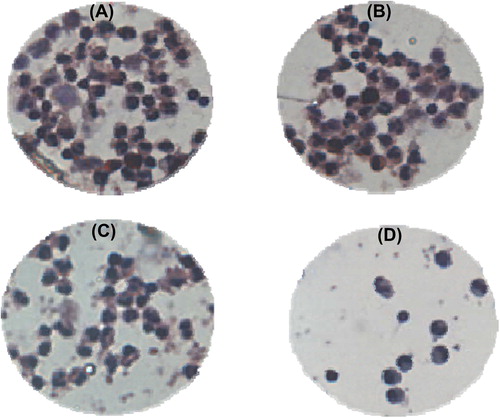
Table I. Number of CTCs under different parameters.
Recovery, accuracy and specificity of CTC detection method
A549 cells of different numbers were mixed into the peripheral blood samples of 5 healthy volunteers, to verify the sensitivity of the novel CTC detection method whose recovery was between 68% and 82% (). The recycled cells had remarkable correlation with the added cells, and the correlation coefficient was 0.9958 (p < 0.001). The regression equation of CTCs mixed into cells was y = 0.6419x + 8.8875. With the decrease in the number of cancer cells mixed, the variable coefficient gradually increased from 6.4% (mix of 1000 A549s) to 18.4% (mix of 50 A549s) (). CTCs were detected in the peripheral blood samples of 20 healthy volunteers.
Table II. Evaluation and test results of cell recoveries from samples of peripheral blood from healthy volunteers mixed with A549.
Detection results of CTCs in peripheral blood samples of lung cancer patients
With the adoption of the above-mentioned cell enrichment and separation method, 42.9% (18/42) of blood samples were detected with CTCs. According to the clinical features of patients, the detected results were divided into several groups (). The positive rate of CTCs detected had no obvious relation with the patients’ age and gender (p > 0.05), but was closely related to tumor size and clinical stages (p < 0.05). More CTCs were detected in patients as the tumor or the level of TNM increased, which was the expected result, that is CTCs were more easily detected in the peripheral blood of patients at a more advanced stage of cancer with tumor metastasis (CitationHuang et al. 2007). The HE dyeing results of CTCs detected in the peripheral blood samples of lung cancer patients are shown in , and the dyeing characteristics are in accordance with those of A549 (presented in ).
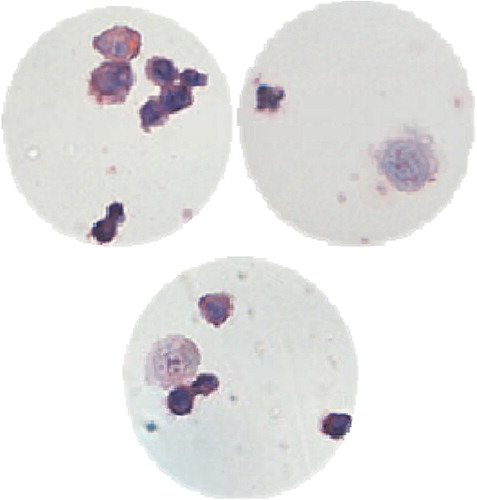
Table III. Results of CTCs detected according to the clinical features of lung cancer patients.
Malignant tumor metastasis is the primary cause of failure of oncotherapy. Patient prognosis can be improved if the micrometastasis can be noted early and timely treatment options can be adopted. But current examinations, such as clinical assessment and imaging examinations, cannot promptly and effectively reveal the occurrence of micrometastasis. Therefore, there is a great need for a new detection method that can reveal micrometastasis early. Tumor cells will enter into blood circulation from a primary lesion or metastatic lesion, which will become CTCs in blood circulation if killed or eliminated by the body autoimmunity system. Therefore, CTC formation is an important part of the occurrence of tumor micrometastasis. Besides the CellSearch system, more common CTC detection methods are also relatively stable, sensitive, and with high specificity, for instance, RT-PCR (CitationSchmitt and Foekens 2009, CitationYu et al. 2011), Immunocytochemistry (ICC) (CitationWulfing et al. 2006), and Flow cytometry (FCM) (CitationJiang and Zang 2011, CitationCohen et al. 2009). Definitely, these methods as examples have various defects in improving the sensitivity and specificity of CTC detection as well. However, compared to other similar detection methods, the novel CTC detection method in this research study has the following features.
Novel design: the cells’ enrichment and separation are completed in a signal slide by self-made tumor enrichment containers, which enables the separation and calculation of CTC to further evaluate expressions of tumor markers in the level of cells.
Simple operation: it is easy to operate the experimental installations including the peristaltic pump, horizontal table, and self-made enrichment and separation container and vacuum pump. Besides, this test avoids the influence of manual operation during enrichment and separation processes of immunomagnetic beads. This test can be made repeatable with semi-automation.
Low cost: laboratories can provide common reagents, such as EpCAM anti-body, magnetic beads, and HE reagent dye dispenser; moreover, with self-made PBS buffer solution and ammonium chloride lysis, the expense of the test is reduced.
Reliable results: the CTC identification applies HE dyeing, a mature pathology technique, whose results are still the golden standards of cell conditions in cellular pathology. Thus, the test results will be more reliable with conventional methods of cell dyeing to identify CTCs.
