Abstract
This study deals with the development of interpenetrating polymer network (IPN) microspheres of acrylamide (AAm) grafted onto a chitosan (CS) backbone and methylcellulose (MC). Chitosan-graft-polyacrylamide (CS-g-PAAm) was synthesized by cerium (IV) ammonium nitrate-induced free radical graft polymerization. The grafting percentage was found to be 50.58%. The synthesized graft copolymer and MC were used to prepare microspheres by the water-in-oil (w/o) emulsion-crosslinking method, and crosslinked with glutaraldehyde (GA) as drug delivery matrices of ibuprofen (IBU). The release of IBU from microspheres decreased when the amount of CS-g-PAAm in the polymer matrix and amount of crosslinker added were increased, while it increased with the increase of the IBU/polymer ratio.
Introduction
Biodegradable and biocompatible polymer grafting and its applications have attracted considerable attention in the field of drug delivery (CitationFu et al. 2007, CitationKumbar et al. 2003, CitationRokhade et al. 2007a). The polymers used are fundamentally modified and tailored to target drug delivery applications, in conjunction with the aim of increasing therapeutic effects, develop new therapeutic strategies, reduce adverse effects, and improve patient compliance (CitationKaity et al. 2013). Among various polymeric systems investigated in drug delivery applications, attention has been focused on natural polymers as well as a combination of both types of polymers because they are nontoxic, biodegradable, and low cost (CitationBulut and Şanlı 2014). However, the major disadvantages of several natural biopolymers are their poor mechanical properties as a result of extensive swelling, and microbial contamination (CitationKrishna Rao et al. 2006, CitationŞanlı et al. 2008). Therefore, the properties of natural polymers have been modified by blending (CitationRamesh Babu et al. 2007), grafting (CitationIşıklan et al. 2011, CitationYuan et al. 2008), and developing interpenetrating polymer networks (IPN) (CitationAngadi et al. 2010, CitationBanerjee et al. 2012, CitationBulut 2014). CitationYuan et al. (2008) prepared a stimulus-responsive magnetic nanoparticle drug carrier from chitosan-g-poly(N-isopropylacrylamide-co-N,N-dimethylacrylamide) (a thermosensitive smart polymer) for controlled drug release. They have reported that the smart polymer combines the stimulus-responsive behavior of both the poly(N-isopropylacrylamide-co-N,N-dimethylacrylamide) polymer and chitosan (CS), with improved biodegradability. CitationBanerjee et al. (2012) studied the controlled release of a non-steroidal anti-inflammatory drug (diclofenac sodium) from IPN hydrogel microspheres of sodium carboxymethyl cellulose (NaCMC) and poly(vinyl alcohol) (PVA), and reported that the microspheres formed were useful as controlled release devices to control the release rates of diclofenac sodium through the polymeric matrices developed.
CS, which is a biomaterial, is a cheap and abundant linear polysaccharide, composed of randomly distributed (1,4)-linked D-glucosamine (deacetylated) and N-acetyl-D-glucosamine (acetylated) units, is one of the most well-known polymers because of wide applications in the area of controlled release delivery (CitationLi et al. 2011). Due to its properties such as good biocompatibility, low toxicity, biodegradability (CitationSinha et al. 2004), and other intrinsic properties (e.g. hydrophilicity, functional amino groups, and a net cationic charge) (CitationLiang et al. 2011), CS has been formulated into microspheres (CitationMallikarjuna Reddy et al. 2006, CitationRokhade et al. 2007b, CitationŞanlı et al. 2009), membranes (CitationThacharodi and Panduranga Rao 1993, CitationWang et al. 2011), and tablets (CitationHuanbutta et al. 2013) for drug delivery applications. On the other hand, CS is a weak base and is insoluble in neutral or basic conditions; however, it is soluble in acidic pH media (pH ˂ 6.5), which can convert the glucosamine units into a soluble form, R–NH3+ (CitationSinha et al. 2004). This is considered a major negative characteristic for CS, and limits its use in some applications (CitationNajafabadi et al. 2014). Therefore, the graft copolymerization of CS with monomers such as AAm is an effective way of improving its performance and solubility. Morever, drug release from polymeric structures such as CS can be controlled and enhanced by the addition of another water-soluble polymer such methylcellulose (MC) (CitationRokhade et al. 2007b). MC, a natural water-soluble carbohydrate polymer (CitationSullad et al. 2010), finds applications as a binder or thickener in the pharmaceutical, food, and ceramic processing industries (CitationBabu et al. 2007). It can form reversible physical gels due to hydrophobic interactions when it is heated above room temperature (CitationSullad et al. 2010), and can be chemically crosslinked with a dialdehyde in the presence of a strong acid to form a hydrogel (CitationHorkay and Zrinyi 1982, CitationPark et al. 2001).
Ibuprofen (IBU), which is a well-known prominent non-steroidal anti-inflammatory drug, has been widely used in the treatment of various musculoskeletal disorders and painful conditions. IBUdissolves in an alkaline solution and ethanol (CitationMundargi et al. 2008), but it is poorly soluble in water (≈1 mg/mL at 22°C) (CitationBulut 2014). Due to its short plasma half-life of 1–3 h, and gastrointestinal side effects ranging from mild dyspepsia to gastric bleeding, IBU is an ideal candidate for preparing controlled release formulations (CitationArica et al. 2005, CitationMundargi et al. 2008, CitationValot et al. 2009). In previous work, CS/poly(vinyl alcohol) IPN microspheres containing IBU have been prepared, to achieve a controlled drug release profile suitable for oral administration (CitationBulut 2014). In this study, the acrylamide (AAm) monomer was grafted onto CS, and then IPN microspheres of CS-g-PAAm and MC were developed by a water-in-oil (w/o) emulsion crosslinking method using glutaraldehyde (GA) as a crosslinker, in various preparation conditions. The entrapment efficiency and particle size of the microspheres were evaluated. IBU release was carried out at three different pH values (1.2, 6.8, and 7.4). The release characteristics of the IPN microspheres were studied in detail for the amount of crosslinker, IBU/polymer ratio, and different ratios of CS-g-PAAm and MC.
