Abstract
This study was designed to investigate whether resuscitation with polymerized human placenta hemoglobin (PolyPHb) extended survival and the efficacy of hydroxyethyl starch (HES) in a rat model of hemorrhagic shock. After withdrawal of 39 ml of blood/kg, rats were randomly resuscitated with PolyPHb/HES or HES alone. Systemic, hemodynamic, and blood gas parameters, tissue oxygenation, and plasma erythropoietin (EPO) were evaluated. The results showed that resuscitation with PolyPHb/HES doubled the survival rate and maintained relatively high blood pressure and tissue oxygenation when compared with the results obtained using HES 130 alone. It is concluded that infusion of PolyPHb improves the resuscitative efficacy of HES in the present experimental conditions.
Introduction
Hemorrhagic shock associated with circulatory collapse and cellular ischemia is the most frequent cause of death during post-trauma intensive care. The primary goals in the treatment of hemorrhagic shock are the restoration of intravascular volume and tissue oxygen delivery. The ideal fluids, if used at the very beginning of resuscitation, would accomplish both of these goals (CitationWettstein et al. 2006, CitationElmer et al. 2012). Whole blood is considered to be the primary agent in the treatment of traumatic hemorrhagic shock (CitationMurdock et al. 2014), but early infusion of whole blood is usually unavailable in prehospital settings. Traditional artificial colloids such as hydroxyethyl starch (HES) or dextran have none of the oxygen-carrying components of blood, though they have been well known for their intravascular volume expansion properties in clinical practice.
In theory, hemoglobin-based oxygen carriers (HBOCs) were initially designed for red blood cell replacement (CitationSquires 2002). HBOCs possess inherent properties compared to stored blood products, such as being devoid of blood type, having a long shelf-life, and high oxygen affinity, which are tremendously useful for hemorrhagic shock patients in emergency (CitationChang 2005). Polymerized human placenta hemoglobin (PolyPHb) is one of the HBOCs mainly studied by Chengmin Yang and coworkers in China (CitationLi et al. 2006, CitationZhou et al. 2013, Citation2014). It has been reported that PolyPHb could protect rat heart (CitationLi et al. 2009) or liver (CitationYou et al. 2014) from ischemic/reperfusion injury and attenuate hemorrhagic shock-induced lung injury (CitationLi et al. 2013), as it has excellent oxygen-carrying capacity. However, there is a paucity of data documenting the resuscitative efficacy of PolyPHb for the treatment of hemorrhagic shock.
In the present study, we investigated whether early resuscitation from acute hemorrhagic shock using PolyPHb extends survival in anesthetized rats. A PolyPHb solution was used with a HES solution used clinically (HES 130/0.4 and sodium chloride injection, 6%, Fresenius Kabi, Germany) in a ratio, and the resuscitative efficacy of PolyPHb/HES was compared with that of HES alone, with the same experimental protocol. Systemic, hemodynamic, and blood gas parameters, tissue oxygenation, plasma erythropoietin (EPO), and survival time were evaluated.
Materials and methods
Resuscitation fluids
The PolyPHb solution used in this study was prepared according to a previously reported procedure (CitationLi et al. 2006, CitationZhou et al. 2014). Briefly, hemoglobin purified from human placental blood (Sichuan Neo-Life Stem Cell Biotech Inc. Chengdu, China) was polymerized by glutaraldehyde. After ultrafiltration and molecular sieve chromatography, the final product had an average molecular weight of 220 (64–600) kDa and a hemoglobin concentration of 6 g/dl (pH 8.1). The physical properties of PolyPHb and HES solutions are summarized in .
Table I. Properties of PolyPHb and HES solutions.
Animal preparation
Studies were performed on male Sprague–Dawley rats, ranging in weight from 230–280 g. Animal handling and care were in accordance with The Guide for the Care and Use of Laboratory Animals (US National Institutes of Health, 1996). The details of the experiment were approved by the Animal Care and Use Committee of Chongqing University and the ethics committee of the Institute of Blood Transfusion, Chinese Academy of Medical Sciences.
Sprague–Dawley rats were anesthetized with 50 mg/kg of intraperitoneal injection of sodium pentobarbital. After catheter implantation in the femoral artery and vein, heparin sodium (500 IU) was injected for anticoagulation. Body temperature was maintained at 36–37°C during the experiment, with a heating blanket. The arterial catheter was used for blood withdrawal and measurements of mean arterial pressure (MAP). Solutions of PolyPHb and HES 130 were infused through the venous catheter. Anesthesia was maintained by injection of 15 mg/kg of sodium pentobarbital into the tail vein if necessary.
