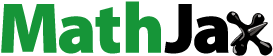
Abstract
Context: Herpes viruses cause threatening infections in humans and stand second as causative agents for most human viral diseases, after influenza and cold viruses. Objective: A novel multiparticulate delivery system for acyclovir (ACV), based on ion-exchange resin, was developed to achieve a gastro-mucoadhesive effect in order to effectively combat the herpes simplex virus. Materials and methods: A combination of ACV and cholestyramine resin was optimized and further entrapped within sodium alginate and Carbopol microbeads. The developed systems were evaluated for drug entrapment efficiency (DEE), percentage of mucoadhesion, and in vitro release characteristics in simulated gastric fluid (SGF, pH 1.2). Results: With the aid of scanning electron microscopy (SEM), differential scanning calorimetry (DSC), and Fourier-transform infrared spectroscopy (FTIR), the interaction of the resinate and polycations with alginate has been revealed, which consequently supports the formation of the membrane by the polyelectrolyte complex. The in vitro drug release studies demonstrate that formulations without the drug-resin complex (DRC) released the drug more rapidly than formulations containing DRC, which released the drug in a controlled manner, due the formation of a complex between drug and resin. Discussion and conclusion: Preliminary results from this study suggest that these DRC-entrapped microbeads may be used to incorporate other antiviral drugs and could be effective against infections caused by herpes viruses. Such formulations developed could be subjected to in vivo studies in future, in order to prove complete clearance of herpes infections.
Introduction
As one of the most threatening disease-causing viruses, herpes viruses stand second as causative agents for most human viral diseases, following influenza and cold viruses. Once infected by this virus, an individual remains infected lifelong. After initial invasion of the human body, this virus goes through a period of latency, followed by reactivation. It is a common infection in most people past their middle age, and the human body usually has antibodies to most strains of the herpes viruses, with the exception of the Human Herpes Virus (CitationCavalli et al. 2009).
Acyclovir (ACV), a selective and one of the most efficient antiviral drugs, is a synthetic purine nucleoside analog derived from guanine (CitationJin et al. 2006). ACV is effective against strains of the herpes simplex virus HSV-1 and HSV-2 and the varicella-zoster virus, and acts by interfering with DNA synthesis in order to inhibit viral replication (CitationStulzer et al. 2008, CitationSultan 2002). The oral bioavailability of ACV ranges from 10% to 30% (CitationFletcher and Bean 1985), which limits its effectiveness. Thus, novel delivery systems are required to enhance the oral bioavailability. Approximately 80% of orally administered ACV remains unabsorbed and is excreted through the feces. The plasma half-life of ACV in adults with normal renal function after oral administration is approximately 3 h (CitationVergin et al. 1995). The poor retention ability of dosage forms at the absorption sites for ACV, that is, the region from the upper gastrointestinal (GI) tract to the duodenum and jejunum, is the foremost reason for the low bioavailability of this antiviral agent (CitationDhaliwal et al. 2008, CitationTao et al. 2009). This shortcoming leads to the inconvenient need for dosing 200 mg five times a day, which can be overcome by the novel approaches in developing new formulations.
The ability of some ion exchange resins (IER), especially anionic exchange resins (AER) such as cholestyramine for electrostatic interactions with mucin and epithelial surfaces confers them with bio/mucoadhesive properties (CitationYao et al. 2008). The incorporation of such bioadhesive IER in the formulations offers attractive alternatives for the targeting of therapeutic agents like ACV to the GI tract (CitationAnand et al. 2001). By this approach, one can retain ACV within the absorption window for a longer period, to achieve enhanced localized delivery, which conventional dosage forms fail to achieve. Several studies have reported the successful utilization of IER that is, indion 454, amberlite IR 400, and cholestyramine with different therapeutic agents (CitationKulkarni et al. 2011, CitationHalder et al. 2005, CitationRobberson et al. 2006, CitationPongjanyakul et al. 2005).
On the basis of previous studies, the present attempt utilizes the bioadhesive property of IER for the development of a controlled-release gastro-retentive multiparticulate system loaded with ACV for the effective treatment of herpes infection. To accomplish this objective, cholestyramine was taken as the anion exchange resin and studied for its binding efficiency with ACV. The drug/resin ratio with maximum drug binding efficiency and better compatibility characteristics was selected. This resinated complex was finally encapsulated within rate-controlling mucoadhesive microbeads, to further sustain the drug release.