Materials and methods
Materials
IBU was received as a gift from Atabay Co. (Turkey). CS (highly viscous) and MC were purchased from Sigma Chemical Co. (St. Louis, USA). Liquid paraffin was supplied by Aklar Chemistry Co. (Turkey). Acrylamide, GA (25% w/w) solution, Span 80 (a commercial surfactant), acetic acid, hydrochloric acid, n-hexane, acetone, Na2HPO4, and NaH2PO4 were all supplied from Merck (Darmstadt, Germany). Cerium (IV) ammonium nitrate (CAN) was supplied by Sigma-Aldrich Chemical Co. (St. Louis, USA).
Synthesis of the graft copolymer of CS
The graft copolymer of CS with AAm was carried out under a nitrogen atmosphere in a 250 mL three-necked flask equipped with a reflux condenser, a stirrer, and a gas inlet system, immersed in a constant temperature bath (50°C). Briefly, 2 g of CS was dissolved in 100 mL of a 2% (w/v) aqueous acetic acid solution in hot water at 50°C, with constant stirring. The solution of CS was degassed under a slow stream of nitrogen gas for 15 min. After that, the AAm solution was added to this CS solution and mixed for 15 min under a slow stream of nitrogen gas. At the end of this period, cerium (IV) ammonium nitrate (CAN) at the required concentration was added slowly to the reaction mixture. A continuous supply of nitrogen was maintained throughout the polymerization reaction period. The grafting reaction was carried out for 6 h at a temperature of 50°C. At the end of 6 h, the reaction solution was added into an excess amount of acetone to precipitate the polymer, which was then filtered through the suction and dried in a vacuum oven (Medcenter, Einrichtungen GmbH, Germany) at 50°C. The scheme of the possible reaction between CS and AAm has been presented in . The percentage grafting was calculated according to the following equation:
Where Wo and Wg denote the weights of the ungrafted CS and grafted CS, respectively.
Preparation of the IPN microspheres
The IPN microspheres of CS-g-PAAm with MC containing IBU were prepared by the w/o emulsion method, with different amounts of GA as the crosslinking agent. CS-g-PAAm (1% (w/v) in 2% (w/v) aqueous acetic acid solution), MC (2% (w/v) in water), and a known amount of IBU were mixed and stirred well for proper mixing for 12 h. The drug-loaded polymer mixture was emulsified in light liquid paraffin in a ratio of 1/5 (v/v) containing 2% (w/v) Span 80, by magnetic stirring (Nuve, Turkey) at 400 rpm for about 10 min. Then, different quantities of GA were added slowly to this emulsion, and stirring was continued for 2 h. The microspheres formed were collected by filtration, washed with n-hexane, and then dried completely in an oven (Memmert, Germany) at 40°C. Unloaded microspheres were prepared in a similar way, without IBU. Different variables, such as the varying ratios of CS-g-PAAm/MC, IBU/polymer, and the amount of crosslinker were considered in the formulation of the IPN microspheres, which have been listed in . A schematic representation of the formation of IPN has been given in .
Table I. Preparation conditions and characterization of the IBU-loaded microspheres.
Fourier transform infrared spectroscopy studies
Fourier transform infrared (FTIR) spectra measurements of IBU, CS, MC, CS-g-PAAm copolymer, and IBU-loaded CS-g-PAAm-MC microspheres were taken with a Perkin-Elmer BX-II (Germany) spectrometer, to confirm the grafting reaction between CS and AAm, and to investigate the presence of crosslinking in CS-g-PAAm-MC. FTIR spectra of samples were recorded within the wavelength region of 400–4000 cm− 1 using KBr disks at ambient temperature.
Differential scanning calorimetry studies
Differential scanning calorimetry (DSC) analysis was performed for IBU, CS, CS-g-PAAm copolymer, IBU-loaded CS-g-PAAm-MC microspheres, and empty CS-g-PAAm-MC microspheres, with a differential scanning calorimeter (Shimadzu, Japan). Sample weights ranged from 5 to 8 mg. They were heated from 30°C to 400°C at a heating rate of 10°C/min.
Scanning electron microscopy studies
Scanning electron microscopy (SEM) photographs were recorded using a LEO 1430 VP (Germany) scanning electron microscope to examine the morphology and surface structure of the microspheres at the required magnification at room temperature. The microspheres were deposited on a brass holder and sputtered with a thin coating of gold, under vacuum.
Microsphere size measurements
The particle size of the microspheres was measured using optical microscopy (Olympus CH20BIMF200, Japan).
Ibuprofen content
The IPN microspheres of a known amount were crushed to get a powder, in an agate mortar with a pestle, and the polymeric powder was refluxed with 100 ml of a pH 7.4 phosphate buffer solution for 4 h to ensure the complete extraction of IBU from the microspheres. At the end of 4h, the precipitated polymer was filtered and the clear solution was analyzed with an UV-VIS spectrophotometer (Shimadzu UV-1700 Pharma) at a wavelength of 223 nm, with a phosphate buffer solution of pH 7.4 as a blank. The entrapment efficiency (%) was then calculated as follows:
Swelling studies
The equilibrium swelling of the crosslinked empty IPN microspheres was determined by the measurement of the extent of their swelling at two different pH values (1.2 and 7.4) at 37°C. To ensure complete equilibration, the microsphere samples were allowed to swell for 24 h, and then filtered. The excess surface-adhered liquid drops were removed by blotting with a soft tissue paper for a few seconds. The swollen microspheres were weighed and then dried in an oven at 40°C, until there was no change in the dried mass of the samples. The equilibrium swelling degree (%) was calculated as follows:
In vitro ibuprofen release
IBU release from the IPN microspheres under varying ratios of CS-g-PAAm/MC (w/w), IBU/polymer (w/w), and the amount of crosslinker added, were investigated in 100 ml of HCl (pH 1.2) and phosphate buffer solutions (pH 6.8 and 7.4) incubated in a shaking water bath (Daihan WSB-18, Korea), at a speed of 100 rpm at 37°C. At 2 h intervals, the pH of the IBU release medium was changed from 1.2 to 6.8, and then to 7.4. At specific time intervals, 2 mL of the solution was withdrawn and the IBU content was determined with an UV spectrophotometer at 223 nm. Experiments were performed in triplicate to minimize the variational error. The average values were used for further data treatment and plotting. Equal volumes of fresh HCl or phosphate buffer solution were added to the release medium, to maintain a constant volume.
