Abstract
Clinically, the therapeutic outcomes in neurodegenerative disorders (NDs) by drug treatment are very limited, and the most insurmountable obstacle in the treatment of NDs is the blood–brain barrier (BBB), which provides the highest level of protection from xenobiotics. A great deal of attention still needs to be paid to overcome these barriers, and surface-engineered polymeric nanoparticles are emerging as innovative tools that are able to interact with the biological system at a molecular level for the desired response. The present review covers the potential importance of surface-structure-engineered nanoparticles to overcome the BBB for good bioavailability, and the evaluation of drug therapy in NDs.
Introduction
Neurodegeneration, which is the persistent and progressive loss of the function and structure of neurons, is a frequently occurring condition including Alzheimer's disease (AD), Parkinson's disease (PD), amyotrophic lateral sclerosis, and Huntington's disease (HD). In all cases of degenerative disorders, the aggregation of protein is a distinctive feature. The interesting observation in all the conditions mentioned above is that nearly the same type of protein has been found to be involved in different disorders of ND, seemingly following the same pathway in different disorders, while involvement of environmental factors (heavy metals, pesticides) is also speculated in different NDs () (CitationCicchetti et al. 2009).
Among NDs, the conditions of AD and PD are the most prevalent (CitationZhou 2010), and both lead to high mortality and morbidity in developed countries (CitationHebert et al. 2001, Citation2003). Despite the presence of different well-developed diagnostic and treatment tools, successful treatment still seems to be challenging. Due to an increase in the aging population, the number of patients with AD and PD may also rise in the coming years. On the other hand, although a number of drugs have been developed to treat NDs, only a few of them reach the market (CitationPardridge 2002). The limited potential of many pharmaceutical entities results in unsatisfactory treatment. In neurodegeneration, the death of a specific type of neuron is the result of multiple deleterious molecular and cellular events, rather than a single pathological factor. A number of strategies have been employed in recent years, including the direct surgical administration of drugs into the brain, osmotic opening of the tight junctions, reversible disruption of the blood–brain barrier (BBB) by different nanocarriers, the use of prodrugs, and carrier-mediated transport (CitationWagner et al. 2012, CitationPardridge 1988, CitationNeuwelt 1989, CitationBegley 2004), followed by the use of polymeric nanoparticles and solid lipid nanoparticles, which are able to cross the BBB. Nanoparticles not only cross the BBB, but also protect the active drug from degradation and minimize the side effects (CitationKreuter et al. 1995) (). Alternative routes of delivery are the intraventricular/intrathecal route and the olfactory pathway. The surface modification of nanoparticles by apolipoprotein is also an effective way to cross the BBB (CitationKreuter et al. 2007, CitationPetri et al. 2007).
Table I. Advantages of surface-modified nanoparticles over unmodi-fied nanoparticles (CitationHuang et al. 2013, CitationGaoet al. 2006).
Blood–brain barrier
The BBB is a specialized biological, highly dynamic, and physically efficient protective barrier interface between the blood and the central nervous system (CNS). Tight junctions, consisting of barrier cells called capillary endothelial cells, hinder the transcellular passage, while adherent tight junctions restrict para-cellular flux of charged molecules and molecules of high molecular weight over the brain parenchyma, while low molecular weight lipophilic molecules are able to enter the CNS by passive diffusion (CitationPaolino et al. 2010). The BBB comprises the brain microvascular endothelial cells (BMECs), pericytes, and astrocytes, and neuronal processes that cover most parts of the brain side of BMECs. Permeability across the BBB is limited because of the BMECs, and only receptor-mediated and carrier-mediated transporters are allowed in through the blood side (luminal), along with various efflux transport systems (CitationBrightman 1977, CitationBanks and Kastin 1990, CitationTamai and Tsuji 2000). It has also been shown that the BMEC express a different type of enzyme which is also used to develop the restriction across the BBB (CitationBodor and Buchwald 1999). Tight endothelial cells were found to have a gap size of up to 600 nm in diameter in tumor-implanted mice (CitationHobbs et al. 1998). The BBB functions to protect the brain against xenobiotics, cytotoxins, peripheral neurotransmitters, and microorganisms.
A variety of strategies have been tried to overcome the BBB, for example, invasive techniques or implantation, drug modification, permeability enhancement (CitationPatel et al. 2009), and coupling of drug with a substance which can pass the BBB. Small lipophilic molecules (with a molecular weight less than 400–500 Da) are able to cross, while the charge-bearing hydrophilic molecules require a gated channel, ATP protein, or receptor to cross the BBB (CitationPardridge 2006). The transporters yet to be identified are for the sugars, amino acids, nucleosides, monocarboxylic acids, organic anions, and organic cations, and include the organic cation transporters, organic anion transporters (polypeptides), glucose transporters, peptide transporters, ATP-binding cassette, and P-glycoprotein, which are mostly expressed in brain. Various efflux transporters (ASCT2, NET, OAT3) are present at the albuminal site of the endothelial cell membrane and they are used to efflux the metabolites and neurotoxic compounds that are developed in the brain (CitationOhtsuki and Terasaki 2007). Among different approaches that do not interfere with route functioning are the receptor and adsorption-mediated transcytosis, the most attractive mechanisms to facilitate the transport across the BBB (CitationBhaskar et al. 2010). For successful brain delivery, many tasks need to be considered, for example, surface decoration, prolonged half-life, bypassing reticuloendothelial uptake (RES), biocompatibility and non-immunogenicity, protection from enzymatic degradation, and the different orders of targeting (cell-, tissue-, and organ-specific) that can be achieved ().
Table II. Various pharmaceutical approaches to overcome the BBB.
Neurodegenerative disorders
Alzheimer's disease
AD is a brain disorder causing the most common type of dementia in the elderly, and is characterized by the formation two protein aggregates—neurofibrillary tangles and senile plaque—which are the main factors in the progressive neuronal degeneration and death. The senile plaques are developed by deposition, in the human brain, of fibrils of amyloid-β peptide, derived from the proteolytic processing of the amyloid precursor protein. Neurofibrillary tangles are a complex filamentous network, and tau protein was found to be the major component of this complex network. Cyclin-dependent kinase (Cdk5) and glycogen synthase kinase (GSK3β) have been shown to be involved in tau phosphorylations. Cdk5 is associated with neurogenesis and plays an important role in brain development. Extracellular amyloid loading induces the deregulation of protein kinase, which results in tau hyper-phosphorylation, further triggering cascades of events that lead to neurodegeneration; as evidenced, inhibition of Cdk5 and GSK3b exhibits protection against neurodegeneration, which, along with oxidative stress, constitutes an important factor in the modification of the general signaling pathway in neurons, causing the neurodegeneration and leading to structural and biochemical abnormalities, which seem to be related to the pathogenesis of AD (CitationMaccioni et al. 2001).
Parkinson's disease
PD is a state of progressive degeneration of midbrain nigrostriatal dopaminergic neurons, which results in a reduction in dopamine (DA) level in the brain, characterized by motor and non-motor symptoms. Selective degeneration of the nigrostriatal dopaminergic pathway is one of the key features known of PD, and the key motor symptoms of PD are the bradykinesia and rigidity. The highly affected areas in the brain include the basal ganglia, brainstem, and cerebellum, which may be associated with tremors. Substantia nigra pars compacta (SNpc) neurons were found to form the nigrostriatal dopaminergic pathway, and the loss of SNpc neurons results in striatal DA deficiency, which is responsible for most symptoms of PD; the striatal DA deficiency or striatal damage may result in the syndrome of Parkinsonism.
