Abstract
Glutaraldehyde-polymerized porcine hemoglobin (pPolyHb) is a hemoglobin-based oxygen carrier currently being developed as a potential red blood cell substitute. We assessed the protective effect of pPolyHb on transient focal cerebral ischemia/reperfusion (I/R) injury. Several outcomes were tested, including infarct size, neurological score, production of inflammatory factors, and markers of oxidative status. Our results show that pPolyHb can not only significantly reduce infarct volume and improve neurological score, but can also inhibit the expression of TNF-α and IL-1β. Furthermore, the generation of MDA and MPO was reduced by pPolyHb. We conclude that pPolyHb has a positive effect on transient focal cerebral I/R injury.
Introduction
Stroke is a common refractory disease that threatens human health and safety. Currently, because of high morbidity and high mortality, stroke is a major public health challenge in both developing and developed countries (CitationRoger et al. 2011). Stroke includes both hemorrhagic stroke and ischemic stroke, with the latter being more common and thus garnering more scholarly attention in finding effective therapies (CitationZhou et al. 2008). Cerebral ischemia is a consequence of a transient or permanent reduction in cerebral blood flow that is restricted to the territory of a major brain artery (CitationMahajan et al. 2004). Researchers have found that cerebral ischemia–reperfusion (I/R) injury is even more serious than cerebral ischemic injury (CitationTsubota et al. 2010). The mechanisms underlying I/R injury have been explored, and include generation of oxygen free radicals, elicitation of an inflammatory response, activation of apoptosis factors, and other toxic processes. The effect of oxygen free radicals is not only to cause oxidative damage to blood vessels but also to destroy cell membranes and inhibit the activity of enzymes responsible for cellular respiration. This leads to a series of reactions that trigger the toxic effects of excitatory amino acids on nerve cells, causing a cascade of cerebral I/R injury reaction events and ultimately resulting in neuronal death. Intracellular calcium overloading causes irreversible damage to inflammatory cells, resulting in inflammatory cell infiltration and an inflammatory response mediated by adhesion molecules. Furthermore, activation of apoptosis factors during I/R leads to neuronal apoptosis (CitationCandelario-Jalil 2009, CitationDoyle et al. 2008, CitationRitz et al. 2008, CitationMukerji et al. 2009).
To date, several methods and substances have been found to reduce the effects of I/R injury in animal models, such as ischemic pretreatment (CitationWang et al. 2009, CitationPeng et al. 2012) and isovolumic hemodilution (CitationHofland and Henny 2007). Ischemic pretreatment induces organisms to protect themselves against future injury by adapting to mild ischemia. This mechanism is known as ischemic tolerance and can alleviate I/R injury through a series of molecular regulatory pathways that involve changes in ion channel permeability and post-translational modifications of proteins (CitationZhao et al. 2012). Isovolumic hemodilution can attenuate I/R injury mainly by reducing blood viscosity and increasing regional blood flow, which provides more oxygen to tissues.
Hydroxyethyl starch (HES), a kind of blood volume expander, is also used to alleviate I/R injury. HES acts by reducing blood viscosity, the systemic inflammatory response, and endothelial cell dysfunction (CitationRittoo et al. 2005, CitationPinar et al. 2015). Hemoglobin-based oxygen carriers (HBOCs) have been found to play a role in attenuating I/R injury. HBOCs allow the transport of more oxygen (O2) to hypoxic tissues owing to their lower viscosity, higher O2 affinity, and smaller mean diameter (CitationLi et al. 2006, CitationStandl et al. 2003), suggesting that HBOCs may be beneficial in providing sufficient microcirculation perfusion to alleviate I/R injury (CitationCaswell et al. 2005, CitationWu et al. 2009, CitationMoore et al. 2005). Sometimes, HBOCs could achieve better effect. For instance, a soluble polyHb-SOD-CAT-CA complex, which can remove damaging oxygen radicals by adding antioxidative enzyme may mitigate I/R injury (CitationBian and Chang 2015). Good progress has been made in demonstrating the potential for certain HBOCs to be used in cerebral I/R injury. For example, hemoglobin vesicles (HbV) were shown to improve neurological scores and reduce infarct size (CitationShimbo et al. 2014). Moreover, the cross-linked PolyHb-SOD-CAT solution can supply oxygen to ischemic tissues without causing reperfusion injury in the transient global brain I/R model (CitationPowanda and Chang 2002). Glutaraldehyde-polymerized porcine hemoglobin (pPolyHb) is an HBOC based on porcine hemoglobin that has been proven safe and effective in an exchange transfusion model (CitationZhu et al. 2011a, CitationZhu et al. 2011b). In this study, we explore whether pPolyHb has an effect on focal transient cerebral I/R injury in a rat model, as measured by cerebral infarct size, neurological scores, inflammatory response, and oxygen free radical injury.