Results and discussion
Characterization of the CS-g-PAAm copolymer and the IPN microspheres
Graft copolymerization of CS with AAm was achieved by CAN-induced free-radical graft polymerization, and the scheme of the possible reaction between CS and AAm has been presented in . The percentage grafting of AAm onto CS was up to 50.58%. The FTIR spectral data were used to characterize the CS-g-PAAm copolymer. The data were also utilized to investigate the presence of crosslinking in the CS-g-PAAm-MC matrix and the chemical stability of the IBU in the IPN microspheres. FTIR spectra of CS (a) and grafted CS (b) are shown in . The IR spectra of CS exhibit characteristic peaks around 3367, 2928, and 1084 cm− 1, indicating the stretching of O–H, aliphatic C–H, and C–O, respectively. The amine group of CS has a characteristic peak in the region of 3000–3500 cm− 1, which has been masked by the peak due to the –OH group (CitationŞanlı et al. 2009). Overlapped peaks around 1639 and 1560 cm− 1 indicate amide bands and the symmetrical stretching vibration absorption of the amino group, respectively (CitationJoshi and Sinha 2007, CitationPeng et al. 2010). The observed sharp peak at 1408 cm− 1 is assigned to stretching vibrations of C–N bond (CitationPeng et al. 2010). The FTIR spectrum of the synthesized CS-g-PAAm shows additional bands at 1667 cm− 1 (amide 1) and 1614 cm− 1 (amide II), due to grafted PAAm chains at CS (CitationSingh et al. 2009, CitationSokker et al. 2011). A broad band around 3356 cm− 1 could be attributed to the O–H stretching vibration of the hydroxyl group of CS-g-PAAm. Moreover, a new peak that appeared at 3193 cm− 1 corresponds to the bonded –NH stretching vibrations (CitationKumbar et al. 2003). These FTIR spectra demonstrate that AAm was successfully grafted onto the chitosan backbone.
FTIR spectra of MC, Cs-g-PAAm, IBU, and IBU-loaded CS-g-PAAm-MC microspheres are shown in . The spectrum of CS-g-PAAm was discussed in the previous paragraph. The MC has a characteristic broad band at 3463 cm− 1 due to the O–H stretching vibrations, and the C–H aliphatic stretching vibrations are shown at 2933 and 2829 cm− 1 (CitationRokhade et al. 2007b, CitationSullad et al. 2010). For IBU, typical O–H stretching, and a sharp peak corresponding to the C = O stretching are observed at 2955 and 1720 cm− 1, respectively (CitationMaheshwari et al. 2003, CitationSogias et al. 2012). A number of peaks observed between 1500 and 700 cm− 1 are due to the aromatic ring present in the IBU, and the characteristic peak at 1420 cm− 1 is due to aromatic C = C stretching (CitationSogias et al. 2012). IBU-loaded microspheres showed a peak at 1726 cm− 1, which is due to the peak of the carbonyl stretching of IBU observed at 1720 cm− 1. This indicates the chemical stability of the drug in the grafted CS-MC polymeric microspheres. In addition, the overlapped peak at 1665 cm− 1 is due to the formation of an imine moiety as a result of the crosslinking reaction between free –NH2 groups of the CS-g-PAAm and the aldehyde groups of GA (CitationKumbar et al. 2003). On the other hand, the band of the acetal group at 1117 cm− 1 can be due to the reaction of GA with the hydroxyl group of MC.
DSC thermograms of CS (curve a) and CS-g-PAAm (curve b) are presented in . As it was reflected from , the Tg value of the CS used in this study was found to be ∼57°C, whereas the Tg value of CS-g-PAAm was found to be ∼120°C. The value of Tg increases as the bulkiness of the side chain increases (CitationŞanlı et al. 2007). The Tg value of PAAm (Tg = 165°C) is higher than that of CS (CitationBrandrup and Immergut 1975). Therefore, the Tg value of the CS increases with the grafting of AAm onto CS. Similar results are available in literature (CitationKumbar and Aminabhavi 2003, CitationŞanlı et al. 2007).
Figure 5. (I) DSC thermograms of CS (a), CS-g-PAAm (b), (II) DSC thermograms of IBU (a), IBU-loaded microspheres (b), and empty microspheres (c).
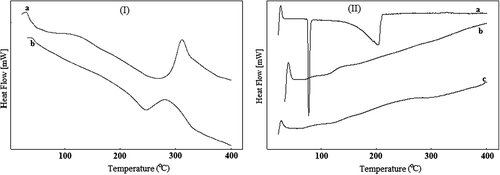
DSC thermograms of IBU (curve a), IBU-loaded CS-g-PAAm-MC microspheres (curve b), and empty CS-g-PAAm-MC microspheres (curve c) are presented in . IBU showed a sharp endothermic peak at 76°C, due to its melting. On the contrary, in the case of IBU-loaded microspheres, no characteristic peak was observed at 76°C, indicating that the drug in the microspheres was molecularly dispersed in the CS-g-PAAm-MC matrix.
SEM photographs of dried empty IPN microspheres and IBU-loaded IPN microspheres taken at 1300x, 1050 and 30000x magnification are shown in . As it is seen from the figure, the microspheres of this study are almost spherical in shape. Empty IPN microspheres show a smooth surface, whereas IBU-loaded IPN microspheres show roughness on the surface.