The first hereditary connection was linked to the protein α-synuclein, and its genetic locus on chromosome 4 is now known as PARK1. Other additional loci, namely PARK2 through PARK12, have also been implicated. The mutation in the α-synuclein gene leads to the amino acid substitution, as was seen in a family with an early-onset autosomal form of PD; α-synuclein is considered to be a major factor in the pathogenesis of PD (CitationPolymeropoulos et al. 1997, CitationSpillantini et al. 1997). Currently, the first choice of therapy is the dopamine agonist levodopa; others are the peripheral decarboxylase inhibitors carbidopa and benserazide, dopaminergic agonists such as bromocriptine, pergolide, pramipexole, MAO inhibitors such as selegiline, and dopamine facilitators such as amantadine.
Huntington's disease
HD is a devastating chronic autosomal dominant, progressive and fatal ND cause by a single mutation on the HTT gene, resulting in the repeat expansion of CAG trinucleotide (36 or more repeats), which encodes the huntingtin protein (containing more than 3000 residues). HD is characterized by weight loss, behavioral changes (chorea, dementia), dystonia, severe motor impairments, and cognitive dysfunction, as a result of brain atrophy and progressive loss of striatal neurons. Neuronal loss has also been observed in the thalamus, subthalamic nucleus, and substantia nigra pars reticulate in advanced cases of HD (CitationFerrante et al. 1985, CitationRubinsztein 2002). Pathologically, HD is characterized by neuronal loss in the cortex and striatum, and the other highly affected area includes the hippocampus. The mutation of the HTT gene in production of huntingtin protein further develops the aggregates within cells, which ultimately produce the toxic, insoluble, and filamentous neuronal intra-nuclear inclusions (NIIs) in the nucleolus of the cell (CitationGil-Mohapel et al. 2011). For the symptomatic management of movement disorder in HD, the most widely used drugs are olanzapine, risperidone, quetiapine, sulpiride, haloperidol, clonazepam, and sodium valproate. The psychiatric symptoms of HD are managed with aripiprazole, citalopram, fluoxetine, paroxetine, sertraline, venelafaxine, limotrigine, and carbamazepine.
Mechanisms involved in penetration of nanoparticles through the BBB
Adsorptive-mediated transcytosis
Adsorptive-mediated transcytosis is associated with interaction between ligand and charge at the luminal surface of endothelial cells. Penetrating peptides and cationic proteins, for example, albumin, are generally used for surface modification of the developed nanocarrier to enable it to cross the BBB ().
Receptor-mediated transport
Endothelial cells of the BBB express the receptor-mediated transport (RMT) system to transport the large molecules by transcytosis to the CNS. It develops the vesicles and dispenses their content on the luminal site of the BBB. RMT of large molecules covers the proteins, nanoparticles, enzymes, liposomes, and genes. RMT is a mechanism based on the receptors expressed at the BBB (endothelial cells) which recognize a few substances and allow them across the BBB; for example, apolipoprotein E (Apo E), transferrin, macroglobulin, and monoclonal antibodies including the OX26, RD3, and R17217 are the biomolecules used for the surface modification to act as a directive unit across the BBB (CitationKim et al. 2007). Various strategies have been used to overcome the BBB, and among them, RMT has been found to be effective, in various studies. The RMT system is designed in such a way that the drug-loaded carrier is appended with the suitable ligands which have high affinity to the receptors, for example, insulin receptors, low density lipoprotein receptors, and transferrin receptors present at the drug entry sites of BBB.
Carrier-mediated transport
Carrier mediated transport (CMT) allows a few small molecules, hormones, and amino acid nutrients across the BBB, in a concentration gradient manner. A CMT transporter is capable of selecting the substrate and carrying it via uni- or bidirectional mono-, co-, or counter transport. The transport rate is associated with substrate affinity toward the transporters (CitationDe Boer and Gaillard 2007, CitationSmith 1993). Many reports have been published in recent years concerning this area, on the structural similarities between the endogenous transporter substrate and the therapeutic molecules (CitationTamai and Tsuji 2000).
Importance of surface-modified nanoparticles
Nanoparticles used for the delivery system are of 10–1000 nm in size, with the capacity for drug delivery to different parts of the body; nanoparticles have gained a lot of attention in recent decades for use in delivery across the BBB, because they are easy to modify according to the physiological requirement, and help in increasing the understanding of receptors, which would facilitate great development in nanotechnology and polymer chemistry. Among different nanocarriers, nanoparticles have been extensively exploited in drug delivery across the BBB and a few of them are under clinical trials ().
Figure 3. Surface-modified nanoparticles (A) Antibody-coated (B) Ligand-appended (C) Cyclodextrin-modified (D) PEGylated nanoparticle.
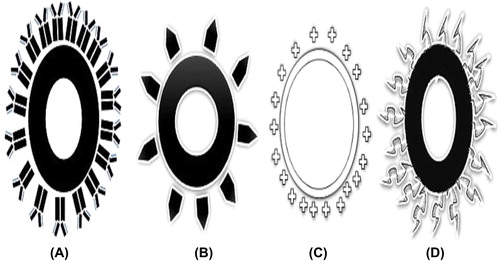
The number of different scientific studies based on surface modification of the nanoparticles for application in brain drug delivery has drastically increased in the recent past few decades, and the nanoparticles are designed in such a way that they are able to overcome many of the problems associated with the traditional carriers. The properties which make surface-modified nanoparticles as a first choice of carrier are, for example, their ability to maintain the integrity of the loaded protein, peptide, gene, MAbs and many more payloads; site-specific delivery, which minimizes the severe side-effects associated with model drug; better uptake by the endothelial cells, ability to cross the BBB and modify the function of the efflux pump, and excellent control over the release of the drug for a longer period of time. All these characteristics make the drug efficacious and effective and may minimize the resistance to some extent. More specifically, the surface-modified nanoparticles are able to deliver the drug into the CNS by opening the tight endothelial junctions or by direct transport by transcytosis, or by retaining at the BBB and improving the absorption against the concentration gradient across the endothelial cell layer, or by solubilizing the lipid on the membrane of endothelial cells and on particle coating (CitationBarbu et al. 2009) ().
Polymeric nanoparticles represent a potential means for transport across the BBB, and are designed in such way that they target the different receptors present at the BBB, ultimately enhancing the brain uptake by endothelial cells. Nanoparticles of different levels of hydrophobicity are effectively coated with a plasma component, for example, albumin or immunoglobulin, and can also be selected on the basis of their ability to bypass the phagocytic cells (CitationMoghimi et al. 2001, CitationOgawara et al. 2001, CitationFurumoto et al. 2004). The other different approaches in the area of surface modification include the use of PEG and its derivatives. Various studies support the observation that coating reduces the uptake of nanoparticles in the liver and increases their circulation time (CitationLeu et al. 1984, CitationIlium et al. 1986, CitationDouglas et al. 1986, CitationPeracchia et al. 1998, Citation1999, CitationGref et al. 1994).
