Abstract
We report a novel method to simultaneously extract superoxide dismutase (SOD), catalase (CAT), and carbonic anhydrase (CA) from the same sample of red blood cells (RBCs). This avoids the need to use expensive commercial enzymes, thus enabling a cost-effective process for large-scale production of a nanobiotechnological polyHb–SOD–CAT–CA complex, with enhancement of all three red blood cell functions. An optimal concentration of phosphate buffer for ethanol–chloroform treatment results in good recovery of CAT, SOD, and CA after extraction. Different concentrations of the enzymes can be used to enhance the activity of polyHb–SOD–CAT–CA to 2, 4, or 6 times that of RBC.
Introduction
Polyhemoglobin (polyHb) is a first-generation blood substitute prepared by the basic method of crosslinking the hemoglobin molecules with glutaraldehyde (CitationChang 1971). This basic principle was later developed independently by two U.S. groups and tested in clinical trials (CitationJahr et al. 2008, CitationMoore et al. 2009, CitationKim and Greenburg 2014). Bovine polyHb has been approved for use in patients in South Africa and Russia (CitationKim and Greenburg 2014). U.S. clinical trials on ambulance hemorrhagic shock patients have shown that human polyHb can be given on the spot without blood typing, and can delay the need for blood transfusion by 12 hours, while the saline control group requires blood transfusion within 1 hour (CitationMoore et al. 2009). The percentage of non-fatal cardiac side-effects was 3%, compared with 0.6% in the control group. The investigators propose that it has potential for use in treating fatal hemorrhagic shock when donor blood is not available (CitationMoore et al. 2009).
The normal red blood cell (RBC) has three main functions: delivery of oxygen to tissues and cells; removal of oxygen radicals; and transport of carbon dioxide from tissues to the lungs (CitationChang 2007). Ischemia/reperfusion injuries may occur in severe hemorrhagic shock, due to the production of oxygen radicals during reperfusion with transfusion (CitationD’Agnillo and Chang 1998, CitationAlayash 2004). The original blood substitutes were prepared from a RBC-stroma-free hemolysate (SFHb) that contains all the red blood cell enzymes, namely superoxide dismutase (SOD), catalase (CAT), and carbonic anhydrase (CA) (CitationChang 1964, Citation1971). In order to enhance the antioxidant enzyme activities, we have added higher concentrations of the enzymes SOD and CAT, to prepare PolyHb–SOD–CAT (CitationD’Agnillo and Chang 1998). This has prevented ischemia/reperfusion in a murine model of hemorrhagic shock stroke (CitationPowanda and Chang 2002). In hemorrhagic shock, the mortality and the severity of myocardial ischemia are related to the elevated intracellular pCO2 (CitationSims et al. 2001). The increase of intracellular carbon dioxide level may lead to acidosis, malfunction of the central nervous system, and even death (CitationGeers and Gros 2000). The RBC enzyme CA is the most important component for the transport of CO2 (CitationGeers and Gros 2000, CitationArthurs and Sudhakar 2005). This fact led this laboratory to prepare an even more up-to-date nanobiotechnological complex of polySFHb–SOD–CAT–CA, with higher concentrations of all three enzymes than those normally present in RBCs (CitationBian et al. 2012, CitationBian and Chang 2015). This polySFHb–SOD–CAT–CA complex with enhanced RBC enzyme activities has been tested in a murine model of 90-min hemorrhagic shock with loss of 2/3rds of blood volume. The result shows that it is more effective than whole blood in lowering the elevated intracellular pCO2, preventing cardiac side-effects, and preventing ischemia/reperfusion injuries in the liver, intestine, and heart (CitationBian and Chang 2015).