Materials and methods
Materials
Acyclovir Sodium (ACV) was received as a gift sample from M/s XL Pharma Pvt. Ltd., Bhivadi, India. Cholestyramine resin (Tulsion 412) was procured as a gift sample from Thermex Ltd., Pune, India. Sodium Alginate and Carbopol were purchased from HiMedia Laboratories Pvt. Ltd., Mumbai, India. Calcium Chloride (CaCl2) was purchased from CDH Laboratory Pvt. Ltd., Mumbai, India. All other chemicals used were of analytical reagent grade.
Purification and activation of resin
The cholestyramine resin was purified and activated according to the previously reported method (CitationIchikawa et al. 2001, CitationHalder and Sa 2006, CitationKulkarni et al. 2011). First, cholestyramine (20 g) was washed with 200 ml of deionized water and 95% ethanol (50 ml) and 50% ethanol (50 ml), to remove impurities. Then, it was activated by recycling three times alternatively with 60 ml of 2 M sodium hydroxide and 60 ml of 2 M hydrochloric acid, followed by washing with deionized water after each treatment. Finally, the treated resin was washed with deionized water until a neutral elute was obtained, and then dried under vacuum at 50°C to a constant weight.
Preparation of the drug-resin complex
A batch process was adopted for the preparation of drug-resin complexes (DRC) (CitationJeong and Park 2008, CitationKulkarni et al. 2011). Drug and cholestyramine resin complexes were prepared in four different ratios (1/1, 1/2, 1/3, and 1/4). For the preparation of each batch, a requisite amount of previously purified cholestyramine resin was added to 20 ml of aqueous ACV solution. This mixture was then stirred for 4 h on a mechanical stirrer (Macro Scientific Works, India) followed by filtration through Whatman filter paper (#41). The residue or drug-loaded resin complex was washed with distilled water to remove the traces of unbound drug, if any, followed by drying in a hot air oven at 40°C for 12 h. Simultaneously, the filtrate was analyzed by a UV spectrophotometer (UV-1800, Shimadzu, Japan) to find the total amount of unbound drug (CitationRaju et al. 2013). The extent of drug-resin complexation was reckoned as the difference between the amount of total drug added initially and the amount remaining in filtrate. The ion exchange resin-drug combination which showed best results in terms of drug binding efficiency with comparatively less resin consumption was selected as the optimum DRC for the formulation of microbeads (data not shown).
Formulation of alginate-Carbopol microbeads
The DRC-loaded alginate-Carbopol beads were prepared by the ionotropic gelation method using CaCl2 as a counter ion (CitationNayak et al. 2011, CitationMohammad et al. 2011). Sodium alginate and Carbopol were dispersed separately in deionized water. DRC was mixed with the Carbopol dispersion followed by homogenization for 10 min at 1000 rpm using a homogenizer (ULTRA-TURRAX®, IKA® Pvt. Ltd., India). The final alginate-Carbopol dispersion containing the polymer mixture in water was kept at 2% w/v, and the mixture was ultrasonicated for 5 min in order to remove air bubbles. The ratio of drug to polymer was maintained at 1/1 (% w/w) in all the formulations. DRC-loaded alginate-carbopol beads were prepared by varying the ratio of sodium alginate to Carbopol (). The resulting dispersion was then added via a needle (#22) to stirred CaCl2 solution (5% w/v). The droplets added were retained in the CaCl2 solution for 20 min to complete the curing reaction and to produce spherical, rigid beads. The beads were collected by decantation, and washed repeatedly with deionized water. Collected beads were dried in a hot air oven at 35°C for 24 h, and stored in a desiccator for further evaluations. The microbeads containing only the drug, without ion exchange resin (NRMB), were also prepared by the same procedure with alginate and Carbopol in a ratio of 3/7.
Table I. Batch specification of the microbeads.
Characterization of drc and microbeads
Drug-loading in cholestyramine resin
The amount of drug bound to the resin was calculated as the difference between the initial and remaining amounts of drug in the filtrate collected at the time of drug-resin complexation. The loading efficiency was calculated by the following formula:
Micromeritic studies
The particle size of drug, resin, DRC, and microbeads was determined by an optical microscope equipped with a previously calibrated ocular micrometer. The mean particle size was calculated by measuring 300 particles with the help of the ocular micrometer. The experiment was performed in triplicate.
For the surface morphology studies of optimized DRC and formulation, scanning electron microscopy (SEM, JSM 5600 JEOL, Japan) was performed. A sample (DRC and microbeads) was mounted on a stub covered with clean glass. A Polaron E5100 sputter coater was used to coat the samples with gold, and the samples were examined under SEM at an accelerating voltage of 20 kV.
The optimized microbeads were characterized for their micromeritic properties such as apparent density, true density, tapped density, porosity, and compressibility index.