Properties required by nanoparticulate carriers to cross the BBB
While considering the successful brain uptake of the drug, lipophilicity is the main factor for transport across the BBB; however, high lipophilicity leads to trapping inside the membrane, which results in a reduced rate of transport. On the other hand, the transport of a substance through the BBB is inversely proportional to its molecular weight, which seems to be a great limiting factor in crossing the BBB. Limited transport was observed in the case of ionization of acidic molecules, while ionization of the basic group has no significant effect. Log P values between − 0.2 and + 1.3 were shown to be optimal for the successful transport through the BBB. Negative charge-bearing hydrophobic molecules promote protein adsorption and activate the complement system (CitationMoghimi et al. 2001). However, in other studies, it has been reported that positive charge-bearing nanoparticles may interact with negative charge-bearing cells that lie in the outer layer of the BBB.
Surface-modified nanoparticles in neurodegenerative disorders
The dual-function nanoparticles, based on the PEGylated poly(lactic acid) (PLA) polymer conjugated with targeting, cell-penetrating peptides on the surface, have been shown to be valuable targeting tools for diagnosis and therapy. The development of effective strategies to enhance the short interfering RNA (siRNA) nose-to-brain delivery system, combined with cell-penetrating, peptide-modified nano-micelles (cell penetrating peptides or CPP) comprising the polyethylene glycol-polycaprolactone (MPEG-PCL) copolymer conjugated with the cell-penetrating peptide, and the in vivo results, all show that MPEG-PCL increases the nose-to-brain delivery as compared to the intravenous route of administration, which shows the cell penetrating property of CPP along with the modified micelles (CitationKanazawa et al. 2013). The nanoparticles capable of permeating the BBB to target the cerebrovascular Aβ proteins are developed as immuno-nanovehicles, which are chitosan-coated PLGA nanoparticles conjugated with the novel anti Aβ antibody. Studies show the enhanced uptake at the BBB and better targeting of the Aβ protein, which, along with chitosan, enhances the aqueous dispensability and stability and finally enables successful delivery of the therapeutic and diagnostic agent to the cerebral vascular amyloid (CitationJaruszewski et al. 2012). Superparamagnetic iron oxide nanoparticles (SPIONs), used in magnetic resonance imaging for AD are made efficient contrast agents for AD using 1,1-dicyano-2-[6-(dimethylamino)naphthalene-2-yl]propene (DDNP) carboxyl derivative to functionalize the SPION surface. The DDNP–SPIONs are prepared by conjugating the DDNP carboxyl derivative to oleic acid-treated SPIONs through ligand exchange. The combination was found to induce the fluorescence enhancement of the DDNP–SPIONs and displayed tremendous promise for use as a contrast agent for MRI of AD (CitationZhou et al. 2014). A novel nanohybrid (surface-modified fluorescent nanocrystal) prepared by using l-glutamic and l-aspartic (as surface-capping agents) quantum dots bio-conjugated with the surface-active neurotransmitter moieties can function as a potential biomarker in cell signaling and cell targeting (CitationMansur et al. 2013). Vitamin E (D-α-tocopheryl polyethylene glycol 1000 succinate, TPGS)-modified PLGA nanoparticles were prepared as a promising carrier to treat neurological symptoms associated with neurological disorders. TPGS is well known as an emulsifier, and degradation of nanoparticles may release the TPGS component, which has a synergistic activity (CitationJalali et al. 2011). The lactoferrin (Lf)-conjugated PEG-co-PCL nanoparticles are found to enhance the cellular accumulation in 16HBE14o-cells of the brain (cerebellum, olfactory tract, olfactory bulb, and hippocampus) through both caveolae-/clathrin-mediated endocytosis, and to enhance the biodistribution of protein and peptide in different parts of the brain by noninvasive nasal brain delivery in AD (CitationLiu et al. 2013). The peptide-ligand functionalized nanoliposomes were produced to facilitate the neuronal cell uptake of galantamine-loaded nanoliposomes, and the final formulation aided neuron accumulation, confirmed by fluorometry and confocal microscopy (CitationMufamadi et al. 2013). Odorranalectin (OL) (small non-immunogenic peptide)-conjugated modified cubosomes, prepared by incorporating maleimide-PEG-oleate and Gly14-Humanin (S14G-HN), serve as a model drug for AD. Here, the OL is used to eliminate the non-immunogenic character as compared to other lectins, and offers a novel, effective, and noninvasive system for brain drug delivery, especially for peptides and proteins (CitationWu et al. 2012). Curcumin, a fluorescent molecule, has high affinity for the Aβ peptide but faces the problem of low solubility in clinical use. Nanoliposomes are conjugated with curcumin, exposed at the surface of the nanoliposomes. The in vivo study has revealed that curcumin-loaded nanoliposomes are able to specifically stain the Aβ deposits in brain tissue in AD, which finally leads toward its application in diagnosis and site-specific delivery in AD (CitationLazar et al. 2013). The poly(ethylene glycol)-block-polylactide (PEG-b-PLA) nanoparticles are able to interact with the Aβ peptide, and were suitably stabilized by the sodium cholate and PEG, in a simple new approach for AD (CitationBrambilla et al. 2011). PLGA nanoparticles coated with Tween 80 can deliver estradiol to the brain upon oral administration. In an ovariectomized (OVX) rat model of AD, the nanoparticles were able to prevent the expression of amyloid β. It was observed that Tween 80-coated nanoparticles administered orally result in the same brain estradiol concentration as achieved by i.m. injection of estradiol, but lower brain estradiol concentration was observed with the administration of uncoated nanoparticles (CitationMittal et al. 2011). Strategies to improve the drug delivery to brain include the use of e peptide sequence—THRPPMWSPVWP—in the gold nanoparticle–CLPFFD conjugate. This sequence interacts with the transferrin receptor in microvascular endothelial cells of the BBB and leads to permeability of the conjugate in brain, in AD (CitationPrades et al. 2012). A 12-amino acid peptide (denoted as pep TGN) is displayed by the bacteriophage clone 12-2. The pep TGN was covalently conjugated onto the surface of poly(ethylene glycol)-poly (lactic-co-glycolic acid) (PEG-PLGA)-based nanoparticles, with great potential for site-specific delivery across the BBB. The cellular uptake and brain-targeting index of Pep TGN-modified nanoparticles was found to be significantly higher than that of unmodified nanoparticles (CitationLi et al. 2011). The formulation of chitosan nanoparticles into SNVs enhanced their transcytosis-mediated uptake at the BBB endothelial cells and their accumulation in various regions of brain. Such types of nanoparticles are capable of delivering the therapeutic agent across the BBB to target the amyloid beta (CitationAgyare et al. 2008). Rhodamine B isothiocyanate, a fluorescent probe, was used to label angiopep-conjugated poly(ethyl glycol)-co-poly (E-caprolactone) nanoparticles as a brain-targeting drug delivery system in mice, and the study results demonstrate that the ANG-PEG-NP significantly enhances the uptake by the brain capillary endothelial cells (more specifically, accumulation of ANG-PEG-NP at a higher extent in the cortical layer, lateral ventricles, and hippocampus) compared with that of PEG-NP through caveolae and clathrin-mediated endocytosis. Elaboration of this issue is important for the future development of ANG-PEG-NP drug delivery system in brain-targeting in NDs (CitationXin et al. 2012). The ApoE is adsorbed on to the surface of polysorbate 80 (Tween-80)-coated nanoparticles which may be able to enter the BBB (CitationKreuter 2001).