Stroma-free hemolysate (SFHb) consists of the RBC content with the membrane removed. This contains hemoglobin and RBC enzymes including CAT, SOD, and CA. SFHb containing these enzymes has been used to prepare the earlier blood substitutes (CitationChang 1964, Citation1971). However, even RBCs with normal CAT, SOD, and CA enzyme activities are not sufficient to prevent irreversible shock in serious sustained hemorrhagic shock (CitationKim and Greenburg 2014, CitationBian and Chang 2015). We therefore successfully prepared the polySFHb–SOD–CAT–CA complex by crosslinking SFHb with additional CAT, SOD, and CA (CitationBian, Rong and Chang 2012, CitationBian and Chang 2015). However, commercial CAT, SOD, and CA are too expensive to scale-up production towards potential clinical use. CitationGu and Chang (2009) have extracted SOD and CAT from SFHb. Ethanol–chloroform was used to denature the hemoglobin, followed by acetone precipitation to purify and precipitate the SOD. CA can also be extracted by the method of ethanol–chloroform treatment and acetone precipitation (Citationda Costa Ores et al. 2012). However, this method results in the inactivation of CAT. Thus CAT had to be precipitated separately by adding ammonium sulfate (CitationGu and Chang 2009). The effect of buffer on the stability of proteins has been studied by CitationUgwu and Apte (2004). They have shown that a phosphate buffer at a pH of 7.4 promotes the conformational stability of enzymes. Therefore, we use potassium phosphate buffer (PPB) to protect CAT during ethanol–chloroform treatment in the present study. Extracting individual enzymes from different batches of SFHb is neither efficient nor cost effective. In the present study, we have designed an optimal method for extracting all three enzymes simultaneously from the same sample of SFHb.
In this study, we have successfully established the method to extract CAT, SOD, and CA from blood and crosslink them with SFHb to prepare PolySFHb–SOD–CAT–CA. The use of different concentrations of PPB for the extraction was analyzed in detail. As a result, we have been able to establish the optimal concentration of PPB for the extraction of CAT together with SOD and CA. When adding 3 M PPB to SFHb, CAT activity can be retained in the Hb-free supernatant after ethanol–chloroform treatment; SOD and CA can also be obtained simultaneously. Finally, the extracted enzymes would be purified and concentrated, followed by crosslinking them with SFHb to generate polySFHb–SOD–CAT–CA. Our results show that our modified ethanol–chloroform treatment can maintain CAT, SOD, and CA activities with high recovery yields. The method we have established is expected to cut down the costs of scale-up production compared with the costs incurred using commercial enzymes.
Materials and methods
Materials
Stroma-free hemoglobin (SFHb) was prepared from bovine blood purchased from the McDonald Campus Cattle Complex at McGill University (Sainte-Anne-de-Bellevue, Canada). Both the Amicon Ultra-15 Centrifugal Filter Unit with Ultracel-10 membrane, and the Biomax polyethersulfone ultrafiltration disc with 300 kDa cut-off were purchased from Millipore. All other experimental and analytical reagents were purchased from Sigma.
Preparation of SFHb
The method was based on that of CitationChang (2007). Briefly, fresh heparinized bovine blood was centrifuged at 4,500 g for 30 min at 4°C. The plasma supernatant containing white blood cells and platelets was aspirated carefully on ice, leaving the red blood cells (RBCs). The RBCs were washed with 0.9 M sodium chloride three times. They were then lysed by adding 2 times the volume of hypotonic sodium phosphate buffer (12.5 mM, pH 7.4) to 1 volume of RBCs. The solution was mixed by repeated inversion and swirling, then left standing for 30 min at 4°C. A volume of 0.5 ml of cold toluene was added to the mixture. After shaking vigorously, the supernatant was allowed to stand for 3 hours at 4°C. The upper layer of the mixture, which contained toluene, stromal lipid, and cellular debris was removed by aspiration. The toluene step was repeated one more time, and the remaining solution was centrifuged at 16 000 g for 2 hours at 4°C. The supernatant was then filtrated by a filter paper. Toluene was removed by vacuum. The stroma-free hemoglobin solution was mixed by stirring, and distributed into 15 ml centrifugal tubes and stored at − 80°C.