Experimental protocol
A rat model of hemorrhagic shock was prepared as described elsewhere (CitationZhao et al. 2009), with modifications. Total blood volume (BV) was estimated as 6.5% of body weight, and this value was used as the reference for all volume manipulations. Hemorrhagic shock was induced by withdrawal of 39 ml of blood/kg (60% of BV) during a 60 min period, consisting of two phases: rapid hemorrhage phase (lasting 10 min) and slow hemorrhage phase (). In brief, immediately after 20 min of stabilization and baseline measurements, a volume-controlled acute hemorrhage of 19.5 ml/kg (approximately 30% of BV) was carried out within 10 min through the femoral arterial catheter. Then the rats were subjected to a slow hemorrhage of 19.5 ml/kg (approximately 30% BV) by continued intermittent blood withdrawal for 40 min. Fluid resuscitation started after 10 min of the end of 60% blood withdrawal.
Figure 1. Volume-controlled hemorrhagic shock model in Sprague–Dawley rats. Hemorrhage (60% of BV) was completed by two steps: rapid hemorrhage (30% of BV) from 0 to 10 min and slow hemorrhage (30% of BV) from 10 to 50 min; resuscitation with PolyPHb/HES (in a 1:2 ratio) and HES 130 alone solutions from 60 to 75 min; and the observation until 72 h.
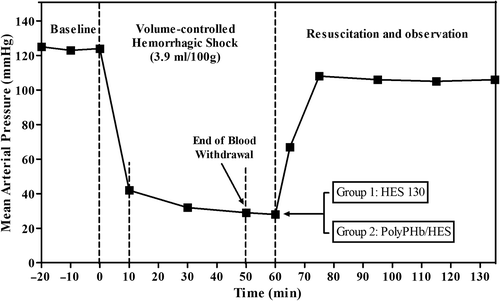
The rats were randomized into one of the following two groups: (1) PolyPHb/HES group, resuscitation with PolyPHb and HES solutions at a ratio of (n = 10); (2) HES 130 group, resuscitation with 6% HES 130/0.4 alone (n = 10). The volume of resuscitative fluid was identical to the BV removed (60% of BV). Rats were observed for 1 h after 15 min of fluid resuscitation. After that, the catheters from the femoral artery and vein were removed, the groin incision was closed, and rats were moved to a regular cage with free access to water and food, for determination of 72-h survival. After the experiment, all the surviving rats were sacrificed by euthanasia.
Systemic hemodynamic and blood gas parameter
MAP and heart rate (HR) were continuously recorded via the arterial catheter (AD Instruments Pty, Bella Vista, AUS), excluding periods where the arterial catheter was used for blood sampling and hemorrhage. The blood samples (0.3 ml) were collected from the arterial catheter and immediately used for blood gas analysis to measure the oxygen pressure (PaO2) and carbon dioxide pressure (PaCO2), base excess (BE), and pH (GEM Premier 3000, Instrumentation Laboratory, Bedford, MA), hematocrit (Hct) (Auto Hematology Analyzer 5800, Mindray, Shenzhen, CHN). Measurements were performed at the following four time-points: (1) baseline (BL) immediately before hemorrhage, (2) immediately after 60% hemorrhagic shock (SH), (3) immediately after resuscitation (RE 0), and (4) 60 min after resuscitation (RE 60).
Tissue oxygenation measurement
Subcutaneous tissue oxygen partial pressure (PtO2) was measured by a real-time tissue oxygen monitor (Oxford Optronix, Oxford, UK), using a fiber optic probe (250– 450 microns in diameter) inserted into the sub-papillary plexuses in the abdomen. These PtO2 measurements only detect dissolved molecular O2 and do not respond to hemoglobin-bound oxygen.
Plasma EPO detection with ELISA
Plasma samples (200 μl) of rats in this study were isolated by centrifugation and collected for determination of EPO immediately after resuscitation (0 h), and at 10, 16, 24, 48, and 72 h after resuscitation. Plasma EPO levels were measured by ELISA with a commercially available EPO kit (Jiancheng Biological Institute, Nanjing, CHN). The operation details were in accordance with the kit manufacturer's protocol.
Statistical analysis
All data were represented in terms of mean ± standard deviation (SD). Analysis of survival was done using the Gehan-Breslow-Wilcoxon test. Differences in mean values between groups were compared by the independent sample t-test. All statistical analyses were performed with Prism 5 for Windows (GraphPad Software, San Diego, CA). Values of p < 0.05 were considered statistically significant.