Figure 6. SEM micrographs at 1300 and 30,000 magnifications of the empty CS-g-PAAm-MC microspheres (a and b) and at 1050 and 30,000 magnifications of the IBU-loaded CS-g-PAAm-MC microspheres (c and d).
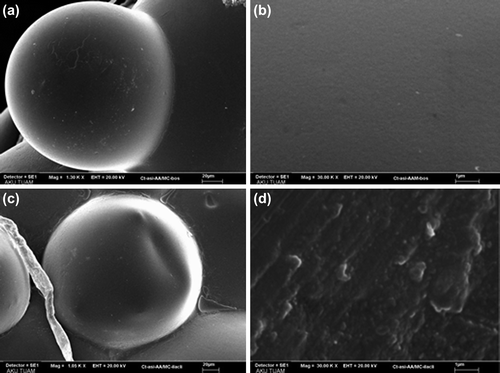
To understand the extent of crosslinking of the polymer matrix, it is important to calculate the molar mass (Mc) between the crosslinks of the polymer, and Mc significantly affects the mechanical and physical properties of the crosslinked polymer network. Equilibrium swelling is a highly useful parameter to determine the Mc of the microspheres. It can be done based on the Flory-Rehner equation (CitationFlory 1953):
The volume fraction, φ of the polymer in the swollen state is calculated as:
In the above equations, ρp and ρs are the densities of polymer and solvent, respectively; Vs is the molar volume of the solvent. Mb and Ma are the mass of polymer before and after swelling, respectively.
The interaction parameter, χ, was calculated using the following equation, based on the procedure published by CitationAithal and Aminabhavi (1990)
where N is:
and dφ/dT is the slope of the line obtained by plotting the volume fraction vs temperature (in Kelvin).
The Mc values were calculated for the IBU-loaded CS-g-PAAm-MC microspheres, and have been presented in . The Mc values increased with increasing temperature, and varied in range from 458 to 50,392. On the other hand, these values decreased with increasing amount of GA, since the polymer network would have become denser. Also, the Mc values decreased with increasing graft copolymer amount of the formulation, which supports the IBU release results. Similar results have also been reported elsewhere (CitationAgnihotri and Aminabhavi 2006, CitationIşıklan and Küçükbalcı 2012). For instance, Işıklan and Küçükbalcı prepared indomethacin-containing alginate–graft-poly(N-isopropylacrylamide) beads by crosslinking with GA. They reported that the Mc values decreased with the increasing concentration of GA in the formulation.
Table II. Mc values of the IBU-loaded CS-g-PAAm-MC IPN microspheres.
Particle size evaluation of the IPN microspheres
The particle size of the microspheres was measured using optical microscopy. The diameter of the IPN microspheres produced by changing the ratios of CS-g-PAAm and MC (w/w), IBU/polymer (w/w) ratio, and amount of GA are shown in . As can be seen from the , the microspheres formed have average particle sizes ranging from 103 ± 2.4 μm to 164 ± 1.9 μm in diameter. These results suggest that with an increasing amount of MC in the polymer matrix, the diameter of the microspheres increased for the microspheres having a 66.7% (wt) MC content, but a significant diversity was not observed for the microspheres having 33.3% and 50% (wt) MC content. CitationRokhade et al. (2007b) prepared novel IPN microspheres of CS and MC for controlled release of theophylline, and reported that as the amount of MC increased, the particle size of the microspheres increased, since a higher amount of MC produced bigger droplets during emulsification due to the increased viscosity in the polymer solution. CitationSullad et al. (2010) studied the controlled release of theophylline through the IPN-blend microspheres prepared from poly(vinyl alcohol) (PVA) and MC with different compositions, and found that with an increasing amount of MC in the blend matrix, the size of the microspheres increased. Conversely, the diameter of the microspheres showed a slight dependence on drug/polymer ratio. The diameter of the microspheres also showed a dependence on the amount of GA, and with an increase in the crosslinker amount, a decrease in the diameters of the microspheres was observed. A higher amount of GA possibly caused the shrinkage of microspheres due to the formation of a more rigid network, and an increase in crosslink density (CitationAL-Kahtani Ahmed and Sherigara 2014, CitationMallikarjuna Reddy et al. 2008).
Entrapment efficiency evaluation of the IPN microspheres
Three different IBU/polymer ratios (1/1, 1/2, and 1/4) (w/w) of IBU were loaded during crosslinking of the microspheres. The results of entrapment efficiencies (%) measurement are displayed in . An increase in entrapment efficiency (%) was observed with increasing amount of CS-g-PAAm in the microspheres, and the highest entrapment efficiency was observed as 51.73% for 66.7% (wt) CS-g-PAAm and 33.3% (wt) MC content (IBU/polymer ratio: 1/4) (C3), but for the remaining formulations, entrapment efficiency (%) ranged from 14.93% to 41.80%. Notice that the entrapment efficiency (%) decreased with an increase in the IBU/polymer ratio from 1/4 to 1/1 in the polymer matrix for all the formulations, and this can be explained as follows: when the drug/polymer ratio increases, the CS-g-PAAm-MC solution traps less IBU molecules, and thus entrapment efficiency decreases, as shown by the results obtained in our previous group studies (CitationBulut and Şanlı 2013, CitationŞanlı et al. 2008). The extent of crosslinking displayed a significant effect on entrapment efficiency (%). As the amount of GA increased, an increase in entrapment efficiency (%) was also observed due to the formation of a more rigid network, thereby causing the retention of more drug molecules during microsphere preparation. In the study by CitationAngadi et al. (2010), the controlled release of isoniazid from IPN microspheres of CS and hydroxyethyl cellulose were studied. They reported that as the concentration of crosslinking agent (GA) increases, encapsulation efficiency increases due to the formation of a rigid network. In another study, CitationRamesh Babu et al. (2006) prepared interpenetrating network microgels of sodium alginate-acrylic acid for the controlled release of IBU, and they also found similar results.