Quantitative determination of Hb concentration
The concentration of Hb was colorimetrically determined by allowing the samples to react with Drabkin's reagent (Sigma-Aldrich). After 12 μl of sample was added to 3 ml of Drabkin's reagent in a cuvette, the solution was allowed to stand for 15 min at room temperature, avoiding light. The concentration of cyanmethemoglobin in the solution was measured by spectrophotometry at 540 nm.
Determination of CAT activity
The established method has been described in the study paper by Zhu and Chang [21]. The SFHb and polySFHb samples were diluted at a ratio of 1:200 with PPB (50 mM, pH 7.0) to a total volume of 5 ml; while the SFHb mixed with extracted enzymes and polySFHb–SOD–CAT–CA samples was diluted at a ratio of 1:1000. The H2O2 solution was prepared by adding 40.6 μl of 30% H2O2 solution to 9 ml of PPB (50 mM, pH 7.0). A UV spectrophotometer at 240 nm was used to measure the rate of elimination of H2O2 within 15 seconds. For the blank, 1 ml of diluted sample and 0.5 ml of buffer were mixed. Then, 1 ml of diluted sample mixed with 0.5 ml of H2O2 solution was applied, to determine the CAT activity in the sample.
Determination of SOD activity
The SOD activity assay measures the decrease of cytochrome c by superoxide, observed using a UV spectrophotometer at 550 nm, within 50 seconds [11]. The SFHb and polySFHb samples were diluted 20-fold with PPB (0.1 M, pH 7.4), while the SFHb mixed with extracted enzymes and polySFHb–SOD–CAT–CA samples were diluted 100-fold. The reaction buffer prepared consists of potassium phosphate (50 mM, pH 7.8), EDTA (10–4 M), xanthine (5 × 10–5 M), cytochrome c (10–5 M), and catalase (500 U/ml). Xanthine oxidase was diluted with 0.154 M NaCl to 6 U/ml. The mixture of 1.45 ml of buffer and 25 μl of diluted sample or buffer was applied as blank, and 25 μl of xanthine oxidase (6 U/ml) was then added to initiate the reaction.
Determination of CA activity
The procedure for CA measurement was based on the method established by Bian in 2012 [17]. Tris buffer (20 mM, pH 8.3) and diluted samples were prepared, as above. The substrate of the reaction was prepared by bubbling CO2 through distilled water. Then, 10 μl of diluted sample or the same amount of buffer as control was added into 3 ml of Tris buffer, while monitoring pH changes. To start the test, 2 ml of saturated CO2 solution was added. The time needed for the pH of the mixture to drop from 8.3 to 6.3 was recorded.
Molecular weight distribution
A Sephacryl-300 HR column at a flow rate of 130 ml/hour was used for the analysis of molecular weight distribution. The column was equilibrated with 0.9% NaCl buffer. After 200 μl of sample with Hb at a concentration of 9 g/dL was loaded into the column, 0.9% NaCl buffer was used to elute the sample. The molecular weight distribution was recorded by a 280 nm UV detector at a velocity of 5 mm/min.
Results
Effects of Potassium Phosphate Buffer Concentrations on Enzyme Extraction
For the study, we followed the procedure of enzyme extraction from hemolysate, as described in the next section, except that we used different concentrations of PPB (pH 7.4):
SFHb 3ml + 4M PPB 0.6ml + ethanol–chloroform 1.2ml
SFHb 3ml + 3M PPB 0.6ml + ethanol–chloroform 1.2ml
SFHb 3ml + 2M PPB 0.6ml + ethanol–chloroform 1.2ml
SFHb 3ml + 1M PPB 0.6ml + ethanol–chloroform 1.2ml
SFHb 3ml + 0.1M PPB 0.6ml + ethanol–chloroform 1.2ml
SFHb 3ml + 0.05M PPB 0.6ml + ethanol–chloroform 1.2ml
SFHb 3ml + water 0.6ml + ethanol–chloroform 1.2ml
SFHb 3ml + ethanol–chloroform 1ml
summarizes the results obtained. The enzyme activity in the SFHb was considered as 100%. For CAT, there was little or no enzyme recovered at the lower phosphate buffer concentrations. Increase in the concentration of phosphate ions resulted in increased recovery of CAT, with the highest yield at 3M PPB. The reasons for the more than 100% apparent recovery of CAT activity will be explained in the Discussion. The recovery yields of SOD and CA were both around 70–100%, compared with the activity recovered in SFHb. The concentration of phosphate buffer had no effect on the recovery yields of SOD and CA.