Materials
Reagents
6% Hetastarch 200/0.5 in sodium chloride solution (HES) (Fresenius Kabi), Trichloroacetaldehyde Hydrate (Sigma), Hepalean 1000 U.S.P. units/ml (Organon), Sodium Chloride (Sigma), Sodium Phosphate Monobasic (Sigma), Disodium Hydrogen Phosphate (Sigma), Phosphate-buffered saline (PBS) (Sigma), Paraformaldehyde (Sigma), Triphenyl Tetrazolium Chloride (Sigma), Phenylmethanesulfonyl Fluoride (PMSF, Beyotime), RIPA Lysis buffer (Beyotime), Rat TNF-α ELISA kit, Rat IL-1β ELISA kit, Rat IL-10 ELISA kit (DAKEWE), Lipid Peroxidation (MDA) Assay Kit (Beyotime), Myeloperoxidase (MPO) assay kit (Njjcbio).
Animal
Adult male Sprague-Dawley rats (provided by the animal laboratory center of the Fourth Military Medical University) weighing 280 ± 20 g were used in the study. All experimental procedures were carried out according to protocols approved by the Ethics Committee for Animal Experimentation of the Fourth Military Medical University (Xi’an, Shaanxi, China) and in accordance with the National Institutes of Health Guide for the Care and Use of Laboratory Animals.
Test Solutions
pPolyHb (9.7 ± 0.5 g/dl polymerized porcine hemoglobin, methemoglobin < 5%, endotoxin < 1.0EU/mL, osmolality 300–330 mOsm, pH 7.4 ± 0.05, average molecular weight of pPolyHb 600 ± 50 kD, 64kD tetramer < 2%) was formulated in buffer consisting of Na+ 135–155 mmol/L, K+ 3.0–5.0 mmol/L, Ca2+ 1–3 mmol/L, and Cl− 140–160 mmol/L and stored at 4°C under nitrogen gas until use.
Methods
Surgical preparation
Male Sprague-Dawley rats were food-deprived for approximately 12 h before surgery. Transient focal cerebral ischemia was induced by right middle cerebral artery occlusion (MCAO) using a modified intraluminal filament technique (CitationKoizumi et al. 1986).
MCAO model
Sprague-Dawley rats were anesthetized with trichloroacetaldehyde hydrate (10%, 3 ml/kg, intraperitoneally) and placed in a supine position. Iodine was used to wipe the neck region and clean the instruments. The right common carotid artery (CCA), the right external carotid artery (ECA), and the right internal carotid artery (ICA) were isolated and a 3–0 silk suture was tied at the origin of the ECA and at the proximal end of the CCA. The right ICA was threaded with a silk suture but not tied at the distal end. A 4–0 nylon monofilament (length 23 mm) was introduced into the CCA and pushed up the ICA until light resistance was felt. The distal end of the silk suture was then tied. Depending on the experimental group, HES or pPolyHb was injected immediately via the tail vein. The amount of pPolyHb corresponded to 0.5 g/Kg body weight hemoglobin, and the volume of HES was equal to the volume of pPolyHb. The control group received no injection, and the sham group was operated on but without inserting the monofilament. The skin was sewed up and rats were kept at 37°C to maintain homeothermy. After 90 min, rats were anesthetized using isoflurane, and the monofilament was removed to allow reperfusion. Once the animals recovered from anesthesia, they were scored based on a four-point scale (described by Longa ZE et al.) (CitationLonga et al. 1989). Briefly: 0, no neurological symptoms; 1, unable to completely extend the front contralateral paw; 2, rotating while crawling and falling to the contralateral side; 3, unable to walk without assistance; and 4, unconsciousness (rather than no recovery). Rats with scores of 1∼3 points were considered successful models and were included in the study. Rats were returned to their cages and kept at 37°C after scoring.
Neurological scores
Each rat was subjected to a set of neurological status tests to arrive at a Garcia score, 24 h after I/R injury. The scores were assessed by a person familiar with the evaluation standard and blind to the experimental groups. According to the Garcia standards, several aspects were evaluated to arrive at composite neurological scores, including spontaneous movements of all limbs; climbing the walls of wire cages; reaching out of forelimbs while being held by the tail; reaction to touch on both sides of the trunk; response to vibrissae touch, etc. (CitationGarcia et al. 1995). The score was graded on a scale of 0 to 18, a higher score indicating greater neurological protection.
Infarct volume measurement
After neurological scores were assessed, the rats were sacrificed with a lethal dose of anesthetic. Brains were quickly removed and frozen at − 80°C for 2–5 min. Frozen brains were sliced into uniform sections of 2 mm via a rat brain matrix. All slices were stained by triphenyl tetrazolium chloride solution (2% TTC, dissolved in phosphate-buffered saline) for 20 min, then fixed by immersion in a paraformaldehyde solution (4%) for 24 h. Photographs were taken and Adobe Photoshop software used to calculate infarct volume. Three groups (control (injury), HES treatment, and pPolyHb treatment) of 8 rats each were used for this experiment.
IL-1β, TNF-α and IL-10 measurement
Blood was drawn from each rat at 6 h, 24 h, and 72 h after I/R injury. Plasma was isolated by centrifuging blood at 3000r/min for 10 min, and kept frozen at − 80°C in small aliquots until analysis. IL-1β, TNF-α and IL-10 levels were detected using the Rat TNF-α ELISA, Rat IL-1β ELISA, and Rat IL-10 ELISA kits (DAKEWE), according to the manufacturer's protocols. The four groups used were the sham (normal group), control (injury group), HES, and pPolyHb (drug groups). Assays were performed in triplicate. The detailed method is as described (CitationChang 1997).