Results
Hematocrit
The Hct value of all rats at baseline was 41.2 ± 2.6%, and decreased to 27.9 ± 2.8% after 60% hemorrhage due to auto-transfusion (). After fluid resuscitation, the Hct values in the HES 130 and PolyPHb/HES groups were reduced to 13.4 ± 1.2% and 13.7 ± 1.5% respectively, due to the dilution of the blood with the different solutions. After 1 h, the Hct of both the groups increased to 19.6 ± 1.5% and 20.6 ± 1.6% respectively, and the differences between groups were not statistically significant. The changes in the Hct clearly demonstrated a good accordance with the hemorrhage and resuscitation. The Hct was reduced by approximately 70% immediately after resuscitation with both PolyPHb/HES and HES 130 alone, compared with baseline.
Survival
shows the survival rate of Sprague–Dawley rats after resuscitation with PolyPHb/HES and HES alone during the course of the experiment. Four of the ten animals in the HES 130 group survived for 72 h after resuscitation until they were sacrificed, while only two of the ten rats died before 72 h in the PolyPHb/HES treatment group. Survival rate was significantly different between groups (p < 0.05). The early resuscitation with PolyPHb did provide some improvement in the appearance of the animals in the present hemorrhagic shock model.
Hemodynamic parameters (MAP and HR)
The values of MAP and HR recorded at baseline, during shock, and after resuscitation are given in . There was no significant difference between the PolyPHb/HES and HES 130 groups at baseline. Hemorrhage significantly reduced MAP and HR in both groups, and revealed no significant differences between groups.
Table II. Mean arterial pressure (MAP) and heart rate (HR).
MAP of the animals before hemorrhage was 125 ± 6 mmHg on average, and declined to 30 ± 6 mmHg after 60% hemorrhage (the data have been pooled since there was no statistical difference between groups). Immediately after resuscitation, the MAP of the HES 130 group recovered to 93 ± 8 mmHg. The MAP of the PolyPHb/HES group recovered to 111 ± 6 mmHg, which was significantly higher than that of the HES 130 group (93 ± 8, p < 0.05). After 60 min, PolyPHb/HES still maintained a statistically higher MAP compared with HES 130 (p < 0.05).
The arterial pressure curve was used to monitor the HR of rats. The HR of all rats decreased during the hemorrhage, from a mean baseline value of 449 ± 18 beats/min to 371 ± 26. The HR of both groups gradually returned to the initial level after resuscitation and there was no significant difference compared with baseline after 1 h of observation.
Arterial blood gas parameters
The changes in arterial blood gas are shown in . Hemorrhagic shock induced significant metabolic acidosis, with base deficit and pH decrease. Base excess decreased from 3.7 ± 1.4 mmol/L at baseline to − 12.6 ± 2.8 mmol/L on average, in shock. PaO2 was statistically significantly increased after hemorrhagic shock in both groups as compensatory hyperventilation, which also led to the decrease in PaCO2. There were no significant differences between the PolyPHb/HES and HES 130 groups.
Table III. Arterial blood gas during the experiment.
After fluid resuscitation, animals in both groups tended to recover arterial blood gas variables and acid-base balance from the hyperventilation and acidosis. The pH in both groups did not show immediate normalization after resuscitation, but completely recovered at 1 h. The BE levels were partially restored from hemorrhagic shock after resuscitation with PolyPHb/HES and HES 130, but the values were still significantly lower than those at baseline after 1 h (p < 0.05). All animals were not able to recover the PaCO2 from hemorrhagic shock (p < 0.05 vs. baseline). The pH, BE, and PaCO2 did not show any significant difference during the experiment between groups. Rats in the PolyPHb/HES group tended to normalize their PaO2 after resuscitation. However, the recovery was not complete and the PaO2 remained at about 28% above baseline after 1 h, while the animals that received HES 130 alone continued to hyperventilate.
Tissue oxygen partial pressure
As shown in , hemorrhagic shock led to a severe decrease of subcutaneous PtO2 in this study, declining to 0–2 mmHg. The infusion of HES 130 or PolyPHb/HES increased the PtO2 immediately. After 15 min of resuscitation, the PtO2 in the PolyPHb/HES group rose to 14.3 ± 2.4 mmHg (about 50% of the baseline), which was significantly higher than that in the HES 130 group (6.0 ± 1.5, p < 0.05). However, the PtO2 was not able to sustain, and gradually decreased to 7.9 ± 2.0 mmHg after 1 h, but it was still higher than that of the animals that received HES 130 alone (4.5 ± 2.4, p < 0.05).