The density measurements were carried out using the benzene displacement method. Pre-weighed beads were placed in a graduated cylinder filled with a known volume of benzene (V1). The total volume following microbead immersion was recorded (V2). The microbeads with the entrapped solvent in the pores were removed, and the remaining volume of benzene in the graduated cylinder was measured (V3). Therefore, the true volume (Vt) of microbeads is given by
For tapped density, a weighed amount of microbeads was placed in a 10 ml measuring cylinder and the exact volume of beads was measured. The cylinder was tapped on a hard surface at a rate of 100 taps per minute until no further change in volume was noted. This final volume was also measured. The values of tapped density and % compressibility index were calculated using the equations:
Porosity (ε) was calculated using the equation:
All the experiments were performed in triplicate.
Infrared spectroscopy
IR spectroscopy of drug, resin, alginate, Carbopol, DRC, and formulation was performed on Fourier transform-infrared spectrophotometer (FTIR-8400 S, Shimadzu, Japan) to confirm the presence of drug in the resinate. The samples and KBr were mixed properly in a ratio of 95/5 and placed on the sample holder as a pellet. The spectra were scanned over 3000 to 400 cm−1.
Differential scanning calorimetry
A differential scanning calorimeter (DSC-60, Shimadzu, Japan) equipped with an intra-cooler and a refrigerated cooling system was used to analyze the thermal behavior. Drug, resin, alginate, Carbopol, DRC, and formulation were placed in hermetically sealed flat aluminum crucibles and scanned over 50°C to 350°C (heating/cooling rate = 10°C/min). Nitrogen was purged at 40 and 100 ml/min through a cooling unit.
Percentage yield and drug entrapment efficiency
The prepared microbeads were collected by filtration, dried in desiccators, and weighed. Experiments were performed in triplicate and percentage yield was calculated using the following formula:
Microbeads (100 mg) were crushed in a glass mortar with pestle and incubated with 20 ml of phosphate buffer (pH 7.4) for complete swelling at 37°C (CitationKulkarni et al. 2011). The dispersion was heated gently for 3 h to extract the drug completely, and centrifuged at 1500 rpm for 15 min to remove the polymeric debris. The clear supernatant solution was analyzed for the drug content using a UV-spectrophotometer at 255 nm. The drug entrapment efficiency (DEE) was calculated using the following equation:
Dynamic swelling study
The dynamic swelling behavior of the microbeads was studied by mass measurement (CitationKulkarni et al. 2011). The microbeads were incubated with 25 ml of simulated gastric buffer solution (pH 1.2) in a Petri dish at 37°C. The microbeads were taken out at different time intervals without pressing hard to remove the excess surface liquid. The swollen microbeads were weighed on an electronic balance (BL310S, Setra, Japan) having an accuracy of 0.001 mg. The studies were performed in triplicate and average values were taken for data analysis.
Percent mucoadhesion
Albino rats (400–500 g) fasted overnight were sacrificed to and their stomach removed (CitationJain et al. 2014, CitationJain and Jangdey 2009), which was divided into pieces of 2 × 1 cm and rinsed with 2 ml of physiological saline. Previously counted microbeads (100 mg) were scattered uniformly over gastric mucosa and placed in a chamber for 20 min, and then maintained at room temperature with relative humidity of 93%. They were then transferred to a polyethylene support and fixed at an angle of 45°, followed by rinsing with physiological saline solution (pH 1.3) for 5 min at a rate of 20 ml/min. The microbeads remaining at the surface of gastric mucosa were counted and the percentage of the remaining microbeads was calculated. The experiment was performed in triplicate, and the percentage of binding was calculated by the following formula:
Where Wo is initial number of microbeads; and Wr is number of residual microbeads.
In vitro drug release study
The in vitro drug release study was carried out in triplicate using a USP XXIII basket type dissolution apparatus (Jyoti Scientific Laboratories, Gwalior, India). A weighed amount of mucoadhesive microbeads (100 mg) was filled into a capsule (#4) and placed in the basket. Simulated gastric fluid (SGF, pH 1.2) (900 ml) was used as the dissolution medium, and maintained at 37 ± 0.5°C with stirring at 100 rpm. Sample (5 ml) was withdrawn at each 30 min interval, passed through a Whatman filter paper (#41) and analyzed spectrophotometrically at 255 nm to determine the concentration of drug present in the dissolution medium. The initial volume of dissolution fluid was maintained by adding 5 ml of fresh dissolution fluid after each withdrawal. The same procedure was used for NRMB and the marketed tablet formulation of ACV (Zovirex, 200 mg, GlaxoSmithKline, India).