Table I. Recovery yield of apparent activities of CAT, SOD, and CA after extraction using different concentrations of phosphate ions (see Discussion, regarding the possible reasons for the higher recovery of CAT activity).
Based on these results, we used 3M for the final procedure of enzyme extraction, as described in the next section.
Final method established for the extraction and concentration of enzymes from stroma-free hemolysate
A volume of 20 ml of SFHb is added to a beaker on ice, followed by 4 ml of 3M PPB (pH 7.4), to obtain a final concentration of 9 g/dL SFHb. The mixture is then stirred using a magnetic stirrer at medium speed for 5 mins, on ice. Next, 8 ml of cold ethanol–chloroform solution is added dropwise at a speed of 1 drop/sec, and then stirred for 30 min at medium speed on ice. The solution is centrifuged for10mins at 5000 rpm at 4°C. The supernatant layer (usually around 20 ml) is collected, which contains the recovered SOD, CA, and CAT, with little or no Hb. The supernatant is transferred to a new centrifugal tube, and centrifuged at 5000 rpm for 10 mins at 4°C to remove any remaining precipitated Hb. Next, 12 ml of the supernatant is transferred to the Amicon Ultra-15 Centrifugal 10 kDa filter unit and centrifuged for 10 min at 6000 rpm at 4°C. The remaining 8–10 ml of supernatant is then added to the same filter unit containing the enzyme solution, and centrifuged at 6000 rpm for 15 mins at 4°C.The ethanol remaining in the extracted enzyme solution is washed out by adding 5 ml of 0.2M Na·PO4 buffer (pH 7.4) to the centrifugal unit, mixing the solution, and then centrifuging at 6000 rpm at 4°C for 15 min. The washing steps are repeated two more times. Finally, to minimize the final volume of concentrated enzymes, the enzyme solution is washed with 2 ml of Na·PO4 buffer (pH 7.4) and centrifuged at 6000 rpm at 4°C for 15 mins. The final volume of concentrated enzymes should be approximately 300 μl.
Final method established for the preparation of PolySFHb–SOD–CAT–CA using extracted enzymes
A volume of 4 ml of 9 g/dl SFHb is added to different amounts of the concentrated enzymes, prepared as described above. Then 102 μl of 4M NaCl is added to the solution and shaken at 160 rpm at 4°C for 5 mins. Frozen glutaraldehyde (0.5M) is thawed on an ice-water mixture using ultrasonication for 30–40 mins. The glutaraldehyde must be mixed evenly before use. Without stopping the shaker, 162.3 μl of glutaraldehyde is added dropwise in 4 equal aliquots at intervals of 15 mins, and shaken at 160 rpm for 24 hrs at 4°C. Next, 641.4 μl of 2M lysine (200:1 lysine: protein molar ratio) is added in 2 equal aliquots over 10 mins and shaken at 160 rpm at 4°C for 1 hour, to quench. The solution is centrifuged at 8000 rpm at 4°C for 1 hour to remove any precipitate.
The polySFHb was prepared following the same procedure as for the preparation of polySFHb–SOD–CAT–CA, but without adding the extracted enzymes. The supernatant of the crosslinked sample was around 4–5 ml. The sample was purified using a 300 KDa ultrafiltration disc with positive air pressure. After purification, the Hb concentrations of the crosslinked samples were tested and adjusted to 9g/dL with 0.1M PPB (pH 7.4).
Molecular Weight Distribution
The molecular weight distributions of polySFHb–SOD–CAT–CA, polySFHb, and SFHb were analyzed with Sephacryl S-300 gel column chromatography. A volume of 200 μl of supernatant was loaded on the column. In the polymerized products polySFHb–SOD–CAT–CA or polySFHb, most of the molecules were in the > 669 KDa range (). For SFHb, most of the molecules were in the 64KD hemoglobin range ().