In vitro ibuprofen release studies
In vitro release experiments of IBU from crosslinked IPN microspheres was studied successively under gastric (1.2), and intestinal (6.8 and 7.4) pH conditions at 37°C. Many parameters, such as the different ratios of CS-g-PAAm/MC (w/w), IBU/polymer (w/w), and amount of GA (mL) affected the drug release of the microspheres. display the effect of these parameters in gastric (1.2), and intestinal (6.8 and 7.4) pH conditions, respectively.
Figure 7. Effect of CS-g-PAAm-MC (% w/w) ratio on the IBU release. Exposure time to GA: 2 h, amount of GA: 5 mL, (a) IBU/polymer ratio: 1/1 (A1, B1, C1), (b) IBU/polymer ratio: 1/2 (A2, B2, C2), (c) IBU/polymer ratio: 1/4 (A3, B3, C3).
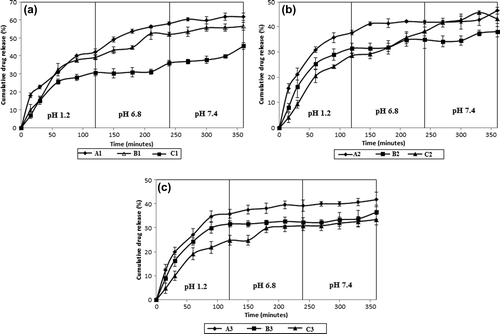
Figure 8. Effect of IBU/polymer ratio on IBU release. Exposure time to GA: 2 h, amount of GA: 5 mL, (a) IPN microsphere containing 33.3% CS-g-PAAm and 66.7% MC (A1, A2, A3), (b) IPN microsphere containing 50% CS-g-PAAm and 50% MC (B1, B2, B3), (c) IPN microsphere containing 66.7% CS-g-PAAm and 33.3% MC (C1, C2, C3).
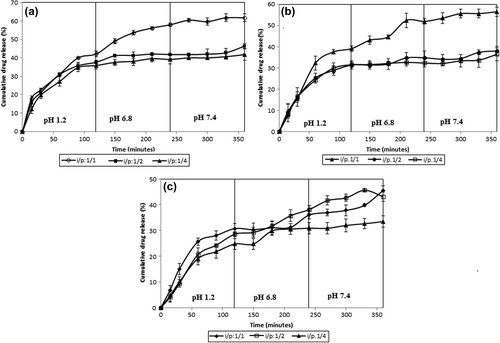
Figure 9. Effect of amount of GA on IBU release. IPN microsphere containing 66.7% CS-g-PAAm and 33.3% MC, IBU/polymer: 1/4, exposure time to GA: 2 h (C4, C5, C3).
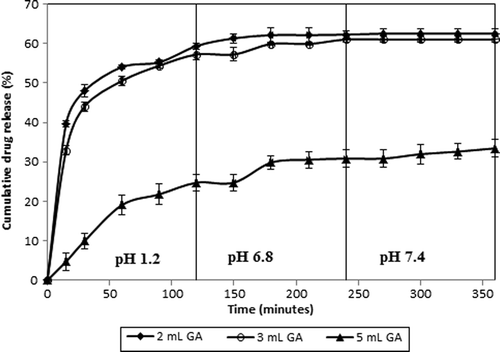
The effect of the varying ratios of CS-g-PAAm and MC (w/w) on the IBU release was studied at different ratios of CS-g-PAAm (with 1/1, 1/2, and 1/4 (w/w) IBU/polymer ratios and 5 mL of GA) for 2 h of crosslinking time. displays the cumulative IBU release of the microspheres. As it is reflected from the figures, an increase in the CS-g-PAAm amount, from 33.3% to 66.7% (wt), caused a decrease in the IBU release from the microspheres. The highest extended cumulative IBU release was found to be 62% with 33.3% CS-g-PAAm and an IBU/polymer ratio of 1/1 microspheres at the end of 6 h. A higher amount of graft copolymer in the IPN microspheres was found to cause the formation of stiffer polymeric chain entanglements, thus making the penetration of liquid molecules through CS-g-PAAm-MC microspheres and then the diffusion of drug to external medium more difficult, when compared to the microspheres having lower amount of graft copolymer. The results were also supported by the swelling measurements given in . As it is seen from the , microspheres including a higher amount of graft copolymer showed lower swelling values as compared to microspheres with a lower amount of graft copolymer. On the other hand, the results were expected because of the increase in the hydrophilic character of IPN microspheres with the presence of MC. CitationRokhade et al. (2007b) investigated the release of theophylline from CS-MC IPN microspheres, and reported that with an increasing MC content of the matrix, the swelling of the matrix as well as release of the drug increased due to the more hydrophilic nature of MC. CitationSullad et al. (2010) have also found similar results.
Table III. Equilibrium swelling degree of the empty IPN microspheres of CS-g-PAAm-MC.
Another parameter affecting the IBU release from the microspheres is the drug/polymer ratio. The effect of IBU/polymer ratio on IBU release is shown in . For this purpose, the formulations in different IBU/polymer ratios (with 33.3%, 50%, and 66.7% (w/w) CS-g-PAAm amounts and 5 mL of GA) were chosen, with 2 h of crosslinking time. The release data, as shown in , demonstrated that as the amount of drug in the microspheres increased, the release of IBU increased. IBU within the polymer matrix presumably acts as an inert filler, and therefore, increases the free volume within the polymer network, and may facilite the relexation of polymer chains (CitationAgnihotri and Aminabhavi 2006). Similar results were also found in the literature (CitationBulut and Şanlı 2014, CitationMundargi et al. 2008). Mundargi et al. (CitationMundargi et al. 2008) studied controlled release of IBU from sequential IPN hydrogel microspheres of poly(methacrylic acid) and poly(vinyl alcohol). They have reported that the drug release increased with increasing amount of drug in the microspheres due to occupation of the free volume spaces inside the swollen hydrogel as an inert filler of the drug.