Statistics
Differences in in vitro drug release of ACV from cholestyramine resin-based microbeads, microbeads without resin, DRC, and marketed formulation (Zovirex) were statistically analyzed by one way analysis of variance (ANOVA) with post test (Dunnett's multiple comparison test). Statistically significant differences between rates of in vitro drug release of formulations were defined as P < 0.05. Calculations were performed with the GraphPad-In Stat software program.
Model fitting of the release study
Reichenberg's and Bhaskar's particle diffusion-controlled models are often used for determination of the diffusion mechanism (CitationReichenberg 1953, CitationBhaskar et al. 1986). In the first model, the expressions presented by Reichenberg are shown below:
For F values higher than 0.85
For F values less than 0.85
Where F stands for fraction of dissolution value, Qt represents the amount of drug release at time ‘t’, Q∞ is total amount of drug in resin, and B shows the exchange rate constant (min− 1). The value of Bt may be plotted against the experimental value of t. At a value of F = 0.85, Equations (2) and (3) yielded values of Bt below 0.005, corresponding to a variation in F of less than 0.001 (CitationReichenberg 1953). If the plot Bt corresponding to the F value against time yields a straight line, it can be assumed that drug diffusion within the resin matrix is the rate-limiting step (CitationAtyabi et al. 1996, CitationIchikawa et al. 2001, CitationJeong et al. 2007).
Another mathematical approach, the Bhaskar expression (CitationBhaskar et al. 1986) is also used to evaluate particle diffusion control, as shown by the following equations:
Where F stands for fraction of dissolution value, dp is the mean diameter of resin (mm), D represents diffusion coefficient or diffusivity (mm2/min), and t is time. Particle diffusion control can be extrapolated by calculating from a linear relationship between − ln(1 − F) and t0.65.
CitationRitger and Peppas (1987) give the equation for a swellable matrix
Where Mt is the amount of drug released at time t, M∞ represents the total amount of drug loaded, and n indicates the type of drug release. The values of n between 0.45 and 0.85 suggest both diffusion-controlled and swelling-controlled transport mechanisms (anomalous/non-Fickian transport); values above 0.85 indicate case II transport, which relates to polymer relaxation during polymer swelling.
Stability studies
The stability studies were performed to observe any degradation behavior of the optimized formulation. Optimized microbeads were kept in amber colored glass bottles at 25 ± 2°C and 40 ± 2°C, with relative humidity of 60 ± 5% and 75 ± 5% respectively, for 30 days. They were then characterized for their morphology, particle size, residual drug content, in vitro percent of mucoadhesive ability, and in vitro drug release.
Results and discussion
Purification and activation of cholestyramine resin
Impurity associated with industrial scale manufacture or absorbed during storage or handling of resin might produce unwanted effects. Cholestyramine was treated with ethanol (95%) to solubilize the impurities, which can then be easily separated by filtration. Further treatment with ethanol (50%) caused total separation of impurities.
The activation of resin to expose exchangeable groups responsible for rapid drug exchange was attributed to higher efficiency of the drug-resin complexation. The highest drug-resin complexation can be achieved when both acid-alkali treatments were used for activation. The acidic group of resin was activated by treatment with hydrochloric acid and the basic group was activated by treatment with sodium hydroxide.
Preparation of DRC
Loading of ACV onto cholestyramine resin involved replacement of the Cl− of cholestyramine resin with the Na+ of ACV due to the strong anionic behavior of cholestyramine (). Initially, the binding sites in the cholestyramine resin are well-occupied by counter-ions (Cl−). Once exposed to a drug solution, the counter-ions on the surface are displaced by drug ions and carried away by the well-stirred bulk liquid (CitationPisal et al. 2004).
ACV-cholestyramine complexation was increased with an increase in concentration of drug solution. The drug/resin ratio of , was selected due to involvement of less resin and higher % complexation compared to 1/3 and 1/4 drug/resin ratios, and hence, was further used for microbead preparation.
Preparation of DRC-loaded microbeads
Different combinations of sodium alginate and Carbopol 934P were used for the coating of DRC. An aqueous insoluble calcium alginate gel was formed by cation exchange between Na+ and Ca2+. The gelation and crosslinking are due to the stacking of the glucuronic acid-G blocks of alginate chains, with the formation of egg-box junction (CitationMatricardi et al. 2008).
Characterization of DRC and microbeads
Micromeritic studies
The optimized DRC was found to be non-uniform in size with irregular shape and rough surface when examined under a photomicroscope (). The mechanical strength of the resin was probably not strong enough, resulting in resin debris as observed in the SEM image. The spherical shape of beads was also revealed by the SEM images (). Small cracks were observed on the rough surface due to shrinkage, probably caused by the drying process. These findings are supported by the earlier SEM studies on beads (CitationTekade and Gattani 2010). The cross-sectional morphology of microbeads confirms the encapsulation of the resinate complex within the microbeads ().