Determination of Enzyme Activities and Recovery of Yield
The CAT, SOD, and CA activities were measured for the following samples before and after crosslinking: SFHb, and SFHb with concentrated enzymes added at 2x, 4x and 6x the original SFHb enzyme concentrations. After purification of the polymerized molecules with the 300 KDa ultrafiltration disc, the enzyme activities of the complexes were measured ().
Figure 2. Comparison of CAT activities in different samples. Left to right: SFHb before and after crosslinking; SFHb + CAT+ SOD + CA 2x (SFHb plus 2x extracted SOD, CAT, and CA) before and after crosslinking; SFHb + CAT+ SOD + CA 4x (SFHb plus 4x extracted SOD, CAT, and CA) before and after crosslinking; SFHb + CAT+ SOD + CA 6x (SFHb plus 6x extracted SOD, CAT, and CA) before and after crosslinking.
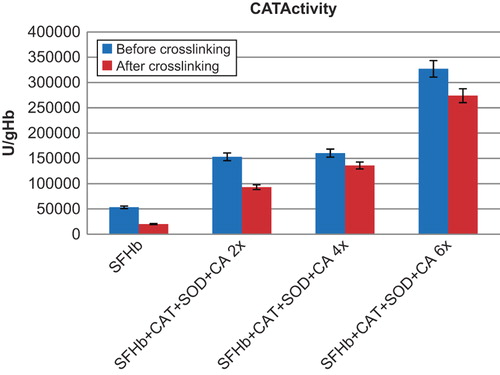
Figure 3. Comparison of SOD activities in different samples. Left to right: SFHb before and after crosslinking; SFHb+ CAT+ SOD+ CA 2x (SFHb plus 2x extracted SOD, CAT, and CA) before and after crosslinking; SFHb+ CAT+ SOD+ CA 4x (SFHb plus 4x extracted SOD, CAT, and CA) before and after crosslinking; SFHb+ CAT+ SOD+ CA 6x (SFHb plus 6x extracted SOD, CAT, and CA) before and after crosslinking.
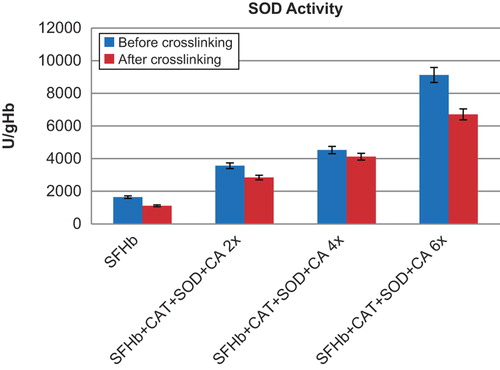
Figure 4. Comparison of CA activities in different samples. Left to right: SFHb before and after crosslinking; SFHb+ CAT+ SOD+ CA 2x (SFHb plus 2x extracted SOD, CAT, and CA) before and after crosslinking; SFHb+ CAT+ SOD+ CA 4x (SFHb plus 4x extracted SOD, CAT, and CA) before and after crosslinking; SFHb+ CAT+ SOD+ CA 6x (SFHb plus 6x extracted SOD, CAT, and CA) before and after crosslinking.