Furthermore, receptor-mediated transcytosis is confirmed by using the Apo E-related nonsense sequences, as these are unable to transport drug across the BBB (CitationMichaelis et al. 2006). The BBB crossing potential can also be confirmed by the data of HAS nanoparticles modified by Apo E in different parts of brain and neurons just 15 min after intravenous injection (CitationZensi et al. 2009); on comparing this with PEGylated nanoparticles which were found in endothelial junction and were absent in different parts of brain after 30 min of injection. This is evidence of the unspecificity of PEGylated nanoparticles (CitationWagner et al. 2012).
Surface-decorated nanoparticles play an important role in the management of PD, and research scientists studying therapeutic formulations are paying great attention towards efficient nanoparticle uptake, targeted delivery, minimizing the unnecessary distribution to areas other than the targeted organ, and many more such effects. More specifically, OL was recently identified as a small molecule with the least immunogenicity as compared to other molecules, and was found to have good potential when used as a ligand with PEG-PLA nanoparticles to enhance the nose-to-brain delivery of the peptide urocortin. The results show increased brain delivery of surface-modified nanoparticles and enhanced therapeutic effects, further suggesting that this could be carrier of interest for CNS delivery (CitationWen et al. 2011). In another study, the nanoparticles were developed by conjugation of Lf with PEG-PLA nanoparticles (lactoferrin thiolated and conjugated to the maleiamide function surrounding the PEGylated nanoparticles), and the presence of ligand on the surface of the nanoparticles was confirmed. The murine studies suggest more pronounced accumulation of surface-modified nanoparticles than unmodified nanoparticles, and such kinds of approach are able to attenuate the lesion in the striatum in PD (CitationHu et al. 2011).
Among different therapeutic approaches, gene therapy is also considered promising to effectively treat PD, but the BBB limits the choices. Lf-modified nanoparticles are used as gene vectors because of their BBB-crossing potential. In vivo study results show that multiple intravenous injections of Lf-modified nanoparticles obtained higher GDNF expression for a longer period of time, with significant improvement in locomotor activity and reduction in neuronal loss (CitationHuang et al. 2010). SNCA (gene encoding the alpha synuclein protein) targeting is another important treatment strategy in PD, but the challenges are to maintain efficient delivery without any toxicity and side effects. Such a goal was achieved by developing polyethylene glycol-polyethyleneimine as a vector for alpha synuclein siRNA delivery to PC12 cells in PD. The study shows that these nanoparticles have low toxicity with high transfection efficiency, and seem to have remarkable potential for gene delivery in PD (CitationLiu et al. 2014). Among various approaches, a new innovative approach is the fabrication of surface-modified polymer-lipid hybrid nanoparticles for the intranasal delivery of ropinirole hydrochloride. Such kinds of nanoparticles were developed with the aim of achieving sustained release, avoiding hepatic first pass metabolism, and enhancing the therapeutic efficacy. The results from the in vivo studies show good retention at nasal mucosa, sustained release pattern, and therapeutic efficiency. This type of carrier was found to be safe and stable for intranasal delivery of nanoparticles for effective treatment of PD (CitationPardeshi et al. 2013) ().
Table III. Various studies on surface-modified nanoparticles and their potential to overcome.
As previously discussed, in HD, the toxic huntingtin protein is expressed, and the use of SiRNA to inhibit the mutant protein was found to be an approach with good potential. Such an approach consists of modifying β-cyclodextrin nanoparticles as neuronal SiRNA delivery vectors, and the results revealed that such nanoparticles are stable in the brain and are able to reduce the expression of the huntingtin protein in rat striatal cells and in humans, while simultaneously limiting toxicity. From the in vivo results, it was reported that these modified nanoparticles are may be a vector of choice in NDs (CitationGodinho et al. 2013).
Different techniques of surface modification for the management of neurodegenerative disorders
Conjugation
The conjugation of nanoparticles with a directing unit is a creative approach to deliver the drug in a temporally and spatially controlled manner, which enhances the efficacy and minimizes the side effects by site-specific delivery. Polymer–drug conjugates were first introduced in the 1970s, and pre-clinically evaluated in the 1980s. The conjugation of PLGA with lectins involves the covalent coupling of PLGA with the ligand or other polymer. Most commonly, the polymer is suitably converted to an ester form by a reagent like N-hydroxy succinimide, in which lectin forms the covalent bond with the NH2 of lectin; in the same way, the amine group of chitosan can be replaced by the thiol group. The chitosan-nanosphere-conjugated PEG bearing the OX26 monoclonal antibody has an affinity towards the transferrin receptor, as was seen by electron microscopy in the brain of mice, and it seems to be a promising carrier for the transport of anticaspase peptide (DEVD-FMK) into the brain (CitationAktas et al. 2005). Among the different types of lectins, OL was found to be smallest lectin with low immunogenicity among the members of the traditional lectin family, capable of enhancing the nose-to-brain delivery and to reducing the immunogenicity of traditional lectins. Urocortin peptide was used as a macromolecule model drug and its therapeutic efficacy on rats was evaluated. The results suggest the increment in brain delivery and enhanced therapeutic effects in PD (CitationWen et al. 2011). The maleimide-mediated (to enhance nasal absorption) wheat gram agglutinin (lectin)-functionalized PEG-PLA nanoparticles, with intranasal administration, was found be present in different areas of brain tissue, at a quantity two-fold that of unmodified nanoparticles), as proved by the fluorescent marker. Such techniques offer an effective noninvasive system for protein and gene delivery to the brain (CitationGao et al. 2006).
Surface-modified nanoparticles as non-viral gene vectors
Angiopep-conjugated dendrigraft poly-L-lysine nanoparticles were prepared to investigate the potential application in gene delivery in PD by taking advantage of the affinity of angiopep (a ligand) towards the low density lipoprotein receptor protein-overexpressed luminal site of the BBB. Angiopep-modified nanoparticles exhibit higher uptake and gene expression, and apparent recovery of dopaminergic neurons, as compared to unmodified nanoparticles; such an approach may be attractive for long-term gene therapy in different NDs (CitationHuang et al. 2013). Bharali and coworkers have shown the ability of nanoparticles to effectively traverse the biological barrier and transfect cells in vivo; more specifically, organically modified silica nanoparticles surface-functionalized with amino groups (as a non-viral vector), for efficient in vivo gene delivery. After fours week of fluorescent visualization, the transfection was observed in the substantia nigra, and the level of this non-viral vector was equal to that of the viral vector (CitationBharali et al. 2005).
Surfactant coating
The concept of surfactant-coating of nanoparticles was first introduced by Troster and coworkers who hypothesized the body distribution of coated nanoparticles (CitationTröster and Kreuter 1988). On the basis of this, later work carried out by same group studied the in vivo distribution of surfactant-coated nanoparticles of PMMA of a size of 131 nm (CitationTröster et al. 1990). In the later phase, different types of surfactant, including the different types of poloxamers 188, 407, 184, 338, and 908, and polysorbate 20, 60, and 80 were being widely used to identify the possible relationship between the surface property of nanoparticles and their bio-distribution. The potential of surfactant-modified nanoparticles was confirmed first by the developing the P80-coated poly(butyl cyano acrylate) nanoparticles to effectively cross the BBB and achieving good therapeutic response as compared to intravenous administration of the same. Poly(butylated cyano acrylate) nanoparticles were among the carriers which were studied in various drug delivery applications, and surface-functionalization by polysorbate 80 was shown to enable crossing of the BBB by receptor-mediated endocytosis on adsorption of the apolipoprotein from blood (CitationFriese et al. 2000).