Figure 2. SEM photomicrographs of (A) drug-resin complex, (B) population of microbeads, (C) Single microbead, and (D) cross-sectional view of a microbead.

The average particle size of optimized DRC (that is, DRC2) was found to be 16.54 ± 4.24 μm, which was slightly higher than the usual particle size of pure resin (13.34 ± 2.5 μm). Particle size analysis of microbeads shows the size ≤ 200 μm for all formulation batches (), a comparable size for oral delivery. An increase in Carbopol concentration increases the size of microbeads, which could be attributed to bigger droplet formation due to higher viscosity of the polymer solution.
Table II. Micromeritic studies of the prepared formulations.
The compressibility index values were less than 20 for all batches, suggesting good flow characteristics of beads. The tapped density was found to be 1.20 ± 0.051 g cm− 3 to 1.63 ± 0.031 g cm− 3, while the apparent density was found to be between 1.37 ± 0.041 g cm− 3 and 1.64 ± 0.015 g cm− 3. The values for tapped density, apparent density, and true density of the formulation were found to be more than the density of gastric fluids (1.04 g/cm3), which does not support floating ability. Porosity was found to be from 13.54 ± 4.7% to 26.82 ± 3.04%, which indicates the porous nature of microbeads ().
Infrared spectroscopy
The IR spectrum of ACV showed prominent peaks at 1413 cm− 1 due to the hydroxyl group (O–H) stretching, at 1352 cm− 1 due to aromatic amine (N–H) stretching, at 1215.15 cm− 1 due to aromatic ring (C–N) stretching, and at 3475.73 cm− 1 due to aromatic ring (N–H) stretching (). The IR spectrum of cholestyramine shows characteristic peaks at 1030 cm− 1 due to the quaternary ammonium group (). The IR spectrum of sodium alginate shows characteristic absorption bands at 1610 cm− 1 due to carbonyl group (C–O) stretching and at 1412 cm− 1 due to ketone group (C–O–C) stretching (). The IR spectrum of Carbopol showed the characteristic absorption bands at 2912 cm− 1 due to aliphatic (C–H) stretching, at 1443 cm− 1 due to hydroxyl (O–H) stretching, and at 1653 cm− 1 due to aliphatic (C–C) stretching (). The IR spectrum of DRC2 has characteristic peaks of ACV such as 1216 cm− 1 (due to aromatic ring C–N stretching), 3475 cm− 1 (due to aromatic ring N–H stretching), and 1112 cm− 1 (due to aromatic amine N–H stretching), confirming the presence of drug in the resinate (). These results indicate that drug binding to resin is not attributed to the –NH2 group of ACV, as there is no change in the peaks of N–H stretching as well as of C–N stretching. The peak of ACV due to aliphatic hydroxyl (1425 cm− 1) vanished, which indicates the potential binding of resin at the hydroxyl group of drug. The IR spectrum of DRC2 shown by the characteristic peak of cholestyramine resin at 1030 cm− 1 due to C–N stretching of quaternary amine was shifted to 1105.21 cm− 1. This analysis confirms the complexation of drug and resin. The IR spectrum of the formulation showed the characteristic peak of DRC at 1105.21 cm− 1, confirming the complex entrapped in formulation (). The characteristic peak of alginate was also found at 1413 cm− 1 due to ketone group (C–O–C) stretching.
Differential scanning calorimeter
Thermograms of ACV, cholestyramine, sodium alginate, Carbopol, DRC2, and microbeads were recorded by DSC, to characterize the solid state of drug in beads and to check any existing interaction between the components and drug. Pure ACV showed a sharp exothermic peak at 252.92°C in the thermogram. This transition is attributed to compound melting (CitationCavalli et al. 2009) (). Pure cholestyramine showed an endothermic peak at 102°C, which may be due to evaporation of water. The peak at 265°C is attributed to compound melting (). DSC scans of sodium alginate showed a wide endothermic peak at around 85°C () that has been attributed to the evaporation of water, and appearance of an exothermic behavior was detected starting at around 200°C, with its maximum at 255°C, coinciding with the exothermic behavior of the sodium alginate, as reported in several publications (CitationCrcarevska et al. 2008, CitationBasu and Rajendran 2008) as indicating the decomposition of the polymer. Pure Carbopol showed an exothermic peak at 248°C, attributed to compound melting (). The thermal behavior of DRC2 shows an endothermic peak at 260°C, corresponding to the peak of cholestyramine. The exothermic peak at 252°C corresponding to the ACV fusion is absent in the DSC thermograms of both DRC2 and the formulation, confirming the drug complexation with resin. The endothermic peak at 225°C and the exothermic peak at 294°C may be due to complex formation (). Thermal behavior of the formulation (microbeads) shows a peak at 258°C, corresponding to the peak of alginate. The peak at 295°C corresponds to the peak of DRC2 ().
