Abstract
In this work, we have formulated novel nanospheres that could be used in the controlled release of the anticancer drug, 5-fluorouracil (5-FU). The nanospheres are composed of magnetite, containing chitosan (CS) and methylcellulose (MC). The drug entrapment was achieved through the encapsulation and adsorption processes. The effects of the preparation conditions, such as magnetite content, CS/MC ratio, crosslinking concentration, exposure time to glutaraldehyde (GA), and the drug/polymer ratio were investigated for both processes. The 5-FU release was found to follow the Fickian mechanism, and the Langmuir isotherm for the nanospheres was achieved through encapsulation and adsorption processes, respectively.
Introduction
5-Fluorouracil (5-FU) (5-fluoro-2,4-pyrimidinedione) is a chemotherapeutic agent that is widely used for the treatment of breast cancer, advanced gastrointestinal tract cancer, and several other types of cancers (CitationZinutti et al. 1996). However, its clinical application is limited due to its toxicity (CitationZinutti et al. 1996, CitationHuang et al. 2010), as it is for many other anticancer drugs. Thus, targeted therapy can be an effective way to lower its toxic effects and to enhance the therapeutic effect. Owing to its short biological half-life and poor oral absorption, the utilization of controlled release systems for 5-FU is a promising approach (CitationOlukman et al. 2012).
To reach therapeutic levels at the desired site, high dosages of an anticancer drug must be administered. However, since only a fraction of the dose will actually reach the intended disease site, the toxic side effects will not occur at the non-targeted organs. Developing colloidal drug delivery systems which can physically direct the drug to the desired site, can be a solution to this problem. The use of magnetic iron oxide nanoparticles for this purpose has attracted researchers in various fields (CitationGan et al. 2008, CitationKuroiwa et al. 2008, CitationButoescu et al. 2009, CitationGuo et al. 2009, CitationJing et al. 2008, CitationCiofani et al. 2009, CitationGu et al. 2006, CitationLu et al. 2007). Magnetic nanoparticles have been studied recently as drug carriers, due to their interesting properties (like size and magnetic properties) and advantages (such as low toxicity and high surface area) (CitationBulte and Kraitchman 2004, CitationOkon et al. 1974, CitationLi et al. 2008). Magnetite (Fe3O4) is a well-known magnetic material which can be used in targeted drug delivery, using a magnetic field. However, magnetite nanoparticles have some limitations. They tend to aggregate due to strong dipole-dipole attractions between particles, and the particles have low drug-loading capacity. To preclude this problem, these particles have been modified by synthetic and natural polymers, by which the resultant materials reveal enhanced loading capacity and drug release (CitationLi et al. 2008, CitationYang et al. 2006, CitationDorniani et al. 2012, CitationXu et al. 2013, CitationMody et al. 2014). For this purpose, chitosan (CS) and methylcellulose (MC) have been used in this study. CS is a natural linear biopolyaminosaccharide derived from alkaline deacetylation of chitin. CS has received increasing attention as a renewable polymeric material, due to its chemical properties (hydrogel, polycationic, biodegradability, biocompatibility, and presence of reactive groups such as –OH and –NH2), and has been widely used in many fields such as controlled release (CitationLee et al. 2008, CitationTrapani et al. 2009, CitationZhang et al. 2009), metal adsorption (CitationChassary et al. 2004), and protein adsorption (CitationMao et al. 2004). Li et al. prepared Fe3O4-chitosan nanoparticles and investigated the effect of the magnetite content of nanoparticles (CitationLi et al. 2008). They have found that the size of the nanoparticles decreased with the increase in magnetite content. Yang et al. synthesized biodegradable magnetite containing poly(ε-caprolactone) nanoparticles, with the anticancer drugs gemcitabine and cisplatin (CitationYang et al. 2006). They have reported that compared to gemcitabine, the release behavior for cisplatin was higher in sustainability. Saikia et al. prepared carboxymethyl starch-CS-coated iron oxide magnetic nanoparticles and studied controlled release of isoniazid from these nanoparticles (CitationSaikia et al. 2014). However, there are some disadvantages of using CS in drug delivery systems, as reported by the researchers. Yao et al. prepared CS nanoparticles solidified by different amounts of glutaraldehyde and modified with either biotin or biotin and avidin, and examined their cytotoxicity on HepG2 cells. Their results showed that CS nanoparticles, with a higher extent of solidification, exhibited a more vigorous inhibitory effect against HepG2 cells (CitationYao et al. 2013); Qi et al. prepared Copper (II)-loaded CS nanoparticles and investigated the cytotoxic activities of these nanoparticles. They found that Copper(II)-loaded CS nanoparticles elicit dose-dependent inhibitory effects on the proliferation of tumor cell lines (CitationQi et al. 2005); Loh et al. published data on the toxicology of CS particles against CaO-2 cells, and the particles were observed to inflict extensive damage to the intracellular organelles (CitationLoh et al. 2012); Loh et al. also investigated the uptake of CS nanoparticles into the cell's nucleus, and the resulting necrotic autophagic cell death (CitationLoh et al. 2010).