Plasma EPO levels
EPO is the principal hormone that mediates hematopoietic responses to hypoxia, regulated by HIF activity. The rat plasma EPO levels in the present study are shown in . In the HES 130 group, hypoxia induced by hemorrhagic shock resulted in an increase of plasma EPO levels starting at 10 h post resuscitation, which increased continuously and reached maximum at 48 h post resuscitation, while the increase of plasma EPO in animals resuscitated with PolyPHb and HES solutions (in a ratio) were completely inhibited during the first 10 h, and started to rise at 16 h post resuscitation. However, after 24 h, the plasma EPO levels in the PolyPHb/HES group rose dramatically and reached the same maximum at 48 h post resuscitation. After 72 h of observation, the EPO levels in HES 130 group were found to be higher than in the PolyPHb/HES group.
Discussion
The principal finding of the present study was that early resuscitation from hemorrhagic shock by infusion of 6% PolyPHb and 6% HES 130 (in a 1/2 ratio) doubled animal survival rate when compared to results obtained using HES 130 alone. The animals in the PolyPHb/HES group also showed better performances on restoration of MAP and PaO2, and greater improvement of tissue oxygenation than in the HES 130 group. Similar outcomes have been previously reported for other various HBOCs, such as PEGylated albumin-heme (CitationHuang et al. 2006), polymerized bovine hemoglobin (PBH) (CitationCabrales et al. 2009), polyethylene glycol-modified human hemoglobin (CitationWettstein et al. 2003), and hemoglobin-vesicles (CitationSakai et al. 2004). This study provided evidence that a moderate amount of PolyPHb can improve resuscitative efficacy of HES in an acute hemorrhagic shock model.
In the present experimental design the actual concentration used of polymerized human placenta hemoglobin was 2 g/dl, which is relatively low as an oxygen-carrying resuscitation fluid. High concentration of HBOCs (> 10 g Hb/dl) were usually used in the early studies of blood substitutes, for their high oxygen-carrying capacity. However, safety and side effects limited the clinical application of HBOCs, especially when used in large doses (CitationAlayash 2014). Other studies have shown that the presence of a low concentration of PBH (1.2 g Hb/dl) in the circulation was more effective in increasing oxygenation than large concentrations (> 2 g Hb/dl), or in the absence of PBH (CitationCabrales et al. 2008). A moderated concentration of HBOC provided superior restoration of systemic and microhemodynamic parameters in small-volume resuscitation from hemorrhagic shock when compared with a greater amount of HBOC (CitationCabrales et al. 2009).
PolyPHb is a nonspecific glutaraldehyde-polymerized placenta Hb, with high oxygen affinity (P50 of 11 mmHg), which results in a material that tends to release oxygen in settings of relatively low tissue oxygen tension. It is vasoactive as well, which may lead to vasoconstriction due to nitric oxide (NO) scavenging (CitationWinslow 2003). Our results show that PolyPHb has positive effects on improved outcomes when used in the early resuscitation from hemorrhagic shock, in comparison to a non-oxygen-carrying colloidal resuscitation fluid (HES 130 alone), though it is vasoactive. In addition, the vasoconstriction can be limited by controlling the concentration of HBOCs. This point was supported by data of MAP in this study, which showed that PolyPHb recovered and maintained a relatively high MAP but not higher than that of baseline. In prehospital and combat settings, when standard resuscitation procedures are unavailable, the vasoactivity associated with this type of oxygen-carrying plasma expander (as PolyPHb) may be a positive factor for maintaining blood pressure and ensuring perfusion (CitationVazquez et al. 2011). Resuscitation with HBOCs is appealing in that their use can restore both BV and tissue oxygenation. The efficacy and functionality of colloidal plasma expanders may be improved by using HBOCs to provide oxygen-carrying capacity.
In conclusion, resuscitation with PolyPHb/HES provides 80% survival in an acute model of hemorrhagic shock, while an identical procedure using a HES-based plasma expander leads to 60% mortality. PolyPHb maintains relatively high blood pressure and peripheral tissue oxygenation when compared with HES 130 alone. It is realized that the ideal resuscitative fluid should possess both volume expansion effect and oxygen-carrying capacity. Our results indicated that early resuscitation from hemorrhagic shock by infusion of moderate PolyPHb improved resuscitative efficacy of HES. We believe that HBOCs could be the most promising early resuscitation fluids, which are indispensable in prehospital settings, military conflicts, and in other situations where blood is unavailable or is not an option.
Acknowledgements
This study was supported by grants from the National Nature Science Foundation of China (No. 31271229 and 11072275) and the National High Technology Research and Development Program of China (No. 2012AA021903).
Declaration of interest
The authors report no conflicts of interest. The authors alone are responsible for the content and writing of the paper.