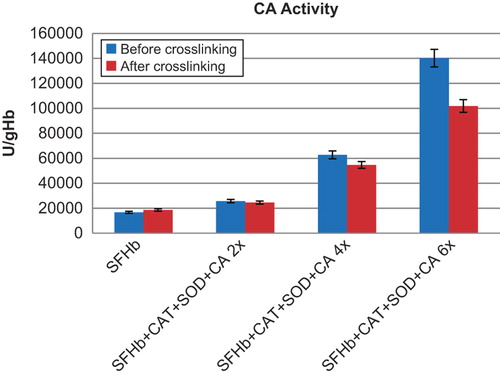
CAT activity in SFHb was found to be 53234 ± 2945 U/g Hb. After crosslinking into polySFHb, the catalase activity was 20124 ± 1053 U/g Hb). SFHb plus different amounts of concentrated extracted enzymes before crosslinking and after crosslinking also showed good recovery of CAT activities (). For example, the CAT activity for SFHb with 2x extracted SOD, CAT, and CA was 153046 ± 2207 U/g Hb before crosslinking, and 93034 ± 3537 U/g Hb after crosslinking. With 4x extracted SOD, CAT, and CA, the activity was 160320 ± 2205 U/g Hb) before crosslinking, and 135909 ± 3918 U/g Hb after crosslinking. With 6x extracted SOD, CAT, and CA, the activity was 326989 ± 7385 U/g Hb) before crosslinking, and 273919 ± 7379 U/g Hb after crosslinking.
SOD activity in SFHb was found to be 1642 ± 48 U/ml. After crosslinking into polySFHb, the SOD activity was 1114 ± 33 U/ml (). SFHb plus different amounts of concentrated extracted enzymes before crosslinking and after crosslinking also showed good recovery of SOD activities (). For example, the SOD activity for SFHb with 2x extracted SOD, CAT, and CA was 3567 ± 100 U/g Hb) before crosslinking, and 2846 ± 68 U/g Hb after crosslinking. For 4x extracted SOD, CAT and CA, the activity was 4526 ± 65 U/g Hb) before crosslinking and 4149 ± 69 U/g Hb after crosslinking. For 6x extracted SOD, CAT, and CA, the activity was 9124 ± 338 U/ml before crosslinking and 6712 ± 181 U/ml after crosslinking.
CA activity in SFHb was 16725 ± 411 U/g Hb. After crosslinking into polySFHb, the CA activity was 18638 ± 711 U/g Hb (). SFHb plus different amounts of concentrated extracted enzymes before crosslinking and after crosslinking also showed good recovery of CA activities (). For example, the CA activity for SFHb with 2x extracted SOD, CAT, and CA was 25722 ± 758 U/g Hb) before crosslinking and 24547 ± 574 U/g Hb after crosslinking. For 4x extracted SOD, CAT, and CA, the activity was 62776 ± 680 U/g Hb before crosslinking and 54707 ± 400 U/g Hb after crosslinking. For 6x extracted SOD, CAT, and CA, the activity was 140243 ± 2911 U/g Hb before crosslinking and 101869 ± 4248 U/g Hb after crosslinking.
Discussion and conclusion
PPB at a pH of 7.4 can prevent CAT from aggregation after ethanol–chloroform treatment. It is likely that the ion strength and pH value of the buffer contribute to the aggregation of hemoglobin and the stability of the three enzymes. The molecular weights (MW) of hemoglobin, CAT, SOD, and CA are 68 KDa, 250 KDa, 32.5 KDa, and 29 KDa respectively. The isoelectric points (pI) of hemoglobin, CAT, SOD, and CA are 6.8, 5.4, 4.95, and 5.9 respectively. As we can see, only the pI of hemoglobin is close to the pH of the solution at 7.4; thus hemoglobin tends to aggregate when adding organic solvents that have reduced dielectric strength (CitationCrowell et al. 2013). In spite of its low pI value, CAT also aggregates after ethanol–chloroform treatment, possibly due to its large size and the ion pairing effect. However, when increasing the final concentration of phosphate ions from 20.3 mM to 257.8 mM, the improvement in CAT recovery yield can be observed (). The phosphate buffer may be able to protect CAT because a large amount of ions migrate to the charged surface residues to generate a solvent layer, preventing the aggregation of CAT; the pI of hemoglobin is close to the pH of solution, therefore hemoglobin cannot attract enough ions to form a hydration shield. Interestingly, the phosphate buffer was reported to destabilize the hemoglobin by binding to the 2,3-bisphosphoglycerate (2,3-BPG) site (CitationUgwu and Apte 2004).Therefore, the phosphate buffer at a pH of 7.4 can protect CAT and simultaneously promote hemoglobin precipitation.