For this reason, we have blended CS with MC and prepared magnetite containing CS/MC, an interpenetrating network (IPN) of nanospheres to achieve a controlled release profile of 5-FU. Entrapment of 5-FU was achieved through both encapsulation and adsorption processes, and the entrapment efficiency and swelling degree of the nanospheres were investigated. Moreover, the effects of exposure time to GA, crosslinking concentration, magnetite content, CS/MC ratio, and drug/polymer ratio, on the release of 5-FU, were also investigated.
Materials
5-Fluorouracil, chitosan (CS), methyl cellulose (MC), and 2-propanol were supplied from Sigma-Aldrich (Steinheim, Germany). Paraffin was obtained from Birpa (Ankara, Turkey). Glutaraldehyde (25% w/w) solution, Tween® 80, C6 H14, Na2HPO4, NaH2PO4, FeCl3, FeCl2.4H2O, and NH4OH were all supplied from Merck (Darmstadt, Germany), and used as received.
Methods
Synthesis of magnetic iron oxide nanoparticles
Magnetite was synthesized using an aqueous Fe+ 3/Fe+ 2 solution and ammonium hydroxide. FeCl3 and FeCl2.4H2O were dissolved in water and blended in molar ratios. Then NH4OH (25% w/w) was added to this solution, and the resultant mixture was stirred for 30 min. The precipitated magnetite was black in color, separated by using a strong magnet, and washed several times until the pH was maintained at 7. Finally, the particles were dried in an oven at 70°C. The overall chemical reaction is as given in the following equation (CitationGupta and Gupta 2005):
Preparation of nanospheres containing magnetite particles
Empty nanospheres of CS/MC were prepared by the emulsion-crosslinking method. Briefly, CS and MC (2% w/w) were dissolved in acetic acid (2% w/w) by stirring. The polymer solution was emulsified using a high speed stirrer in light liquid paraffin (30 ml) containing Tween® 80 (0.5 ml) and magnetite. Then, GA was added slowly, and the emulsion was further stirred at 2700 rpm for 30 min. Later, the nanospheres were collected using an ultracentrifuge (Electro-Mag M4812), washed repeatedly with n-hexane and 2-propanol, and then dried in an oven at 40°C and stored for further analysis. The preparation conditions of empty nanospheres have been shown in . 5-FU was added to the nanospheres by following two procedures. In the first method, the required amount of 5-FU was dispersed in the polymer mixture for 12 h. This water phase was added into the oil phase, stirred strongly, and then crosslinked with GA and washed, then dried, similar to the process for preparation of empty nanospheres. The preparation conditions of nanospheres containing 5-FU have been presented in . The second method was the adsorption of 5-FU onto empty magnetic nanospheres. For this purpose, placebo nanospheres were immersed into the drug solution for different time intervals, then the nanospheres were collected by ultracentrifugation at 10000 rpm, and dried in an oven at 40°C. The adsorption capacity of the nanospheres was calculated using the following equation:
Where q: adsorption capacity, C0 and C: initial and final drug concentrations, respectively, V: volume of drug solution, and m: mass of nanospheres.
Table I. Conditions of preparation of empty nanospheres.
Table II. Formulation codes and different ingredients used in the preparation of the nanospheres.
The in vitro release results were analyzed using the following equation proposed by CitationPeppas (1985).
Where Mt: the amount of 5-FU released at time t, M∞: the total amount of drug loaded, k: a constant characteristic of the drug-polymer system, and n: the diffusion exponent that suggested the nature of the release mechanism. Fickian release was defined by an initial t1/2 time dependence of the fractional release for slabs, cylinders, and spheres (CitationRitger et al. 1987). A value of n = 0.5 indicated the Fickian transport, while n = 1 was of Case II, or non-Fickian transport (swelling controlled) (CitationBabu et al. 2006). The intermediary values ranging between 0.5 and 1.0 were indicative of anomalous transport (CitationRitger et al. 1987, CitationIşıklan et al. 2008, Citationİnal et al. 2008).