Percentage yield and drug entrapment efficiency
The percentage yield of the prepared formulations was found to be in the range of 89.23 ± 0.96% to 95.86 ± 2.62%, showing neither a regular trend nor a significant change with increase in polymer content (). The DEE of all formulations ranged from 72.48 ± 0.56% to 91.32 ± 1.13% and was prominently affected by polymer concentration, with a proportional increase toward higher concentration of polymer. This enhancement may possibly be due to the highly dense polymeric network of alginate and Carbopol (CitationAhmed et al. 2010).
Table III. Yield, drug entrapment, and mucoadhesion of formulations.
Dynamic swelling study and percent mucoadhesion
Polymer crosslinking markedly influences the swelling behavior of microbeads. Diminished swelling was observed with increase in sodium alginate concentration, owing to the formation of a stiffer network. At low crosslink density, the polymer network is loose with more hydrodynamic free volume, and can absorb more solvent, resulting in higher swelling. The swelling property of the formulation followed the order: NRMB ≥ MB-9 > MB-8 > MB-7 > MB-6 > MB-5 > MB-4 > MB-3 > MB-2 > MB-1. These results are in agreement with the results obtained by CitationKulkarni et al. (2011) and CitationHalder and Sa (2006). Mucoadhesion studies were carried out to ensure adhesion of the formulation to the mucosa for a prolonged period of time. Higher concentration of the mucoadhesive polymer, that is, Carbopol, results in better mucoadhesive property, as shown by the formulation MB-9, to an extent of 89% in comparison to other formulations ().
In vitro drug release study
The rates of drug release from the marketed formulation (Zovirex), DRC, NRMB and MB-9 were found to be 99.59 ± 0.19% within 2.5 h, 99.81 ± 0.16% within 3.5 h, 93.21 ± 0.32% within 8 h, and 59.39 ± 1.27% within 8 h, respectively (). The release of ACV from prepared formulations followed the order: DRC > NRMB > MB-1 > MB-2 > MB-3 > MB-4 > MB-5 > MB-6 > MB-7 > MB-8 > MB-9.
Figure 5. Comparison of in vitro drug release profile of prepared formulations and marketed product. NRMB, Non-Resinate Microbeads; Zovirex (marketed product of Acyclovir); DRC2, Drug-resin complex; MB, Microbeads. The values are mean ± S.D. (n = 3).

The effect of complexation of drug and resin was clearly demonstrated as the release of drug is rapid from DRC when compared to that from NRMB. Encapsulation of DRC into microbeads further sustained the release of ACV due to the hindrance created by the polymeric network. The overall comparison of release profiles revealed that microbeads containing the DRC have better control over the drug release in comparison to DRC and NRMB. The release pattern also provides an idea about the effect of polymer content, that is, as the polymer concentration increases, drug release decreases.
Upon comparing the data of Zovirex (marketed product) with DRC2 and microbeads containing DRC2 by the one-way ANOVA (Dunnett's multiple comparison) test, the in vitro rates of release in SGF (pH 1.2) from formulations were found to be significant (P < 0.01) except in the case of DRC2 (P > 0.05) ().
Table IV. One way ANOVA (Dunnett's multiple comparison) test for in vitro drug release of acyclovir in SGF (pH 1.2).
Further, kinetic parameters for release data were calculated by Reichenberg's and Bhaskar's diffusion-controlled models and the RitgerPeppas model. The release of drug from MB-1, MB-3, MB-4, MB-5, MB-6, MB-7 and DRC2 were fitted with Reichenberg's model, as indicated by a correlation coefficient > 0.95, so it can be believed that drug diffusion within the matrix is a rate-limiting step. The release of the drug from MB-1, MB-2, MB-4, MB-9, and DRC2 were fitted with Bhaskar's model, as indicated by a correlation coefficient > 0.95, so it can be assumed that drug release was controlled by particle diffusion control. The rates of release of the drug from MB-1, MB-4 and DRC2 were fitted with both Reichenberg's model and Bhaskar's model, as indicated by a correlation coefficient > 0.95. Therefore, the kinetics of ACV release from the ion-exchange resin was controlled by particle diffusion control or by the release of drug through the porous particles.