Why is it that compared with the original enzyme activities assayed in SFHb, the apparent CAT activities increase in the extracted enzyme solution after ethanol–chloroform treatment? There are a number of possible explanations. Firstly, hemoglobin has peroxidase-like activity (CitationPaco et al. 2009). We have done the following test on the effect of hemoglobin on CAT activity. When CAT is added to the SFHb, the total CAT activity of the mixture is lower than the summation of CAT activities in the extracted enzymes and the SFHb (). Therefore, it is likely that hemoglobin may suppress the CAT as a competitive inhibitor. Secondly, ethanol has been found to increase the CAT activity (CitationMagner and Klibanov 1995). We compared the activity of pure CAT and CAT-ethanol mixture. The ethanol concentration in this test is equal to the concentration during the extraction procedure. The result shows that 12.5% ethanol can increase CAT activity by 1.4 times. Thirdly, hemoglobin can be converted to choleglobin by coupling with ascorbic acid in the presence of oxygen (CitationMiller 1958). Choleglobin formation can even increase when RBCs are hemolyzed (CitationFoulkes and Lemberg 1949). The hemoglobin–ascorbic acid complex is the intermediary substance in choleglobin formation and acts as a hydrogen peroxide donor (CitationMiller 1958). The assay of CAT is based on the measurement of H2O2 left after the reaction of samples with H2O2 solution.
In conclusion, we have devised a novel method to simultaneously extract all three enzymes, CAT, SOD, and CA, from RBC hemolysate (SFHb). When using bovine RBC, the unlimited and inexpensive source of bovine blood would be much less costly than commercial enzymes to scale-up the production of blood substitute. Earlier studies from this laboratory have shown that bovine PolySFHb containing the bovine RBC enzymes did not show immunological reactions when tested in rats (CitationZhu et al. 2010). We shall investigate the effects of using higher concentrations of enzymes. If there is an immunological problem, we can also use our novel method for the extraction of human hemoglobin and enzymes from human placental RBCs (cord RBCs) for the preparation of polySFHb–SOD–CAT–CA.
Acknowledgements
This laboratory is not connected with any commercial organizations. The research support for the study reported here comes from the joint program of the Canadian Blood Service/Canadian Institutes of Health Research that requires the authors to state that: “The opnions expressed in this article are the opnions of the investigators of this paper and not neccssarily those of the Canadian Government nor of the Canada Blood Service”.
Declaration of interest
The authors report no declarations of interest. The authors alone are responsible for the content and writing of the paper.
References
- Alayash AI. 2004. Redox biology of blood. Antioxid Redox Signal. 6:941–943.
- Arthurs G, Sudhakar M. 2005. Carbon dioxide transport. Continuing Education in Anaesthesia. Critical Care & Pain. 5:207–210.
- Bian Y, Rong Z, Chang TM. 2012. Polyhemoglobin-superoxide dismutase-catalase-carbonic anhydrase: a novel biotechnology-based blood substitute that transports both oxygen and carbon dioxide and also acts as an antioxidant. Artif Cells Blood Substit Immobil Biotechnol. 40:28–37.
- Bian Y, Chang TMS. 2015. A novel nanobiotherapeutic poly-[hemoglobin-superoxide dismutase-catalase-carbonic anhydrase] with no cardiac toxicity for the resuscitation of a rat model with 90 minutes of sustained severe hemorrhagic shock with loss of 2/3 blood volume. Artif Cells Nanomed Biotechnol. 43:1–9.
- Chang TM. 1964. Semipermeable microcapsules. Science. 146:524–525.
- Chang TM. 1971. Stabilisation of enzymes by microencapsulation with a concentrated protein solution or by microencapsulation followed by cross-linking with glutaraldehyde. Biochem Biophys Res Commun. 44:1531–1536.
- Chang TM. 2007. Artificial cells: Biotechnology, nanotechnology, blood substitutes, regenerative medicine, bioencapsulation, cell/stem cell therapy. World Scientific Publisher/Imperial College Press, p. 455. Available at http://www.medicine.mcgill.ca/artcell/2007%20ebook%20artcell%20web.pdf. Accessed on April 2 2015.