Characterization
The glass transition temperatures (Tg) of the nanospheres were determined using a Dupont General V4.1C 2000 differential scanning calorimeter (DSC) (USA). The measurements were performed over the temperature range of 0°C to 300°C, at the heating rate of 100°C/min. FTIR spectra of the magnetite, pure CS and MS, and the empty and drug-loaded nanospheres containing magnetite, were recorded on a Mattson 1000 FTIR spectrometer (Welwyn Garden, England). The electrokinetic properties (zeta potential) of magnetite particles, empty, and 5-FU-loaded magnetite containing CS/MS nanospheres were recorded on a Nano-ZS, Malvern, (UK). The XRD patterns of magnetite and A1e formulated nanospheres were recorded using a Rigaku Ultima-IV model diffractometer. The morphology of the nanoparticles was investigated using FEI, Tecnai G2 Spirit Biotwin model HRTEM.
Determination of 5-Fluourouracil content of the nanospheres
The 5-Fluourouracil content of the nanospheres was determined through extraction of the drug. For this purpose, a known mass of nanospheres was placed in water (50 mL) and refluxed under ambient conditions for 2 h, to ensure the complete extraction of 5-FU from the nanospheres. Then, the solution was centrifuged at 10000 rpm for 5 mins and filtered. The resulting aliquot was analyzed for its 5-FU content by using a Unico 4802 ultraviolet-visible spectrophotometer at a wavelength of 266 nm. The entrapment efficiency (%) was then calculated using Eq. (4), and the results have been stated in .
Swelling studies
The equilibrium swelling degree of placebo nanospheres was determined by the gravimetric measurement of the extent of their swelling in water at 37°C. To ensure complete equilibration, the samples were allowed to swell for 24 h. The excess surface-adhesion of nanospheres was removed by blotting. The swollen nanospheres were weighed with an electronic balance (XB 220 A Precisa, United States). The nanospheres were then dried in an oven at 40°C, until there was no change in the dried mass of the samples. The equilibrium swelling degree (%) was calculated as follows:
where Ms and Md were the mass of the swollen nanospheres and dry nanospheres, respectively.
In vitro release studies of 5-fluorouracil
In vitro drug release studies from the nanospheres were carried out in phosphate buffer solutions at a pH of 7.4, incubated in a shaking water bath (Medline BS-21, Korea) at 37°C for 6 h. At specific time intervals, samples were taken from the dissolution media and nanospheres were precipitated using a magnet, and the 5-FU content was determined by UV-VIS studies at 266 nm. The samples taken were added back to the dissolution media, to maintain a constant volume. From the absorbance values, the percentage of the amount cumulatively released was determined.
Results and discussion
Characterization of nanospheres
The thermal analysis was carried out with a differential scanning calorimeter (DSC), and the results have been presented in . The MC and CS used in this study showed a Tg of 108°C and 150°C, respectively. However, for A1e nanospheres, Tg was found to be 117°C. This result could be evidence of blending of MC and CS (CitationRokhade et al. 2007). The thermogram of 5-FU showed a sharp peak at 282°C, indicating the melting of the drug. In addition, A1 and A1e nanospheres revealed a Tg of 138°C and 117°C respectively. In case of 5-FU loaded nanospheres, there was no peak corresponding to 5-FU, which indicated the amorphous dispersion of 5-FU in the nanospheres.
Figure 1. DSC diagrams of (a) pristine MC, (b) pristine CS, (c) A1e formulated empty nanospheres, (d) A1 formulated nanospheres, and (e) pure 5-FU.
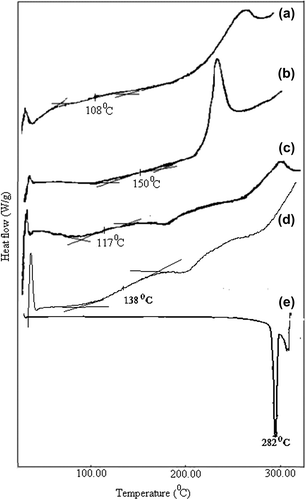
The FTIR spectra of pure CS, MC, Fe3O4, placebo CS/MC nanospheres, 5-FU-loaded CS/MC nanospheres, and 5-FU, have been presented in . In the spectrum of Fe3O4, the peak at 598 cm− 1 was assigned to the Fe–O band. A band at 3472 cm− 1 was due to N–H and O–H stretching vibrations. Bands at 1662 cm− 1 and 1380 cm− 1 were due to amide groups. Besides, the peaks at 2926 cm− 1 and 2856 cm− 1 corresponded to aliphatic C–H stretching; the C–O group was indicated by a band at 1078 cm− 1. In the FTIR spectra of MC, the band at 3472 cm− 1 was due to O–H stretching vibration, bands at 2929 cm− 1 and 2844 cm− 1 corresponded to aliphatic C–H stretching, and the band at 1061 cm− 1 was assigned to the C–O group. These peaks were also observed in the spectra of empty CS/MC nanospheres (CitationRokhade et al. 2007). For 5-FU, the band at 1250 cm− 1 was assigned as the characteristic C–F stretching vibration. A similar peak was also observed at 1324 cm− 1 in the spectrum of the 5-FU-loaded nanospheres. Moreover, the absence of such a signal in the FTIR spectrum of the empty nanospheres, confirmed the presence of 5-FU in the nanospheres.