All formulations (MB-1 to MB-9) and DRC2 had maximum r2 value for the Ritger–Peppas model, and NRMB follow the Reichenberg model. The values of n (Ritger–Peppas) for all formulations and DRC2 were calculated between 0.45 and 0.85 (), which is an indication of both diffusion-controlled and swelling-controlled transport mechanism (anomalous/non-Fickian transport). The drug release model (Ritger–Peppas) for DRC2 is in agreement with the results of earlier studies (CitationJunyaprasert and Manwiwattanakul 2008, CitationJeong and Park 2008, CitationHalder and Sa 2006).
Table V. Release kinetics of acyclovir sodium.
The comparison of release profiles clearly demonstrates the superiority of MB-9 over other formulations, including the marketed formulation, in terms of drug sustainability. These findings regarding the control over drug release after encapsulation are also supported by the experimental results of CitationKulkarni et al. (2011), CitationHalder and Sa (2006), and CitationIchikawa et al. (2001).
Stability studies
There was no significant morphological change seen in microbeads stored at 25 ± 2°C, but the surface of microbeads appeared slightly rough in the case of microbeads stored at 40°C. The particle size (221.65 ± 5.42) was slightly decreased (220.29 ± 4.68) at 40 ± 2°C, which might be attributed to loss of moisture at higher temperature, but at 25 ± 2°C, the change in particle size was negligible (221.98 ± 5.27). There is no change in residual drug content after storage for 30 days at 25 ± 2°C and 40 ± 2°C, which shows that drugs are stable at 25 ± 2°C and 40 ± 2°C. There was no significant change (P > 0.05) in drug release from the optimized formulation when stored at 25 ± 2°C and 40 ± 2°C. No change was observed in the mucoadhesive ability of microbeads after storage for a specified period.
Conclusion and future perspectives
It is concluded that the method followed for the preparation of microbeads was simple and reproducible, with good percentage yield. The prepared formulation showed better prolonged release when compared to the marketed product of ACV. A formulation such as the one developed could be further subjected to in vivo studies in order to design a viable formulation for complete clearance of Herpes viral infections.
Acknowledgment
One of the authors, Ajay Kumar, gratefully acknowledges the University Grants Commission, New Delhi, India for awarding him the Junior Research Fellowship during his PG program.
Declaration of interest
The authors declare no conflict of interest. The authors alone are responsible for the content and writing of the paper.
References
- Ahmed MG, Satish KBP, Kiran KGB. 2010. Formulation and evaluation of gastric mucoadhesive drug delivery systems of captopril. J Curr Pharm Res. 2:26–32.
- Anand V, Kandarapu R, Garg S. 2001. Ion-exchange resins: carrying drug delivery forward. Drug Discov Today. 6:905–914.
- Atyabi F, Sharma HL, Mohammad HAH, Fell JT. 1996. In vivo evaluation of a novel gastric retentive formulation based on ion-exchange resins. J Control Release. 42:105–113.
- Basu SK, Rajendran A. 2008. Studies in the development of nateglinide loaded calcium alginate and chitosan coated calcium alginate beads. Chem Pharm Bull (Tokyo). 56:1077–1084.
- Bhaskar R, Murthy RSR, Miglani BD, Viswanathan K. 1986. Novel method to evaluate diffusion controlled release of drug from resinates. Int J Pharm. 28:59–66.
- Cavalli R, Donalisio M, Civra A, Ferruti P, Ranucci E, Trotta F, Lembo D. 2009. Enhanced antiviral activity of Acyclovir loaded into β-cyclodextrin-poly(4-acryloylmorpholine) conjugate nanoparticles. J Control Release. 137:116–122.
- Crcarevska MS, Dodov MG, Goracinova K. 2008. Chitosan coated Ca–alginate microparticles loaded with budesonide for delivery to the inflamed colonic mucosa. Eur J Pharm Biopharm. 68:565–578.
- Dhaliwal S, Jain S, Singh HP, Tiwary AK. 2008. Mucoadhesive microspheres for gastro-retentive delivery of acyclovir: in vitro and in vivo evaluation. The AAPS Journal. 10: 322–330.
- Fletcher C, Bean B. 1985. Evaluation of oral acyclovir therapy. Drug Intell Clin Pharm. 19:518–524.
- Halder A, Maiti S, Sa B. 2005. Entrapment efficiency and release characteristics of polyethyleneimine-treated or–untreated calcium alginate beads loaded with propranolol-resin complex. Int J Pharm. 302:84–94.
- Halder A, Sa B. 2006. Sustained release of propranolol hydrochloride based on ion-exchange resin entrapped within polystyrene microcapsules. J Microencap. 23:899–911.
- Ichikawa H, Fujioka K, Adeyeye MC, Fukumori Y. 2001. Use of ion-exchange resins to prepare 100 mm-sized microcapsules with prolonged drug-release by the Wurster process. Int J Pharm. 216: 67–76.