On the mechanistic aspects, different explanations for how these surfactant-modified nanoparticles work have been published, which are as follows: as these nanoparticles increase the retention time or their adsorption, the concentration gradient in the wall of the capillaries enhances the transport, solubilizing the lipid of the endothelial cell membrane, opening tight junctions to enhance the paracellular transport, and inhibiting the efflux system (CitationKreuter 2004, 2005). Iron chelator (2-methyl-N-(3-aminopropyl)-3-hydroxyl-4-pyridinon) (MAPHP)-conjugated nanoparticles also behave like lipoprotein particles by preferentially adsorbing apolipoprotein A-1 and are able to cross the BBB by mimicking low density lipoprotein (LDL), to facilitate the removal of nanoparticles to remove iron from the brain tissue (from paraffin sections). Through the same (LDL) transport system, metal ion concentration is reduced at the disease-affected area (CitationLiu et al. 2006).
Polymer coating or PEGylation
PEG chains were first linked to the PLA nanoparticles by Gref and coworkers (CitationGref et al. 1994). Subsequently, PEG was chemically linked with poly(hexadecyl) cyanoacrylate nanoparticles. Both these PEGylated nanoparticles significantly prolong the blood circulation time and minimize their uptake by liver. Dextran-coated nanoparticles have also been used in different biomedical applications (CitationHuong et al. 2009).
Nanoparticles coated with PEG have gained a lot of attention. The protective properties of PEG on nanoparticles was demonstrated by Torchilin (CitationTorchilin 1998). Minakshi and coworkers introduced a novel strategy for synthesizing peptide-tagged polyethylene glycol, PEGylated chitosan polymer, to develop nanoparticles for siRNA delivery. From a mechanistic point of view, the conjugation of monomethoxy PEG, at the C2 hydroxyl group of the chitosan polymer, with conjugation of PEG to a cell-penetrating peptide transactivator of transcription (TAT), attenuates the neurodegeneration (CitationMalhotra et al. 2013).
Block polymer
A number of amphiphilic diblock copolymers like MePEG-PLA were synthesized by ring-opening polymerization of D,L-lactide, initiated with the hydroxyl group of methoxy poly(ethylene glycol), with stannous octoate used as catalyst. The chemical structure of the conjugate was confirmed by NMR (CitationZhang et al. 2004).
Effect of formulation variables on the functioning of surface-modified nanoparticles
It has been observed in various studies that on conjugation, the particle showed an increase in diameter. During formulation, it is necessary to consider that the flexibility of the directing unit (ligand) might enhance the receptor recognition and binding capacity (CitationOlivier et al. 2003). The ligand density on the surface of nanocarrier has a great effect on the brain uptake of the nanocarrier. The neuronal cell uptake of ligand-appended nanoparticles at 37°C (which is important for normal cellular functioning) was higher as compared to that at 4°C (at this temperature, all active, energy-consuming transport processes are stopped) (CitationWagner et al. 2012), suggesting the uptake of nanoparticles in a temperature-dependent manner. With regard to the concentration, at 37°C, probe-labeled ligand-appended nanoparticles showed an increase in size with an increase in concentration, while at 4°C, on increasing the concentration, saturation concentration was easily achieved at lower dose of both ligand-appended nanoparticles and non-ligand-appended nanoparticles, showing no significant difference between the two types of nanoparticles. In a qualitative uptake study using fluorescent microscopy, the ligand-appended nanoparticles showed higher fluorescent intensity than that of the non-ligand-appended nanoparticles. Sometimes, the complex formation between fluorescent dye and the copolymer may produce negative results, and the incubation time may also affect the fluorescence (CitationDemeule et al. 2002). The best way to enhance the circulation time is PEGylation, and on increasing the density of PEG on nanoparticle surface, the RES uptake can be avoided to a higher extent. Jalali and coworkers used the PLGA nanoparticle with vitamin E (d-α-tocopheryl polyethylene glycol 1000 succinate, TPGS) as an effective emulsifier for the delivery of drugs (both hydrophilic and lipophilic) to the brain. In addition to having the desired surface morphology, several studies have demonstrated the effect of TPGS as an absorption enhancer, and vitamin E is also a free radical scavenger in the brain (CitationAmano et al. 1994, CitationJalali et al. 2011).
Conclusion
Different kinds of surface modifications of nanoparticles are of great interest from the pharmacological as well as pharmaceutical points of view, offering an impressive resolution not only for the NDs but also for different types of life-threatening diseases. These modified nanocarriers can potentially act at a molecular level to attenuate the premature death of neurons and also afford the observation of the molecular basis of the disease and the associated events which may give rise to new pathways of disease-causing factors. The targeting ability of nanoparticles can be improved without affecting the normal physiological body events, by selecting the ligands which simulate already existing compounds in our body, and these ligands, though from an external source, have an affinity toward the specific receptor present at the target site. In neurodegeneration, the gap developed by the neuronal loss can be filled by developing artificial cells in order to retain the long term memories. The developed nanocarriers are also associated with some serious problems, more specifically, the reversible disruption of the BBB allows the entry of endogenous as well as foreign particles. Such aspects need to be considered in the design and development of nanocarriers, and such disruptions can also be modified for the limited entry of specific carriers and the required nutrients.
Acknowledgement
The authors are thankful to Mr. Praveen Garg, Chairman, ISF College of Pharmacy, Moga, Punjab, for his continuous support and encouragement.
Declaration of interest
The authors report no declarations of interest. The authors alone are responsible for the content and writing of the paper.
References
- Agyare EK, Curran GL, Ramakrishnan M, Caroline CY, Poduslo JF, Kandimalla KK. 2008. Development of a smart nano-vehicle to target cerebrovascular amyloid deposits and brain parenchymal plaques observed in Alzheimer's disease and cerebral amyloid angiopathy. Pharm Res. 25:2674–2684.
- Aktas Y, Yemisci M, Andrieux K, Gursoy RN, Alonso MJ, Fernandez-Megia E, et al. 2005. Development and brain delivery of chitosan-PEG nanoparticles functionalized with the monoclonal antibody OX26. Bioconjug Chem. 16:1503–1511.
- Amano S, Ohashi M, Kirihara M, Yang XH, Hazama F. 1994. α-Tocopherol protects against radical-induced injury in cultured neurons. Neurosci Lett. 170:55–58.
- Banks WA, Kastin AJ. 1990. Peptide transport systems for opiates across the blood-brain barrier. Am J Physiol Endocrinol Metab. 259:E1–E10.
- Barbu E, Molnàr É, Tsibouklis J, Górecki DC. 2009. The potential for nanoparticle-based drug delivery to the brain: overcoming the blood-brain barrier. Expert Opin Drug Deliv. 6:553–565.
- Batrakova EV, Li S, Vinogradov SV, Alakhov VY, Miller DW, Kabanov AV. 2001. Mechanism of pluronic effect on P-glycoprotein efflux system in blood-brain barrier: contributions of energy depletion and membrane fluidization. J Pharmacol Exp Ther 299: 483–493.
- Begley DJ. 2004. Delivery of therapeutic agents to the central nervous system: the problems and the possibilities. Pharmacol Ther. 104: 29–45.