- Chang TM. 2012. From artificial red blood cells, oxygen carriers, and oxygen therapeutics to artificial cells, nanomedicine, and beyond. Artif Cells Blood Substit Immobil Biotechnol. 40:197–199.
- Chang TM. 2013. Selected topics in nanomedicine. In: Chang TMS, Ed. Artificial cells that started nanomedicine. World Science Publisher/Imperial College Press, pp. 1–21.
- Crowell AM, Wall MJ, Doucette AA. 2013. Maximizing recovery of water-soluble proteins through acetone precipitation. Anal Chim Acta. 796:48–54.
- da Costa Ores J, Sala L, Cerveira GP, Kalil SJ. 2012. Purification of carbonic anhydrase from bovine erythrocytes and its application in the enzymic capture of carbon dioxide. Chemosphere. 88:255–259.
- D’Agnillo F, Chang TM. 1998. Polyhemoglobin-superoxide dismutase-catalase as a blood substitute with antioxidant properties. Nat Biotechnol. 16:667–671.
- Foulkes E, Lemberg R. 1949. The formation of choleglobin and the role of catalase in the erythrocyte. Proceedings of the Royal Society of London. Series B-Biological Sciences. 136:435–448.
- Geers C, Gros G. 2000. Carbon dioxide transport and carbonic anhydrase in blood and muscle. Physiol Rev. 80:681–715.
- Gu J, Chang TM. 2009. Extraction of erythrocyte enzymes for the preparation of polyhemoglobin-catalase-superoxide dismutase. Artif Cells Blood Substit Immobil Biotechnol. 37:69–77.
- Jahr JS, Mackenzie C, Pearce LB, Pitman A, Greenburg AG. 2008. HBOC-201 as an alternative to blood transfusion: efficacy and safety evaluation in a multicenter phase III trial in elective orthopedic surgery. J Trauma. 64:1484–1497.
- Kim HW, Greenburg AG (Eds.). 2014. Hemoglobin-based oxygen carriers as red cell substitutes and oxygen therapeutics. Springer.
- Magner E, Klibanov AM. 1995. The oxidation of chiral alcohols catalyzed by catalase in organic solvents. Biotechnology Bioeng. 46:175–179.
- Miller H. 1958. The relationship between catalase and haemoglobin in human blood. Biochem J. 68:275–282.
- Moore EE, Moore FA, Fabian TC, Bernard AC, Fulda GJ, Hoyt DB. 2009. Human polymerized hemoglobin for the treatment of hemorrhagic shock when blood is unavailable: the USA multicenter trial. J Am Coll Surg. 208:1–13.
- Paco L, Galarneau A, Drone J, Fajula F, Bailly C, Pulvin S, Thomas D. 2009. Catalase‐like activity of bovine met‐hemoglobin: interaction with the pseudo‐catalytic peroxidation of anthracene traces in aqueous medium. Biotechnol J. 4:1460–1470.
- Powanda DD, Chang TM. 2002. Cross-linked polyhemoglobin-superoxide dismutase-catalase supplies oxygen without causing blood-brain barrier disruption or brain edema in a rat model of transient global brain ischemia-reperfusion. Artif Cells Blood Substit Immobil Biotechnol. 30:23–37.
- Sims C, Seigne P, Menconi M, Monarca J, Barlow C, Pettit J, Puyana JC. 2001. Skeletal muscle acidosis correlates with the severity of blood volume loss during shock and resuscitation. J Trauma. 51:1137–1145; discussion 1145–1146.
- Ugwu SO, Apte SP. 2004. The effect of buffers on protein conformational stability. Pharm Technol. 28:86–109.
- Zhu H, Du Q, Chen C, Chang TM. 2010. The immunological properties of stroma-free polyhemolysate containing catalase and superoxide dismutase activities prepared by polymerized bovine stroma-free hemolysate. Artif Cells Blood Substit Immobil Biotechnol. 38:57–63.