In addition, conventional CTC detection methods require 7.5 ml anti-freezing peripheral blood samples from patients and volunteers for the experiment (CitationFarace et al. 2011, CitationWeissenstein et al. 2012, CitationHou et al. 2011). In comparison, this it would be easier to receive patients’ and volunteers’ approvals for this experiment, because it limits the anti-freezing peripheral blood sample required to only 2 ml. However, the decrease of blood samples does not mean a decrease in sensitivity. In the early stage, the recovery of the tumor cell-mixed test was between 68%∼82% when the test groups were not marked by immunomagnetic bead antibodies. More than 27 tumor cells were captured with minimum A549s mixed into 2 ml of anti-freezing peripheral blood. Lung cancer patients, regardless of whether the cancer was in the early stage, or whether the tumor diameter was less than 3 cm, could be detected with positive CTCs, which proves the high sensitivity of this experiment. In addition, high specificity has also been witnessed since no CTC was detected in 20 healthy volunteers. Among 42 lung cancer patients, 18 had positive CTCs which were similar to those of tumors of epithelial origin (CitationHou et al. 2011, CitationBevilacqua et al. 2009, CitationOkegawa et al. 2009). Compared to the iconographic tests, such as CT, the detection and random visit of CTCs in lung cancer patients are inexpensive and more easily accepted by patients.
Conclusion
The novel CTC detection equipment is convenient and time-saving, with high sensitivity and specificity to detect CTCs in the peripheral blood of lung cancer patients, using EpCAM tumor markers combined with immunomagnetic beads. The results of this detection method prove that CTC detection rate is closely related to tumor size and TNM stage. With the tumor enlarging and malignantly transferring, more positive CTCs are detected. On the basis of CTC detection results, early discovery of invisible lung cancer micrometastasis is possible, and it is also possible to assess the curative effects of anti-tumor drugs, judge the patient's prognosis, and guide individualized clinical treatment.
Declaration of interest
The authors report no declarations of interest. The authors alone are responsible for the content and writing of the paper.
References
- Bevilacqua S, Gallo M, Franco R, Rossi A, De Luca A, Rocco G, et al. 2009. A “live” biopsy in a small-cell lung cancer patient by detection of circulating tumor cells. Lung Cancer. 65:123–125.
- Cohen SJ, Punt CJ, Iannotti N, Saidman BH, Sabbath KD, Gabrail NY, et al. 2009. Prognostic significance of circulating tumor cells in patients with metastatic colorectal cancer. Ann Oncol. 20: 1223–1229.
- Okegawa T, Nutahara K, Higashihara E. 2009. Prognostic significance of circulating tumor cells in patients with hormone refractory prostate cancer. J Urol. 181:1091–1097.
- Farace F, Massard C, Vimond N, Drusch F, Jacques N, Billiot F, et al. 2011. A direct comparison of CellSearch and ISET for circulating tumor-cells detection in patients with metastatic carcinomas. Br J Cancer. 105:847–853.
- Hoeppener AE, Swennenhuis JF, Terstappen LW. 2012. Immunomagnetic separation technologies. Recent Results Cancer Res. 195:43–48.
- Hou JM, Matthew K, Tim W, Robert S, Lynsey P, Andrew H, et al. 2011. Circulating tumor cells as a window on metastasis biology in lung cancer. Am J Pathol. 178:989–996.
- Helo P, Cronin AM, Danila DC, Wenske S, Gonzalez-Espinoza R, Anand A, et al. 2009. Circulating prostate tumor cells detected by RT-PCR in men with localized or castration-refractory prostate cancer: concordance with CellSearch assay and association with bone metastasis and with survival. Clin Chem. 55:765–773.
- Huang TH, Wang Z, Li Q, Qi H, Zhou HX, Li FR. 2007. Clinical significance of nano immunomagnetic beads enrichment and test of circulating tumor cells in patients with non-small cell carcinoma. Chin J Oncol. 29:676–680.
- Jiang WF, Zang HL. 2011. Enrichment and detection of circulating tumor cells in peripheral blood. Chin Ger J Clin Oncol. 10:240–244.
- Riethdort S, Fritsche H, Muller V, Rau T, Schindlbeck C, Rack B, et al. 2007. Detection of circulating tumor cells in peripheral blood of patients with metastatic breast cancer: a validation study of the CellSearch system. Clin Cancer Res. 13:920–928.
- Schmitt M, Foekens JA. 2009. Circulating tumor cells in blood of primary breast cancer patients assessed by a novel RT-PCR test kit and comparison with status of bone marrow-disseminated tumor cells. Breast Cancer Res. 11:109.
- Wulfing P, Borchard J, Buerger H, Heidl S, Zänker KS, Kiesel L, Brandt B. 2006. HER-2 positive circulating tumor cells indicate poor clinical outcome in stage I t o III breast cancer patients. Clin Cancer Res. 12:1715.
- Weissenstein U, Schumann A, Reif M, Link S, Toffol-Schmidt UD, Heusser P. 2012. Detection of circulating tumor cells in blood of metastatic breast cancer patients using a combination of cytokeratin and EpCAM antibodies. BMC Cancer. 12:206.
- Yu M, Stott S, Toner M, Maheswaran S, Haber DA. 2011. Circuling tumor cells: approaches to isolation and characterization. J Cell Bilo. 192:373–382.