One of the most effective ways of changing the release rate of microspheres is to change crosslinking density of the polymer network by varying the concentration of the crosslinker. Therefore, the effect of the amount of GA on the release of IBU was investigated by varying the amount of GA from 2 to 5 mL; the release results have been presented in (66.7% CS-g-PAAm and 33.3% MC content, IBU/polymer ratio of 1/4, exposure time to GA of 2 h). clearly indicates that as the amount of GA increased from 2 to 5 mL, the release of IBU from the CS-g-PAAm-MC microspheres decreased. On the other hand, the release results shown that the release rates of IBU were similar for crosslinker amounts of 2 and 3 mL, but decreased for 5 mL. The highest cumulative IBU release was found to be 62.5% for the IPN microspheres prepared with a GA amount of 2.0 mL, while the lowest was found to be 33.4% for the IPN microspheres prepared with a GA amount of 5.0 mL. This result is due to the formation of a tighter network structure, which reduces the rate of drug release from the matrix. Similar observations have been reported in some studies in the literature (CitationAL-Kahtani Ahmed et al. 2009, CitationBulut 2014, CitationIşıklan et al. 2008). In a study by AL-Kahtani Ahmed et al. (AL-Kahtani et al. 2009), who investigated the release of diclofenac sodium from the GA-crosslinked CS-based pH-sensitive semi-IPN microspheres, it was reported that as the amount of crosslinking agent (GA) increased from 3 to 9 mL, the release rate of drug decreased. In a previous study, IPN microspheres of CS and PVA were prepared and the release characteristics of the IBU from the IPN microspheres were investigated. It was found that an increase in the amount of GA and crosslinking time results in an increase in the crosslink density of the IPN microspheres.
Kinetics modeling of drug release
The kinetics of drug release from the IPN microspheres were tested by fitting the fraction release data Mt/M∞ using the empirical equations proposed by CitationRitger and Peppas (1987).
For the Peppas model, Mt and M∞ are the amount of drug released at time t and ∞, respectively. n is an empirical parameter characterizing the release mechanism. k is a constant, incorporating structural and geometrical character of the dosage form, and Mt/M∞ is the fraction of drug released at time t. Using the least squares procedure, the values of k, n, and the correlation coefficient (r) are presented in . In spherical polymeric matrices, if n ≤ 0.43, a Fickian diffusion (case-I), if 0.43 ≤ n˂0.85, anomalous or non-Fickian transport, and if n ≥ 0.85, a case-II transport (zero order) drug release mechanism dominates. According to the data in , the n value ranged between 0.1839 and 0.9465, with a correlation coefficient close to 0.99, indicating that IBU release from the microspheres followed mainly a non-Fickian or anomalous type of transport, where drug release is governed by coupling of the drug diffusion and polymer relaxation processes (CitationPeppas 1985, CitationRitger and Peppas 1987). On the other hand, the n values for the microspheres crosslinked with 2 and 3 mL of GA are smaller than the microspheres crosslinked with 5 mL of GA. This can be due to the loose crosslinking of the polymer IPN matrix, which leads to greater swelling (CitationRokhade et al. 2007a).
Table IV. The results of k, n, and r values calculated from Eq. (8).
The diffusion coefficient (D) can be calculated for water absorption or drug release from the microspheres using the equations as follows (CitationAgnihotri and Aminabhavi 2006):
Here, M∞ is the maximum drug release, r is the radius of the dry microspheres, and ø is slope of the linear portion of the plot of Mt/M∞ vs t1/2. The relationship between D and the CS-g-PAAm-MC ratio, amount of GA, and IBU/polymer ratio is shown in . It is noticed that the D value decreased from 1.55 × 10− 14 to 0.94 × 10− 14 cm2/s with increasing the amount of GA from 2 to 5 mL. An increase in the CS-g-PAAm amount also decreased the diffusion coefficient, which is in agreement with the release results. The D values increased with increasing IBU/polymer ratio. Similar results were also obtained in the previous studies (CitationBulut 2014, CitationBulut and Şanlı 2014).
Conclusions
In the present study, a graft copolymer of CS with AAm was synthesized by free radical polymerization using cerium (IV) ammonium nitrate (CAN) as redox initiator, and IPN microspheres of MC with the synthesized graft copolymer were successfully prepared for efficient delivery of the poorly water-soluble drug, ibuprofen (IBU). IBU, an anti-inflammatory drug, was encapsulated into the CS-g-PAAm-MC polymer matrix with the highest percentage of 51.73. Empty IPN microspheres formed were almost spherical, with a smooth surface, whereas IBU-loaded microspheres showed roughness in the surface. The particle size of the IBU-loaded microspheres was observed in the range of 103–164 μm, depending upon the nature of the matrix. The entrapment efficiency and ESD were affected by the various processing parameters such as different ratios of CS-g-PAAm and MC, amount of crosslinking agent, and the IBU/polymer ratio. It was found from the release study that IBU release from the microspheres decreased with the increase in the CS-g-PAAm amount in the polymer structure and the extent of crosslinking. On the other hand, it increased with the increase in the IBU/polymer (w/w) ratio. The highest cumulative release value (%) was observed as 62.5% for the microspheres having 66.7% CS-g-PAAm and 33.3% MC content, a drug/polymer ratio of 1/4, and GA amount of 2 mL, at the end of 6 h. The drug release mechanism suggested mainly a non-Fickian or anomalous type of transport of drug through the matrices developed. Finally, this work indicates the applicability of the IPN matrices with a physical blend of biocompatible polymers such as CS-g-PAAm and MC.
Declaration of interest
The author is responsible for the content and writing of the article. The author is grateful to the Afyon Kocatepe University Scientific Research Foundation (BAP) for financial support, with the project number of 12.FENED.02, and to Sanovel Company for the supply of the drug Ibuprofen.
References
- Agnihotri SA, Aminabhavi TM. 2006. Novel interpenetraitng network chitosan-poly(ethylene oxide-g-acrylamide) hydrogel microspheres for the controlled release of capecitabine. Int J Pharm. 324:103–115.