- Bharali DJ, Klejbor I, Stachowiak EK, Dutta P, Roy I, Kaur N, et al. 2005. Organically modified silica nanoparticles: a nonviral vector for in vivo gene delivery and expression in the brain. Proc Natl Acad Sci USA. 102:11539–11544.
- Bhaskar S, Tian F, Stoeger T, Kreyling W, de la Fuente JM, Grazú V, et al. 2010. Multifunctional Nanocarriers for diagnostics, drug delivery and targeted treatment across blood-brain barrier: perspectives on tracking and neuroimaging. Part Fibre Toxicol. 7:3.
- Blanchette M, Fortin D. 2011Blood-brain barrier disruption in the treatment of brain tumors. In: The Blood-Brain and Other Neural Barriers New York: Springer, pp. 447–463.
- Bodor N, Buchwald P. 1999. Recent advances in the brain targeting of neuropharmaceuticals by chemical delivery systems. Adv Drug Deliv Rev. 36:229–254.
- Borlongan C, Emerich D. 2003. Facilitation of drug entry into the CNS via transient permeation of blood brain barrier: laboratory and preliminary clinical evidence from bradykinin receptor agonist, Cereport. Brain Res Bull. 60:297–306.
- Brambilla D, Souguir H, Nicolas J, Mackiewicz N, Verpillot R, Le Droumaguet B, et al. 2011. Colloidal properties of biodegradable nanoparticles influence interaction with amyloid-β peptide. J Biotechnol. 156:338–340.
- Brightman MW. 1977. Morphology of blood-brain interfaces. Exp Eye Res. 25:1–25.
- Chauhan NB. 2002. Trafficking of intracerebroventricularly injected antisense oligonucleotides in the mouse brain. Antisense Nucleic Acid Drug Dev. 12:353–357.
- Chou KJ, Donovan MD. 1998. Lidocaine distribution into the CNS following nasal and arterial delivery: a comparison of local sampling and microdialysis techniques. Int J Pharm. 171:53–61.
- Cicchetti F, Drouin-Ouellet J, Gross RE. 2009. Environmental toxins and Parkinson's disease: what have we learned from pesticide-induced animal models? Trends Pharmacol Sci. 30:475–483.
- Cosco D, Paolino D, Muzzalupo R, Celia C, Citraro R, Caponio D, et al. 2009. Novel PEG-coated niosomes based on bola-surfactant as drug carriers for 5-fluorouracil. Biomed Microdevices. 11:1115–1125.
- Czeisler BM, Janigro D. 2006. Reading and writing the blood-brain barrier: relevance to therapeutics. Recent Pat CNS Drug Discov. 1:157–173.
- De Boer A, Gaillard P. 2007. Drug targeting to the brain. Annu Rev Pharmacol Toxicol. 47:323–355.
- Demeule M, Poirier J, Jodoin J, Bertrand Y, Desrosiers RR, Dagenais C, et al. 2002. High transcytosis of melanotransferrin (P97) across the blood–brain barrier. J Neurochem. 83:924–933.
- Douglas SJ, Davis SS, Illum L. 1986. Biodistribution of poly (butyl 2-cyanoacrylate) nanoparticles in rabbits. Int J Pharm. 34:145–152.
- Erdlenbruch B, Alipour M, Fricker G, Miller DS, Kugler W, Eibl H, Lakomek M. 2003. Alkylglycerol opening of the blood–brain barrier to small and large fluorescence markers in normal and C6 glioma-bearing rats and isolated rat brain capillaries. Br J Pharmacol. 140:1201–1210.
- Ferrante R, Kowall N, Beal M, Richardson E, Bird E, Martin J. 1985. Selective sparing of a class of striatal neurons in Huntington's disease. Science. 230:561–563.
- Friese A, Seiller E, Quack G, Lorenz B, Kreuter J. 2000. Increase of the duration of the anticonvulsive activity of a novel NMDA receptor antagonist using poly (butylcyanoacrylate) nanoparticles as a parenteral controlled release system. Eur J Pharm Biopharm. 49: 103–109.
- Furumoto K, Nagayama S, Ogawara K, Takakura Y, Hashida M, Higaki K, Kimura T. 2004. Hepatic uptake of negatively charged particles in rats: possible involvement of serum proteins in recognition by scavenger receptor. J Control Release. 97:133–141.
- Gao X, Tao W, Lu W, Zhang Q, Zhang Y, Jiang X, Fu S. 2006. Lectin-conjugated PEG–PLA nanoparticles: preparation and brain delivery after intranasal administration. Biomaterials. 27:3482–3490.
- Gil-Mohapel J, Simpson JM, Ghilan M, Christie BR. 2011. Neurogenesis in Huntington's disease: can studying adult neurogenesis lead to the development of new therapeutic strategies? Brain Res. 1406:84–105.
- Godinho BM, Ogier JR, Darcy R, O’Driscoll CM, Cryan JF. 2013. Self-assembling modified β-cyclodextrin nanoparticles as neuronal siRNA delivery vectors: focus on Huntington's disease. Mol Pharm. 10:640–649.
- Gref R, Minamitake Y, Peracchia MT, Trubetskoy V, Torchilin V, Langer R. 1994. Biodegradable long-circulating polymeric nanospheres. Science. 263:1600–1603.
- Harmon BT, Aly AE, Padegimas L, Sesenoglu-Laird O, Cooper MJ, Waszczak BL. 2014. Intranasal administration of plasmid DNA nanoparticles yields successful transfection and expression of a reporter protein in rat brain. Gene Ther. 21:514–521.
- Hebert LE, Beckett LA, Scherr PA, Evans DA. 2001. Annual incidence of Alzheimer disease in the United States projected to the years 2000 through 2050. Alzheimer Dis Assoc Disord. 15:169–173.
- Hebert LE, Scherr PA, Bienias JL, Bennett DA, Evans DA. 2003. Alzheimer disease in the US population: prevalence estimates using the 2000 census. Arch Neurol. 60:1119–1122.
- Hobbs SK, Monsky WL, Yuan F, Roberts WG, Griffith L, Torchilin VP, Jain RK. 1998. Regulation of transport pathways in tumor vessels: role of tumor type and microenvironment. Proc Natl Acad Sci. 95:4607–4612.
- Hu K, Shi Y, Jiang W, Han J, Huang S, Jiang X. 2011. Lactoferrin conjugated PEG-PLGA nanoparticles for brain delivery: preparation, characterization and efficacy in Parkinson's disease. Int J Phar. 415:273–283.
- Huang R, Ke W, Liu Y, Wu D, Feng L, Jiang C, Pei Y. 2010. Gene therapy using lactoferrin-modified nanoparticles in a rotenone-induced chronic Parkinson model. J Neurol Sci. 290:123–130.
- Huang R, Ma H, Guo Y, Liu S, Kuang Y, Shao K, et al. 2013. Angiopep-conjugated nanoparticles for targeted long-term gene therapy of Parkinson's disease. Pharma Res. 30:2549–2559.
- Huong NT, Giang LTK, Binh NT. 2009. Surface modification of iron oxide nanoparticles and their conjuntion with water soluble polymers for biomedical application. J Phys. 012046.