Figure 2. FTIR spectrum of (a) magnetite, (b) pristine CS, (c) pristine MC, (d) A1e, (e) pure 5-FU, and (f) A1-formulated nanosphere.
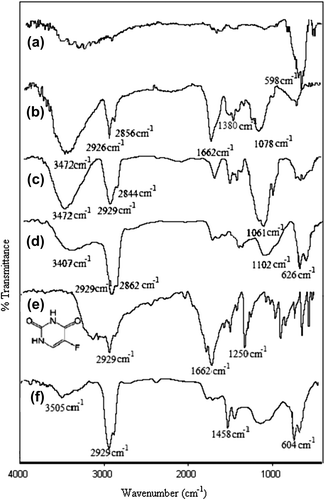
The zeta potentials of magnetite, empty (D3e) and drug-loaded (D3) nanospheres are given in . As seen, the zeta potential of the magnetite nanoparticles was nearly zero mV, which is probably due to particle aggregation. The empty nanospheres, on the other hand, revealed a zeta potential of about− 50 mV, due to the –OH groups of CS. Compared to the empty nanospheres, the drug-loaded nanospheres revealed higher zeta potential values (between − 50 and 0 mV), which could be related to the enhancement of the positive charges in the drug-loaded nanospheres.
The particle size distribution of empty and drug-loaded nanospheres have been presented in . The average particle size of empty and drug-loaded nanospheres were calculated as 232.5 nm and 306.8 nm, respectively.
X-ray diffractograms of (a) magnetite, and (b) A1e nanospheres have been presented in . The XRD spectrum of magnetite revealed the peaks at 2θ: 35.620, 56.820, and 62.780. The XRD spectrum of the A1e nanospheres showed very similar peaks at 2θ: 35.80 0, 57.420, and 62.800, which provided a solid proof for the presence of magnetite particles in the CS/MC polymer matrix.
The morphology of nanospheres were determined by taking high resolution TEM (HRTEM) images, and they are shown in . These images clearly demonstrate that the magnetic particles are incorporated in the cores of CS/MC nanospheres (CitationYang et al. 2006).
In vitro release studies
In this study, 5-FU was added to the nanospheres by following two different procedures.
The studies of release of 5-FU from the nanospheres were carried out using both the procedures.
Surface adsorption of 5-FU
displays the amount of 5-FU adsorbed as a function of adsorption time. As seen, the adsorption capacity increased with the increase in the amount of drug in the solution. 5-FU adsorption on CS/MC nanoparticles followed the Langmuir adsorption isotherm, which is presented in . Langmuir adsorption constants a, and b, were calculated as 158.7 and 0.003, respectively, using the following equation:
where Ce is the equilibrium drug concentration.
Figure 7. Change of adsorption capacity with time (◊: 0.077 M 5-FU solution, : 0.038 M 5-FU solution, Δ: 0.0192 M 5-FU solution), (crosslinking concentration: 0 042 M, exposure time to crosslinking: 5 min, percent of magnetite: 67%, CS/MC (w/w): 1/1, temperature: 37°C).
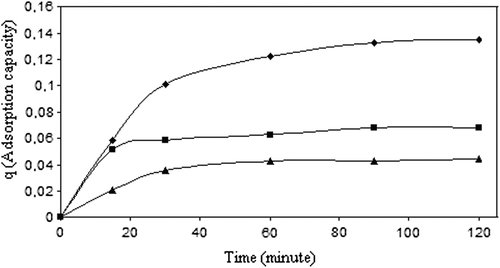
Figure 8. The curve of the adsorption isotherm (crosslinking concentration: 0.058 M, exposure time to crosslinking: 5 min, percent of magnetite: 67%, adsorption time: 2 h, CS/MC (w/w): 1/1).