- Aithal US, Aminabhavi TM. 1990. Sorption and diffusion of organic solvents in polyurethane elastomers. Polymer. 31:1757–1762.
- AL-Kahtani Ahmed A, Naik HSB, Sherigara BS. 2009. Synthesis and characterization of chitosan-based pH-sensitive semi-interpenetrating network microspheres for controlled release of diclofenac sodium. Carbohydr Res. 344:699–706.
- AL-Kahtani Ahmed A, Sherigara BS. 2014. Controlled release of diclofenac sodium through acrylamide grafted hydroxyethyl cellulose and sodium alginate. Carbohydr Polym. 104:151–157.
- Angadi SC, Manjeshwar LS, Aminabhavi TM. 2010. Interpenetrating polymer network blend microspheres of chitosan and hydroxyethyl cellulose for controlled release of isoniazid. Int J Bio Macromol. 47:171–179.
- Arıca B, Çalış S, Atilla P, Durlu NT, Çakar N, Kaş HS, Hıncal AA. 2005. In vitro and in vivo studies of ibuprofen-loaded biodegradable alginate beads. J Microencap. 22:153–165.
- Babu VR, Sairam M, Hosamani KM, Aminabhavi TM. 2007. Preparation of sodium alginate–methylcellulose blend microspheres for controlled release of nifedipine. Carbohydr Polym. 69:241–250.
- Banerjee S, Siddiqui L, Bhattacharya SS, Kaity S, Ghosh A, Chattopadhyay P, et al. 2012. Interpenetrating polymer network (IPN) hydrogel microspheres for oral controlled release application. Int J Bio Macromol. 50:198–206.
- Brandrup J, Immergut EH. 1975. Polymer Handbook, 2nd ed. New York: John Wiley and Sons.
- Bulut E, Şanlı O. 2013. Delivery of Alzheimer's drug donepezil hydrochloride from ionically crosslinked alginate microspheres prepared by water-in-oil emulsion technique: optimization of release conditions. Asian J Chem. 25:3993–4000.
- Bulut E. 2014. In-vitro evaluation of ibuprofen-loaded microspheres prepared from novel chitosan/poly(vinyl alcohol) interpenetrating polymer network. Polym Plast Technol Eng. 53:371–378.
- Bulut E, Şanlı O. 2014. Optimization of release conditions of Alzheimer's drug donepezil hydrochloride from sodium alginate/sodium carboxymethyl cellulose blend microspheres. J Macromol Sci B Phys. 53:902–917.
- Flory PJ. 1953. Principles of Polymer Chemistry Ithaca, NY: Cornell University.
- Fu G, Zhao J, Yu H, Liu L, He B. 2007. Bovine serum albumin-imprinted polymer gels prepared by graft copolymerization of acrylamide on chitosan. React Func Polym. 67:442–450.
- Horkay F, Zrinyi M. 1982. Studies on the mechanical and swelling behavior of polymer networks based on the scaling concept. 4. Extension of the scaling approach to gels swollen to equilibrium in a diluent of arbitrary activity. Macromol. 15:1306–1310.
- Huanbutta K, Cheewatanakornkool K, Terada K, Nunthanid J, Sriamornsak P. 2013. Impact of salt form and molecular weight of chitosan on swelling and drug release from chitosan matrix tablets. Carbohydr Polym. 97:26–33.
- Işıklan N, İnal M, Yiğitoğlu M. 2008. Synthesis and characterization of poly(N-Vinyl-2-Pyrrolidone) grafted sodium alginate hydrogel beads for the controlled release of ındomethacin. J Appl Polym Sci. 110:481–493.
- Işıklan N, İnal M, Kurşun F, Ercan G. 2011. pH responsive itaconic acid grafted alginate microspheres for the controlled release of nifedipine. Carbohydr Polym. 84:933–943.
- Işıklan N, Küçükbalcı G. 2012. Microwave-induced synthesis of alginate–graft-poly(N-isopropylacrylamide) and drug release properties of dual pH- and temperature-responsive beads. Eur J Pharm Biopharm. 82:316–331.
- Joshi JM, Sinha VK. 2007. Ceric ammonium nitrate induced grafting of polyacrylamide onto carboxymethyl chitosan. Carbohydr Polym. 67:427–435.
- Kaity S, Isaac J, Kumar PM, Bose A, Wong TW, Ghosh A. 2013. Microwave assisted synthesis of acrylamide grafted locust bean gum and its application in drug delivery. Carbohydr Polym. 98: 1083–1094.
- Krishna Rao KSV, Naidu BVK, Subha MCS, Sairam M, Aminabhavi TM. 2006. Novel chitosan-based pH-sensitive interpenetrating network microgels for the controlled release of cefadroxil. Carbohydr Polym. 66:333–344.
- Kumbar SG, Aminabhavi TM. 2003. Synthesis and characterization of modified chitosan microspheres: effect of the grafting ratio on the controlled release of nifedipine through microspheres. J Appl Polym Sci. 89:2940–2949.
- Kumbar SG, Soppimath KS, Aminabhavi TM. 2003. Synthesis and characterization of polyacrylamide-grafted chitosan hydrogel microspheres for the controlled release of indomethacin. J Appl Polym Sci. 87:1525–1536.
- Li G-Y, Zhong M, Zhou Z-D, Ding P, Li Y-J. 2011. Novel carboxymethyl chitosan microspheres for controlled delivery of chelerythrine. J Macromol Sci Part A Pure Appl Chem. 48:904–911.
- Liang Y, Deng L, Chen C, Zhang J, Zhou R, Li X, et al. 2011. Preparation and properties of thermoreversible hydrogels based on methoxy poly(ethylene glycol)-grafted chitosan nanoparticles for drug delivery systems. Carbohydr Polym. 83:1828–1833.