- Jain SK, Jangdey MS. 2009. Lectin conjugated gastroretentive multiparticulate delivery system of clarithromycin for the effective treatment of H. Pylori. Mol Pharm. 6:295–304.
- Jain SK, Gupta M, Sahoo AS, Pandey AN, Jain AK. 2014. Lectin conjugated gastro-retentive microspheres of amoxicillin for effective treatment of H. pylori. Curr Sci. 106:267–276.
- Jeong SH, Park K. 2008. Drug loading and release properties of ion-exchange resin complexes as a drug delivery matrix. Int J Pharm. 361:26–32.
- Jeong SH, Berhane NH, Haghighi K, Park K. 2007. Drug release properties of polymer coated ion-exchange resin complexes: Experimental and theoretical evaluation. J Pharm Sci. 96:618–632.
- Jin Y, Tong L, Ai P, Li M, Hou X. 2006. Self-assembled drug delivery systems, Properties and in vitro/in vivo behavior of acyclovir self-assembled nanoparticles (SAN). Int J Pharm. 309: 199–207.
- Junyaprasert VB, Manwiwattanakul G. 2008. Release profile comparison and stability of diltiazem-resin microcapsules in sustained release suspensions. Int J Pharm. 352:81–91.
- Kulkarni RV, Mangond BS, Mutalik S, Biswanath S. 2011. Interpenetrating polymer network microcapsules of gellan gum and egg albumin entrapped with diltiazem–resin complex for controlled release application. Carb Polymers. 83:1001–1007.
- Matricardi P, Meo CD, Coviello T, Alhaique F. 2008. Recent advances and perspectives on coated alginate microspheres for modified drug delivery. Expert Opin Drug Deliv. 5:417–425.
- Mohammad Shadab, Ahuja A, Khar RK, Baboota S, Chuttani K, Mishra AK, Ali J. 2011. Gastroretentive drug delivery system of acyclovir-loaded alginate mucoadhesive microspheres: Formulation and evaluation. Drug Deliv. 18:255–264.
- Nayak A, Jain SK, Pandey RS. 2011. Controlling release of metformin HCl through incorporation into stomach specific floating alginate beads. Mol Pharm. 8:2273–2281.
- Pisal S, Zainnuddin R, Nalawade P, Mahadik M, Kadam S. 2004. Drug release properties of polyethylene-glycol-treated ciprofloxacin-indion 234 complexes. AAPS PharmSciTech. 5: 101–106.
- Pongjanyakul T, Prakongpan S, Rungsardthong U, Chancham P, Priprem A. 2005. Characteristics and in vitro release of dextromethorphan resinates. Powder Technol. 152:100–106.
- Raju PN, Dey B, Fathi HA, Prakash K, Babu RC. 2013. UV-spectrophotometric estimation of acyclovir in bulk and pharmaceutical dosage forms. J Pharm Sci Innovation. 2:40–43.
- Reichenberg D. 1953. Properties of ion-exchange resins in relation to their structure: III. Kinetics of exchange. J Am Chem Soc. 75: 589–597.
- Ritger PL, Peppas NA. 1987. A simple equation for description of solute release. II. Fickian and anomalous release from swellable devices. J Control Release. 5:37–42.
- Robberson KA, Waghe AB, Sabatini DA, Butler EC. 2006. Adsorption of the quinolone antibiotic nalidixic acid onto anion-exchange and neutral polymers. Chemosphere. 63:934–941.
- Stulzer HK, Lacerda L, Tagliari MP, Silva MAS, Favere VT, Laranjeira MCM. 2008. Synthesis and characterization of cross-linked malonyl-chitosan microspheres for controlled release of acyclovir. Carb Polymers. 73:490–497.
- Sultan M. 2002. Spectrophotometric determination of acyclovir in some pharmaceutical formulations. Farmaco. 57:865–870.
- Tao Y, Lu Y, Sun Y, Gu B, Lu W, Pan J. 2009. Development of mucoadhesive microspheres of acyclovir with enhanced bioavailability. Int J Pharm. 378:30–36.
- Tekade AR, Gattani SG. 2010. Dual cross-linked pulsatile beads for chronotherapy of asthma: Development and evaluation. Drug Deliv. 17:581–586.
- Vergin H, Kikuta C, Mascher H, Metz R. 1995. Pharmacokinetics and bioavailability of different formulations of acyclovir. Arzneimittelforschung. 45:508–515.
- Yao H, Xu L, Han F, Che X, Dong Y, Guan J, Li S. 2008. A novel riboflavin gastro-mucoadhesive delivery system based on ion-exchange fiber. Int J Pharm. 364:21–26.