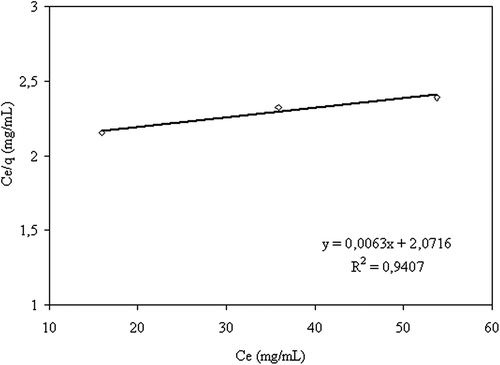
A schematic representation of the synthesis of IPN nanospheres from CS/MC polymers has been shown in , and the cross-linking reaction of these polymers with GA has been given in .
Effect of GA concentration and exposure time to GA on the release of 5-FU
The effect of crosslinking concentration on the release of 5-FU has been presented in , for drug-loaded nanospheres prepared through the encapsulation and adsorption processes, respectively. As seen from the , the percentage of cumulative 5-FU release from the nanospheres increased with an increase in the concentration of GA from 0.023 M to 0.11 M. The reason for this unusual result might be attributed to the hydrogen bonding between GA and –N and –F groups of 5-FU. The release results were supported by the entrapment efficiency results given in , which confirm that the percentage of entrapment efficiency increased with an increase in the crosslinking concentration. A comparison of the results, given in , shows that the release of 5-FU from the encapsulation technique (64%), is almost twice that of the release in the adsorption technique (36%).
Figure 10. Effect of GA concentration on the 5-FU release in (a) encapsulation, and (b) adsorption processes. (□: A1, ◊: A3, □: A5), (CS/MC ratio (w/w): 1/1, exposure time to GA: 5 min, drug/polymer ratio: 1/8, magnetite content: 67%).
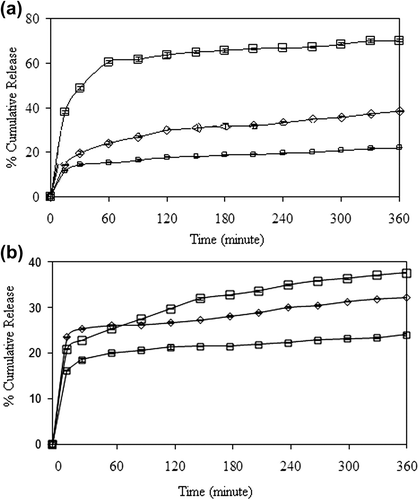
Table III. The change of the entrapment efficiency with the cross-linking concentration.
The effect of exposure time to GA on the release of 5-FU was investigated at exposure times of 5, 15 and 30 min, for both the encapsulation and adsorption processes. The results are presented in , which clearly indicate that with increasing exposure time to GA (5–15 min.), the release rate decreased for both processes. Such lowering in the cumulative release might be due to the increase in crosslinking density of the nanospheres. As a result of the reduction in the free volume, the penetration of water molecules and the diffusion of 5-FU molecules became difficult. Similar results were also found in the literature (CitationŞanlı and Kondolot Solak 2009, CitationKulkarni et al. 1999). As seen from , the percentage of cumulative release decreased with an increase in the exposure time to GA, from 5–30 min, similar to other processes. Şanlı and Kondolot Solak studied the controlled release of naproxen from sodium alginate and poly(vinyl alcohol)/sodium alginate-blended beads crosslinked with glutaraldehyde. They have investigated the effect of the time of exposure to GA on the release rate of naproxen sodium at 5, 10, and 15 min, and they have reported that an increase in exposure time to GA (5–15 min) decreased the cumulative release (CitationŞanlı and Kondolot Solak 2009).
Effect of magnetite content on the release of 5-fluorouracil
The effect of the magnetite content on the release of 5-FU was investigated by preparing nanospheres with different magnetite ratios. The results have been presented in , for both encapsulation and adsorption processes, respectively. It can be seen from the figures that the cumulative drug release increased with the increase in the magnetite content of the nanospheres. For both processes, the highest drug release was recorded for the nanospheres that contain 100% magnetite. Thus, rest of the study was carried out using a 100% magnetite content. The cumulative release from nanospheres was found to be 74% and 40% at the end of 6h, for encapsulation and adsorption processes, respectively.