- Maheshwari M, Ketkar AR, Chauhan B, Patil VB, Paradkar AR. 2003. Preparation and characterization of ibuprofen–cetyl alcohol beads by melt solidification technique: Effect of variables. Int J Pharm. 261:57–67.
- Mallikarjuna Reddy K, Ramesh Babu V, Sairam M, Subha MCS, Mallikarjuna NN, Kulkarni PV, Aminabhavi TM. 2006. Development of chitosan-guar gum semi-interpenetrating polymer network microspheres for controlled release of cefadroxil. Des Monomers Polym. 9:491–501.
- Mallikarjuna Reddy K, Ramesh Babu V, Krishna Rao KSV, Subha MCS, Chowdoji Rao K, Sairam M, Aminabhavi TM. 2008. Temperature sensitive semi-IPN microspheres from sodium alginate and n-isopropylacrylamide for controlled release of 5-fluorouracil. J Appl Polym Sci. 107:2820–2829.
- Mundargi RC, Patil SA, Kulkarni PV, Mallikarjuna NN, Aminabhavi TM. 2008. Sequential interpenetrating polymer network hydrogel microspheres of poly(methacrylic acid) and poly(vinyl alcohol) for oral controlled drug delivery to intestine. J Microencapsul. 25:228–240.
- Najafabadi AH, Abdouss M, Faghihi S. 2014. Synthesis and evaluation of PEG-O-chitosan nanoparticles for delivery of poor water soluble drugs: Ibuprofen. Mater Sci Eng C. 41:91–99.
- Park J-S, Park J-W, Ruckenstein E. 2001. Thermal and dynamic mechanical analysis of PVA/MC blend hydrogels. Polymer. 42:4271–4280.
- Peng H, Xiong H, Li J, Xie M, Liu Y, Bai C, Chen L. 2010. Vanillin cross-linked chitosan microspheres for controlled release of resveratrol. Food Chem. 121:23–28.
- Peppas NA. 1985. Analysis of Fickian non-Fickian drug release from polymers. Pharm Acta Helv. 60:110–111.
- Ramesh Babu V, Sairam M, Hosamani KM, Aminabhav TM. 2007. Preparation of sodium alginate–methylcellulose blend microspheres for controlled release of nifedipine. Carbohydr Polym. 69:241–250.
- Ramesh Babu V, Krishna Rao KSV, Sairam M, Naidu BVK, Hosamani KM, Aminabhavi TM. 2006. pH sensitive interpenetrating network microgels of sodium alginate-acrylic acid for the controlled release of ibuprofen. J Appl Polym Sci. 99:2671–2678.
- Ritger P, Peppas NA. 1987. A simple equation for description of solute release II: Fickian and anomalous release from swellable devices. J Control Release. 5:37–42.
- Rokhade, AP, Patil SA, Aminabhavi TM. 2007a. Synthesis and characterization of semi-interpenetrating polymer network microspheres of acrylamide grafted dextran and chitosan for controlled release of acyclovir. Carbonhydr Polym. 67:605–613.
- Rokhade AP, Shelke NB, Patil SA, Aminabhavi TM. 2007b. Novel interpenetrating polymer network microspheres of chitosan and methylcellulose for controlled release of theophylline. Carbohydr Polym. 69:678–687.
- Sogias IA, Williams AC, Khutoryanskiy VV. 2012. Chitosan-based mucoadhesive tablets for oral delivery of ibuprofen. Int J Pharm. 436:602–610.
- Singh V, Sharma AK, Sanghi R. 2009. Poly(acrylamide) functionalized chitosan: An efficient adsorbent for azo dyes from aqueous solutions. J Hazard Mater. 166:327–335.
- Sinha VR, Singla AK, Wadhawan S, Kaushik R, Kumria R, Bansal K, Dhawan S. 2004. Chitosan microspheres as a potential carrier for drugs. Int J Pharm. 274:1–33.
- Sokker HH, El-Sawy NM, Hassan MA, El-Anadouli BE. 2011. Adsorption of crude oil from aqueous solution by hydrogel of chitosan based polyacrylamide prepared by radiation induced graft polymerization. J Hazard Mater. 190:359–365.
- Sullad AG, Manjeshwar LS, Aminabhavi TM. 2010. Controlled release of theophylline from interpenetrating blend microspheres of poly(vinyl alcohol) and methyl cellulose. J Appl Polym Sci. 116:1226–1235.
- Şanlı O, Ay N, Işıklan N. 2007. Release characteristics of diclofenac sodium from poly(vinyl alcohol)/sodium alginate and poly(vinyl alcohol)-grafted-poly(acrylamide)/sodium alginate blend beads. Eur J Pharm Biopharm. 65:204–214.
- Şanlı O, Biçer E, Işıklan N. 2008. In vitro release study of diltiazem hydrochloride from poly(vinyl pyrrolidone)/sodium alginate blend microspheres. J Appl Polym Sci. 107:1973–1980.
- Şanlı O, Karaca İ, Işıklan N. 2009. Preparation, characterization, and salicylic acid release behavior of chitosan/poly(vinyl alcohol) blend microspheres. J Appl Polym Sci. 111:2731–2740.
- Thacharodi D, Panduranga Rao K. 1993. Release of nifedipine through crosslinked chitosan membranes. Int J Pharm. 96:33–39.
- Valot P, Baba M, Nedelec J-M. Sintes-Zydowicz, N. 2009. Effects of process parameters on the properties of biocompatible ibuprofen-loaded microcapsules. Int J Pharm. 369:53–63.
- Wang Z, Wu H, Liao C, Zhou N, Cheng W, Wan Y. 2011. Sustained release of ketoprofen from fibrous chitosan-poly(ε-caprolactone) membranes. Carbohydr Polym. 84:624–630.
- Yuan Q, Venkatasubramanian R, Hein S, Misra RDK. 2008. A stimulus-responsive magnetic nanoparticle drug carrier: magnetite encapsulated by chitosan-grafted-copolymer. Acta Biomater. 4:1024–1037.