- Hynynen K, McDannold N, Vykhodtseva N, Raymond S, Weissleder R, Jolesz FA, Sheikov N. 2006. Focal disruption of the blood-brain barrier due to 260-kHz ultrasound bursts: a method for molecular imaging and targeted drug delivery. J Neurosurg. 105:445–454.
- Ilium L, Hunneyball I, Davis S. 1986. The effect of hydrophilic coatings on the uptake of colloidal particles by the liver and by peritoneal macrophages. Int J Pharm. 29:53–65.
- Immordino ML, Dosio F, Cattel L. 2006. Stealth liposomes: review of the basic science, rationale, and clinical applications, existing and potential. Int J Nanomedicine. 1:297.
- Jalali N, Moztarzadeh F, Mozafari M, Asgari S, Motevalian M, Alhosseini SN. 2011. Surface modification of poly (lactide-co-glycolide) nanoparticles by d-α-tocopheryl polyethylene glycol 1000 succinate as potential carrier for the delivery of drugs to the brain. Colloids Surf A Physicochem Eng Asp. 392:335–342.
- Jaruszewski KM, Ramakrishnan S, Poduslo JF, Kandimalla KK. 2012. Chitosan enhances the stability and targeting of immuno-nanovehicles to cerebro-vascular deposits of Alzheimer's disease amyloid protein. Nanomedicine. 8:250–260.
- Juillerat-Jeanneret L. 2008. The targeted delivery of cancer drugs across the blood–brain barrier: chemical modifications of drugs or drug-nanoparticles? Drug Discov Today. 13:1099–1106.
- Kanazawa T, Akiyama F, Kakizaki S, Takashima Y, Seta Y. 2013. Delivery of siRNA to the brain using a combination of nose-to-brain delivery and cell-penetrating peptide-modified nano-micelles. Biomaterials. 34:9220–9226.
- Karanth H, Murthy RSR. 2008. Nanotechnology in brain targeting. Int J Pharm Sci Nanotechnol. 1:9–24.
- Kim HR, Andrieux K, Gil S, Taverna M, Chacun H, Desmaële D, et al. 2007. Translocation of poly (ethylene glycol-co-hexadecyl) cyanoacrylate nanoparticles into rat brain endothelial cells: role of apolipoproteins in receptor-mediated endocytosis. Biomacromolecules. 8:793–799.
- Kreuter J. 2004. Influence of the surface properties on nanoparticle-mediated transport of drugs to the brain. J Nanosci Nanotechnol. 4:484–488.
- Kreuter J. 2001. Nanoparticulate systems for brain delivery of drugs. Adv Drug Deliv Rev. 47:65–81.
- Kreuter J, Alyautdin RN, Kharkevich DA, Ivanov AA. 1995. Passage of peptides through the blood-brain barrier with colloidal polymer particles (nanoparticles). Brain Res. 674:171–174.
- Kreuter J, Hekmatara T, Dreis S, Vogel T, Gelperina S, Langer K. 2007. Covalent attachment of apolipoprotein AI and apolipoprotein B-100 to albumin nanoparticles enables drug transport into the brain. J Control Release. 118:54–58.
- Lazar AN, Mourtas S, Youssef I, Parizot C, Dauphin A, Delatour B, et al. 2013. Curcumin-conjugated nanoliposomes with high affinity for Aβ deposits: possible applications to Alzheimer disease. Nanomedicine. 9:712–721.
- Leu D, Manthey B, Kreuter J, Speiser P, Deluca PP. 1984. Distribution and elimination of coated polymethyl [2‐14C] methacrylate nanoparticles after intravenous injection in rats. J Pharm Sci. 73:1433–1437.
- Li J, Feng L, Fan L, Zha Y, Guo L, Zhang Q, et al. 2011. Targeting the brain with PEG–PLGA nanoparticles modified with phage-displayed peptides. Biomaterials. 32:4943–4950.
- Licciardi M, Paolino D, Celia C, Giammona G, Cavallaro G, Fresta M. 2010. Folate-targeted supramolecular vesicular aggregates based on polyaspartyl-hydrazide copolymers for the selective delivery of antitumoral drugs. Biomaterials. 31:7340–7354.
- Liu G, Men P, Harris PL, Rolston RK, Perry G, Smith MA. 2006. Nanoparticle iron chelators: a new therapeutic approach in Alzheimer disease and other neurologic disorders associated with trace metal imbalance. Neurosci Lett. 406:189–193.
- Liu YY, Yang XY, Li Z, Liu ZL, Cheng D, Wang Y, et al. 2014. Characterization of polyethylene glycol‐polyethyleneimine as a vector for alpha‐synuclein siRNA delivery to PC12 cells for Parkinson's disease. CNS Neurosci Ther. 20:76–85.
- Liu Z, Jiang M, Kang T, Miao D, Gu G, Song Q, et al. 2013. Lactoferrin-modified PEG-co-PCL nanoparticles for enhanced brain delivery of NAP peptide following intranasal administration. Biomaterials. 34:3870–3881.
- Maccioni RB, Muñoz JP, Barbeito L. 2001. The molecular bases of Alzheimer's disease and other neurodegenerative disorders. Arch Med Res 32:367–381.
- Malhotra M, Tomaro-Duchesneau C, Prakash S. 2013. Synthesis of TAT peptide-tagged PEGylated chitosan nanoparticles for siRNA delivery targeting neurodegenerative diseases. Biomaterials. 34:1270–1280.
- Mansur AA, Saliba JB, Mansur HS. 2013. Surface modified fluorescent quantum dots with neurotransmitter ligands for potential targeting of cell signaling applications. Colloids Surf B Biointerfaces. 111: 60–70.
- Michaelis K, Hoffmann MM, Dreis S, Herbert E, Alyautdin RN, Michaelis M, et al. 2006. Covalent linkage of apolipoprotein e to albumin nanoparticles strongly enhances drug transport into the brain. J Pharmacol ExpTher. 317:1246–1253.
- Mittal G, Carswell H, Brett R, Currie S, Kumar M. 2011. Development and evaluation of polymer nanoparticles for oral delivery of estradiol to rat brain in a model of Alzheimer's pathology. J Control Release. 150:220–228.
- Moghimi SM, Hunter AC, Murray JC. 2001. Long-circulating and target-specific nanoparticles: theory to practice. Pharmacol Rev. 53:283–318.
- Mufamadi MS, Choonara YE, Kumar P, Modi G, Naidoo D, van Vuuren S, et al. 2013. Ligand-functionalized nanoliposomes for targeted delivery of galantamine. Int J Pharm. 448:267–281.
- Neuwelt E, Abbott NJ, Abrey L, Banks WA, Blakley B, Davis T, et al. (2008). Strategies to advance translational research into brain barriers. Lancet Neurol. 7:84–96.
- Neuwelt EA. 1989Implications of the Blood-brain Barrier and Its Manipulation New York: Plenum Pub Corp.
- Ogawara K, Furumoto K, Takakura Y, Hashida M, Higaki K, Kimura T. 2001. Surface hydrophobicity of particles is not necessarily the most important determinant in their in vivo disposition after intravenous administration in rats. J Control Release. 77:191–198.
- Ohtsuki S, Terasaki T. 2007. Contribution of carrier-mediated transport systems to the blood–brain barrier as a supporting and protecting interface for the brain; importance for CNS drug discovery and development. Pharm Res. 24:1745–1758.