Effect of the CS/MC ratio on the drug release
We have researched the effect of polymer ratio on the release of 5-FU in both processes. The results have been given in . The maximum cumulative release of 5-FU was obtained in formulation D3, as 100% at the end of 6 h, for the encapsulation process. The results could be explained by the fact that as the CS content of the polymer matrix increased the swelling of the matrix, due to the hydrophilic nature of CS. The results obtained are in good consistency with the swelling results (). As reflected in , the equilibrium swelling degree increased as the CS content of the nanospheres increased. When the swelling degree of the nanospheres increased, the free volume in the matrix increased. Hence, penetration of the liquid molecules through the free volume increased, which increased the diffusion of the drug to the dissolution media.
Figure 13. Effect of CS/MC ratio on 5-FU release at (a) encapsulation and (b) adsorption processes (Δ: C2,□: D3), (crosslinking concentration: 0.1 M, exposure time to GA: 5 min, magnetite content: 100%, drug/polymer ratio:1/8).
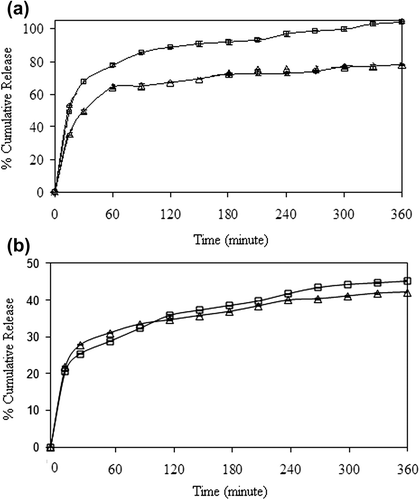
Table IV. Equilibrium swelling degrees for nanospheres.
Effect of the drug/polymer ratio on the release of 5-fluorouracil
The effect of drug/polymer ratio on the release of 5-FU release has been presented in . The cumulative release of 5-FU from nanospheres increased with a decrease in the drug/polymer ratio from 1/8 to 1/16. The lower drug content might lead to easier penetration of liquid through the nanospheres, and then a faster 5-FU diffusion could occur from the nanospheres. In the other words, while 5-FU content of the nanospheres decreased, loose structure in the polymeric nanospheres was formed, through which liquid and 5-FU could easily penetrate and diffuse (CitationKumbar and Aminabhavi 2003; CitationSoppimath and Aminabhavi 2002; CitationKumbar et al. 2003; CitationMc Gann et al. 2009). The highest cumulative release was found to be 100%, for the drug/polymer ratio of 1/16.
Results of percentage entrapment efficiency of CS/MC nanospheres
The results of entrapment efficiency (%) values have been shown in . Entrapment efficiencies were found to be dependent on the conditions of nanosphere preparation, such as the CS/MC ratio, magnetite content, GA concentration, and exposure time to GA. The results of the percentage entrapment efficiency increased from 27.91 to 96.2, as crosslinking concentration was increased from 0.023 M to 0.11 M. On the contrary, the results of percentage entrapment efficiency decreased with an increase in the exposure time to GA. In accordance with the literature (CitationIŞıklan 2006), the effect of the exposure time to GA and concentration of GA had a similar effect on the 5-FU release, for both processes.
Analysis of kinetic results
The results of k, n and r, calculated from Eq. (3), have been given in . From these data, the n values are found to range between 0.1029 and 0.2860. The results indicate that 5-FU release from nanospheres displayed Case I transport for both processes.
Table V. The k, n, and r values of CS/MC nanospheres, prepared with encapsulation and adsorption processes.
Conclusion
In this study, 5-FU, as a model anticancer drug, was successfully loaded onto CS/MC IPN nanospheres, using both encapsulation and adsorption processes. The release study demonstrated that the 5-FU release, the percentage of entrapment efficiency, and the values of equilibrium swelling degree increased with increase in the GA concentration and the content of magnetite, whereas they decreased with the exposure time to GA, for both processes. According to the release data, all formulations followed the Fickian mechanism. The 5-FU release from nanospheres was found to be 46% for the adsorption process, whereas the drug release was found to be 100% for the encapsulation process, at the end of 6 h. Further experiments need to be conducted, to clarify the cytotoxicity of the CS/MC magnetite-containing nanospheres.
Acknowledgment
We are grateful to Gazi University Research Fund for the support of this study.
Declaration of interest
The authors report no declarations of interest. The authors alone are responsible for the content and writing of the paper.
References
- Babu VR, Rao KSV, Sairam M, Naidu BV, Hosamani KM, Aminabhavi TM. 2006. pH-sensitive interpenetrating network microgels of sodium alginate-acrylic acid for the controlled release of ibuprofen. J Appl Polym Sci. 99:2671–2678.
- Bulte JWM, Kraitchman DL. 2004. Iron oxide MR contrast agents for molecular and cellular imaging. NMR Biomed. 17:484–499.