References
- Alayash AI. 2014. Blood substitutes: why haven't we been more successful? Trends Biotechnol. 32:177–185.
- Cabrales P, Tsai AG, Intaglietta M. 2008. Isovolemic exchange transfusion with increasing concentrations of low oxygen affinity hemoglobin solution limits oxygen delivery due to vasoconstriction. Am J Physiol Heart Circ Physiol. 295:H2212–2218.
- Cabrales P, Tsai AG, Intaglietta M. 2009. Polymerized bovine hemoglobin can improve small-volume resuscitation from hemorrhagic shock in hamsters. Shock. 31:300–307.
- Chang TM. 2005. Therapeutic applications of polymeric artificial cells. Nat Rev Drug Discov. 4:221–235.
- Elmer J, Alam HB, Wilcox SR. 2012. Hemoglobin-based oxygen carriers for hemorrhagic shock. Resuscitation. 83:285–292.
- Huang Y, Komatsu T, Yamamoto H, Horinouchi H, Kobayashi K, Tsuchida E. 2006. PEGylated albumin-heme as an oxygen- carrying plasma expander: Exchange transfusion into acute anemia rat model. Biomaterials. 27:4477–4483.
- Li T, Yu R, Zhang HH, Wang H, Liang WG, Yang XM, et al. 2006. A method for purification and viral inactivation of human placenta hemoglobin. Artif Cells Blood Substit Immobil Biotechnol. 34: 175–188.
- Li T, Zhang Z, Wu W, Liao D, Chen Y, Li S, et al. 2013. Resuscitation with polymerized human placenta hemoglobin attenuated hemorrhagic shock-induced lung injury. Artif Cells Nanomed Biotechnol. 41:27–31.
- Li T, Li J, Liu J, Zhang P, Wu W, Zhou R, et al. 2009. Polymerized placenta hemoglobin attenuates ischemia/reperfusion injury and restores the nitroso-redox balance in isolated rat heart. Free Radic Biol Med. 46:397–405.
- Murdock AD, Berseus O, Hervig T, Strandenes G, Lunde TH. 2014. Whole blood: the future of traumatic hemorrhagic shock resuscitation. Shock. 41 Suppl 1:62–69.
- Sakai H, Masada Y, Horinouchi H, Yamamoto M, Ikeda E, Takeoka S, et al. 2004. Hemoglobin-vesicles suspended in recombinant human serum albumin for resuscitation from hemorrhagic shock in anesthetized rats. Crit Care Med. 32:539–545.
- Squires JE. 2002. Artificial blood. Science. 295:1002–1005.
- Vazquez BY, Hightower CM, Martini J, Messmer C, Frienesenecker B, Corbels P, et al. 2011. Vasoactive hemoglobin solution improves survival in hemodilution followed by hemorrhagic shock. Crit Care Med. 39:1461–1466.
- Wettstein R, Tsai AG, Erni D, Winslow RM, Intaglietta M. 2003. Resuscitation with polyethylene glycol-modified human hemoglobin improves microcirculatory blood flow and tissue oxygenation after hemorrhagic shock in awake hamsters. Crit Care Med. 31:1824–1830.
- Wettstein R, Tsai AG, Harder Y, Erni D, Intaglietta M. 2006. Early resuscitation with polymerized bovine hemoglobin reverses acidosis, but not peripheral tissue oxygenation, in a severe hamster shock model. Shock. 26:496–503.
- Winslow RM. 2003. Alternative oxygen therapeutics: products, status of clinical trials, and future prospects. Curr Hematol Rep. 2: 503–510.
- You Z, Li Q, Li B, Yang C, Liu J, Li T. 2014. Isovolemic hemodilution with glutaraldehyde-polymerized human placenta hemoglobin (PolyPHb) attenuated rat liver ischemia/reperfusion injury. Artif Cells Nanomed Biotechnol. 42:83–87.
- Zhao L, Wang B, You G, Wang Z, Zhou H. 2009. Effects of different resuscitation fluids on the rheologic behavior of red blood cells, blood viscosity and plasma viscosity in experimental hemorrhagic shock. Resuscitation. 80:253–258.
- Zhou W, Li S, Hao S, Liu J, Wang H, Yang C. 2014. An optimal polymerization process for low mean molecular weight HBOC with lower dimer. Artif Cells Nanomed Biotechnol.18:1–4.
- Zhou W, Zhao L, Wang J, Li S, Chen G, Liu J, Yang C. 2013. An optimal polymerization conditions for poly-human placenta hemoglobin with lower mean molecular weight. Artif Cells Nanomed Biotechnol. 41:289–292.