- Olivier V, Meisen I, Meckelein B, Hirst TR, Peter-Katalinic J, Schmidt MA, Frey A. 2003. Influence of targeting ligand flexibility on receptor binding of particulate drug delivery systems. Bioconjug Chem. 14:1203–1208.
- Paolino D, Cosco D, Racanicchi L, Trapasso E, Celia C, Iannone M, et al. 2010. Gemcitabine-loaded PEGylated unilamellar liposomes vs GEMZARZ Biodistribution, pharmacokinetic features and in vivo antitumor activity. J Control Release. 144:144–150.
- Pardeshi CV, Belgamwar VS, Tekade AR, Surana SJ. 2013. Novel surface modified polymer–lipid hybrid nanoparticles as intranasal carriers for ropinirole hydrochloride: in vitro, ex vivo and in vivo pharmacodynamic evaluation. J Mater Sci Mater Med. 24:2101–2115.
- Pardridge WM. 1988. Recent advances in blood-brain barrier transport. Annu Rev Pharmacol Toxicol. 28:25–39.
- Pardridge WM. 2002. Why is the global CNS pharmaceutical market so under-penetrated? Drug Discov Today. 7:5–7.
- Pardridge WM. 2006. Molecular Trojan horses for blood–brain barrier drug delivery. Curr opin Pharmacol. 6:494–500.
- Pardridge WM. 2007. Blood–brain barrier delivery. Drug Discov Today. 12:54–61.
- Patel MM, Goyal BR, Bhadada SV, Bhatt JS, Amin AF. 2009. Getting into the brain. CNS Drugs. 23:35–58.
- Peracchia M, Fattal E, Desmaele D, Besnard M, Noel J, Gomis J, et al. 1999. Stealth® PEGylated polycyanoacrylate nanoparticles for intravenous administration and splenic targeting. J Control Release. 60:121–128.
- Peracchia MT, Vauthier C, Desmaële D, Gulik A, Dedieu J-C, Demoy M, et al. 1998. Pegylated nanoparticles from a novel methoxypolyethylene glycol cyanoacrylate-hexadecyl cyanoacrylate amphiphilic copolymer. Pharm Res. 15:550–556.
- Petri B, Bootz A, Khalansky A, Hekmatara T, Müller R, Uhl R, et al. 2007. Chemotherapy of brain tumour using doxorubicin bound to surfactant-coated poly (butyl cyanoacrylate) nanoparticles: revisiting the role of surfactants. J Control Release. 117:51–58.
- Polymeropoulos MH, Lavedan C, Leroy E, Ide SE, Dehejia A, Dutra A, et al. 1997. Mutation in the α-synuclein gene identified in families with Parkinson's disease. Science. 276:2045–2047.
- Prades R, Guerrero S, Araya E, Molina C, Salas E, Zurita E, et al. 2012. Delivery of gold nanoparticles to the brain by conjugation with a peptide that recognizes the transferrin receptor. Biomaterials. 33:7194–7205.
- Rautioa J, Chikhale PJ. 2004. Drug delivery systems for brain tumor therapy. Curr Pharm Des. 10:1341–1353.
- Rubinsztein DC. 2002. Lessons from animal models of Huntington's disease. Trends Genet. 18:202–209.
- Smith QR. 1993Drug delivery to brain and the role of carrier-mediated transport In: Frontiers in Cerebral Vascular Biology. New York: Springer, pp. 83–93.
- Spillantini MG, Schmidt ML, Lee VMY, Trojanowski JQ, Jakes R, Goedert M. 1997. α-synuclein in lewy bodies. Nature. 388:839–840.
- Su Y, Sinko PJ. 2006. Drug delivery across the blood-brain barrier: why is it difficult? how to measure and improve it? Expert Opin Drug Deliv. 3:419–435.
- Tamai I, Tsuji A. 2000. Transporter-mediated permeation of drugs across the blood–brain barrier. J Pharm Sci. 89:1371–1388.
- Temsamani J, Scherrmann JM, Rees AR, Kaczorek M. 2000. Brain drug delivery technologies: novel approaches for transporting therapeutics. Pharm Sci Technolo Today. 3:155–162.
- Torchilin V. 1998. Polymer-coated long-circulating microparticulate pharmaceuticals. J Microencapsul. 15:1–19.
- Tröster S, Kreuter J. 1988. Contact angles of surfactants with a potential to alter the body distribution of colloidal drug carriers on poly (methyl methacrylate) surfaces. Int J Pharm. 45:91–100.
- Tröster S, Müller U, Kreuter J. 1990. Modification of the body distribution of poly (methyl methacrylate) nanoparticles in rats by coating with surfactants. Int J Pharm. 61:85–100.
- van Laar T, Van der Geest R, Danhof M. 1999. Future delivery systems for apomorphine in patients with Parkinson's disease. Adv Neurol. 80:535–544.
- Vauthier C, Dubernet C, Fattal E, Pinto-Alphandary H, Couvreur P. 2003. Poly (alkylcyanoacrylates) as biodegradable materials for biomedical applications. Adv Drug Deliv Rev. 55:519–548.
- Wagner S, Zensi A, Wien SL, Tschickardt SE, Maier W, Vogel T, et al. 2012. Uptake mechanism of ApoE-modified nanoparticles on brain capillary endothelial cells as a blood-brain barrier model. PloS One. 7:e32568.
- Wen Z, Yan Z, Hu K, Pang Z, Cheng X, Guo L, et al. 2011. Odorranalectin-conjugated nanoparticles: preparation, brain delivery and pharmacodynamic study on Parkinson's disease following intranasal administration. J Control Release. 151:131–138.
- Wu H, Li J, Zhang Q, Yan X, Guo L, Gao X, et al. 2012. A novel small Odorranalectin-bearing cubosomes: Preparation, brain delivery and pharmacodynamic study on amyloid-β25–35-treated rats following intranasal administration. Eur J Pharm Biopharm. 80:368–378.
- Xin H, Sha X, Jiang X, Chen L, Law K, Gu J, et al. 2012. The brain targeting mechanism of Angiopep-conjugated poly (ethylene glycol)-co-poly (ɛ-caprolactone) nanoparticles. Biomaterials. 33: 1673–1681.
- Zensi A, Begley D, Pontikis C, Legros C, Mihoreanu L, Wagner S, et al. 2009. Albumin nanoparticles targeted with Apo E enter the CNS by transcytosis and are delivered to neurones. J Control Release. 137:78–86.
- Zhang C, Wan X, Zheng X, Shao X, Liu Q, Zhang Q, Qian Y. 2014. Dual-functional nanoparticles targeting amyloid plaques in the brains of Alzheimer's disease mice. Biomaterials. 35:456–465.
- Zhang Y, Zhang Q, Zha L, Yang W, Wang C, Jiang X, Fu S. 2004. Preparation, characterization and application of pyrene-loaded methoxy poly (ethylene glycol)–poly (lactic acid) copolymer nanoparticles. Colloid Polym Sci. 282:1323–1328.
- Zhou J. 2010. Recent progress in neurodegenerative disorder research in China. Sci China Life Sci. 53:348–355.
- Zhou J, Fa H, Yin W, Zhang J, Hou C, Huo D, et al. 2014. Synthesis of superparamagnetic iron oxide nanoparticles coated with a DDNP-carboxyl derivative for in vitro magnetic resonance imaging of Alzheimer's disease. Mater Sci Eng C Mater Biol Appl. 37: 348–355.