- Butoescu N, Seemayer CA, Foti M, Jordan O, Doelker E. 2009. Dexamethasone-containing PLGA superparamagnetic microparticles as carriers for the local treatment of arthritis. Biomaterials. 30:1772–1780.
- Chassary P, Vincent T, Guibal E. 2004. Metal anion sorption on chitosan and derivative materials: a strategy for polymer modification and optimum use. React Funct Polym. 60:137–149.
- Ciofani G, Riggio C, Raffa V, Menciassi A, Cuschieri A 2009. A bi-modal approach against cancer: magnetic alginate nanoparticles for combined chemotherapy and hyperthermia. Med Hypotheses. 73:80–82.
- Dorniani D, Bin Husscin MZ, Kura AU, Fakurazi S, Shaari AH, Ahmad Z. 2012. Preparation of Fe3O4 magnetic nanoparticles coated with gallic acid for drug delivery. Int J Nanomed. 7:5745–5756.
- Gan ZF, Jiang JS, Yang Y, Du B, Qian M, Zhang P. 2008. Immobilization of homing peptide on magnetite nanoparticles and its specificity in vitro. J Biomed Mater Res. 84:10–18.
- Gu HW, Xu KM, Xu CJ, Xu B. 2006. Biofunctional magnetic nanoparticles for protein separation and pathogen detection. Chem Commun. 12:941–949.
- Guo SJ, Li D, Zhang LX, Li J, Wang E. 2009. Monodisperse mesoporous superparamagnetic single-crystal magnetite nanoparticles for drug delivery. Biomaterials. 30:1881–1889.
- Gupta AK, Gupta M. 2005. Synthesis and surface engineering of iron oxide nanoparticles for biomedical applications. Biomaterials. 26:3995–4021.
- Huang L, Sui W, Wang Y, Jiao Q. 2010. Preparation of chitosan/ chondroitin sulfate complex microcapsules and application in controlled release of 5-fluorouracil. Carbohyd Polym. 80:168–173.
- Işıklan N. 2006. Controlled release of insecticide carbaryl from sodium alginate, sodium alginate/gelatin and sodium alginate/sodium carboxymethyl cellulose blend beads crosslinked with glutaraldehyde. J Appl Polym Sci. 99:1310–1319.
- Işıklan N, İnal M, Yiğitoğlu M. 2008. Synthesis and characterization of poly(N-vinyl-2-pyrrolidone) grafted sodium alginate hydrogel beads for the controlled release of indomethacin. J Appl Polym Sci. 110:481–493.
- İnal M, Yiğitoğlu M, Işıklan N. 2008. Controlled release of indomethacin from crosslinked alginate bead. e-Polymers. 8:177–192.
- Jing XH, Yang L, Duan XJ, Xie B, Chen W, Li Z, Tan HB. 2008. In vivo MR imaging tracking of magnetic iron oxide nanoparticle labeled, engineered, autologous bone marrow mesenchymal stem cells following inrra-articular injection. Jt Bone Spine. 75:432–438.
- Kulkarni AR, Soppimath KS, Aminabhavi TM. 1999. Controlled release of diclofenac sodium from sodium alginate beads crosslinked with glutaraldehyde. Pharm Acta Helv. 74:29–36.
- Kumbar SG, Soppimath KS, Aminabhavi TM. 2003. Synthesis and characterization of polyacrylamide-grafted chitosan hydrogel microspheres for the controlled release of indomethacin. J Appl Polym Sci. 87:1525–1536.
- Kumbar SG, Aminabhavi TM. 2003. Synthesis and characterization of modified chitosan microspheres: effect of the grafting ratio on the controlled release of nifedipine through microspheres. J Appl Poly Sci. 89:2940–2949.
- Kuroiwa T, Noguchi Y, Nakajima M, Sato S, Mukataka S, Ichikawa S. 2008. Production of chitosan oligosaccharides using chitosanase immobilized on amylose-coated magnetic nanoparticles. Process Biochem. 43:62–69.
- Lee E, Lee J, Lee IH, Yu M, Kim H, Chae SY, Jon S. 2008. Conjugated chitosan as a novel platform for oral delivery of paclitaxel. J Med Chem. 51:6442–6449.
- Li G, Jiang Y, Huang K, Ding P, Chen J 2008. Preparation and properties of magnetic Fe3O4-chitosan nanoparticles. J Alloy Compd. 466:451–456.
- Loh JW, Yeoh G, Saunders M, Lim LY. 2010. Uptake and cytotoxicity of chitosan nanoparticles in human liver cells. Toxicol Appl Pharm. 249:148–157.
- Loh JW, Saunders M, Lim LY. 2012. Cytotoxicity of monodispersed chitosan nanoparticles against the Caco-2 cells. Toxicol Appl Pharmacol. 262:273–282.
- Lu A, Salabas EL, Schuth F. 2007. Magnetic nanoparticles: synthesis, protection, functionalization and application. Angew Chem Int Ed Engl. 46:1222–1244.
- Mao C, Zhu JJ, Hu YF, Ma QQ, Qiu YZ, Zhu AP, et al. 2004. Surface modification using photocrosslinkable chitosan for improving hemocompatibility. Colloid Surface B. 38:47–53.
- Mc Gann MJ, Higginbotham CL, Geever LM, Nugent MJ. 2009. The synthesis of novel pH-sensitive poly(vinyl alcohol) composite hydrogels using a freeze/thaw process for biomedical applications. Int J Pharm. 372:154–161.
- Mody VV, Cox A, Shah S, Singh A, Beving W, Parihar H. 2014. Magnetic nanoparticle drug delivery systems for targeting tumor. Appl Nanosci. 4:385–392.
- Okon E, Pouliquen D, Okon P, Kovaleva ZV, Stepanova TP, Lavit SG, et al. 1974. Biodegradation of magnetite dextran nanoparticles in the rat: a histologic and biophysical study. Lab Invest. 71: 895–903.
- Olukman M, Şanlı O, Kondolot Solak E. 2012. Releasse of anticancer drug 5-fluorouracil from different ionically crosslinked alginate beads. J Biomater Nanobiotech. 3:469–479.
- Peppas NA. 1985. Analysis of Fickian and non-Fickian drug release from polymer. Pharm Acta Helv. 60:110–111.
- Qi L, Xu Z, Jiang X, Li Y, Wang M. 2005. Cytotoxic activities of chitosan nanoparticles and copper-loaded nanoparticles. Bioorg Med Chem Lett. 15:1397–1399.
- Ritger PL, Peppas NA. 1987. A simple equation for description of solute release II Fickian and anomalous release from swellable devices. J Control Release. 5:37–42
- Rokhade AP, Shelke NB, Patil SA, Aminabhavi TM. 2007. Novel interpenetrating polymer network microspheres of chitosan and methylcellulose for controlled release of theophylline. Carbohydr Polym. 69:678–687.
- Saikia C, Hussain A, Ramteke A, Sharma HK, Maji TK. 2014. Carboxy methyl starch-chitosan-coated iron oxide magnetic nanoparticles for controlled delivery of isoniazid. Artif Cell Nanomed B. 4:1–11.
- Soppimath KS, Aminabhavi TM. 2002. Water transport and drug release study from cross-linked polyacrylamide grafted guar gum hydrogel microspheres for the controlled release application. Eur J Pharm Biopharm. 53:87–98.
- Şanlı O, Kondolot Solak E. 2009. Controlled release of Naproxen from Sodium Alginate and Poly(vinyl alcohol)/Sodium Alginate Blend Beads Crosslinked with Glutaraldehyde. J Appl Polym Sci. 112:2057–2065
- Trapani A, Sitterberg J, Bakowsky U, Kissel T. 2009. The potential of glycol chitosan nanoparticles as carrier for low water soluble drugs. Int J Pharm. 375:97–106.
- Xu Y, Lin Y, Zhuang L, Lin J, Lv J, Huang Q, Sun J. 2013. Bleomycin loaded magnetite nanoparticles functionalized by polyacrylic acid as a new antitumoral drug delivery system. Biomed Res Int. 2013:462589.
- Yang J, Park SB, Yoon HG, Huh YM, Haam S. 2006. Preparation of poly ε-caprolactone nanoparticles containing magnetite for magnetic drug carrier. Int J Pharm. 324:185–190.
- Yao Q, Liu W, Gou XJ, Gou XQ, Yan J, Song Q, et al. 2013. Preparation, characterization and cytotoxicity of various chitosan nanoparticles. J Nanomat. 2013:ID:183871.
- Zhang Y, Huo M, Zhou J, Yu D, Wu Y. 2009. Potential of amphiphilically modified low molecular weight chitosan as a novel carrier for hydrophobic anticancer drug: Synthesis, characterization, micellization and cytotoxicity evaluation. Carbohyd Polym. 77:231–238.
- Zinutti C, Kedzierewicz F, Hoffman M, Benoit JP, Maincent P. 1996. Influence of the casting solvent on the physico-chemical properties of 5-fluorouracil-loaded microspheres. Int J Pharm. 133:97–105.