MDA and MPO measurement
Careful preparation of the brain tissue homogenate was critical for this experiment. First, ischemic penumbrae separated from the right side of the brains were kept in small aliquots. RIPA lysis buffer (10 μl/mg) and PMSF (0.1 μl/mg) were added according to the weight of the ischemic penumbra. Second, brain tissue protein was extracted by sonication and then kept stationary for 30 min in an ice bath. Third, brain tissue homogenate was isolated by centrifuging at 12 000 r/min for 20 min, then aliquoted and kept frozen at − 80°C. All of the above steps were carried out using an ice bath. Tissue homogenates (0.2 ml, 0.1 ml) were used to detect MPO and malondialdehyde (MDA), respectively. MPO and MDA were measured by a colorimetric method, each according to the manufacturers’ protocols. Assays were performed in triplicate. The time points and groups were similar to those above.
Statistical analysis
Data was represented as means ± SD for replicate experiments. The differences between treatment groups were assessed by one-way ANOVA followed by unpaired Student's t-test. Statistical significance was defined as p < 0.05 to reject a null hypothesis. All statistical calculations were performed with JMP version 3.2 for the Macintosh (SAS Institute, Cary, NC).
Results
Neurological scores
Transient focal cerebral ischemia was induced by MCA occlusion in 3 groups (control, HES, pPolyHb). Neurological status was examined at 24 h after ischemia and reperfusion and scored on an 18-point scale (). Each group included 8 successful model rats. The neurological scores of both the HES group and the pPolyHb group were significantly higher than those of the control group that received no injection. There was no significant difference between scores for the HES group and the pPolyHb group. The main improvements following treatment were in the animals’ movement, balance, and rotation.
Figure 1. The assessment of neurological scores and infarct volume. Neurological scores and infarct volumes were appraised at 24 h after cerebral I/R injury. The Garcia score is a functional test used to assess movement, sensory, reflex, and balance deficits. Sprague-Dawley rats (n = 24) were randomized into three groups: control (injury) group, HES group, and pPolyHb treatment groups. A: Comparison of neurological scores at 24 h after I/R injury in different groups. *P < 0.05 compared with the control group. **P < 0.01 compared with the control group. B: TTC staining pictures of brain slices from the three groups. C: Infarct volumes were determined by TTC staining and Adobe Photoshop analysis. At 24 h after reperfusion, isolated brains were sectioned and stained. *P < 0.05 in comparison to the control group.
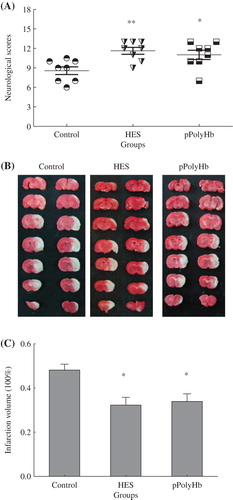
Infarct volume
The infarct volume in ischemic brains induced by MCA occlusion followed by 24 h of reperfusion was measured in the three groups (). Damage to the cerebral cortex was the dominating injury. The boundary between areas of infarct and normal brain were clearly distinguished, with the area of infarct appearing white and normal brain appearing red. Adobe Photoshop CS2 was used to calculate the infract volume percentage, and one-way ANOVA statistical analysis was used to determine if there was a significant difference. The results have been presented in . The control group exhibited the most serious injury (largest infarct volume), whereas both the HES group and the pPolyHb group had significantly lower infarct volumes than the control group (P < 0.05 for both). There was no difference in infarct volume between the HES and pPolyHb groups.
Assessment of inflammatory activity
Inflammation contributes to I/R-related brain injury. Depending on the physiological conditions, inflammatory cytokines elicit diverse responses and activate different pathways. Cytokines can be upregulated in the brain and are expressed not only in cells of the immune system, but also produced by resident brain cells. The most studied cytokines related to inflammation in I/R injury are IL-1β, TNF-α and IL-10. Among those cytokines, IL-1β and TNF-α appear to exacerbate cerebral injury, whereas IL-10 may be neuroprotective (CitationHan and Yenari 2003).
The concentration of IL-1β, TNF-α, and IL-10 in rat blood at various times following reperfusion is presented in . At 24 h after reperfusion, the concentration of IL-1β in the control group was significantly higher than in the sham group (P < 0.05) (). The other two groups (HES and pPolyHb) showed no difference compared with the sham group. At 72 h after reperfusion, the IL-1β concentrations of the four groups were 324 pg/ml, 525 pg/ml, 340 pg/ml, and 394 pg/ml respectively, with the concentrations in the two treatment groups near to those in the sham group at 72 h after reperfusion. A similar tendency was observed for TNF-α (), which reached a peak concentration at 24 h after reperfusion. The TNF-α concentration of the control group rose dramatically between 6 h and 24 h (P < 0.05), and was significantly higher compared to that of the sham group at 24 h (P < 0.05). The concentration of TNF-α in the two treatment groups showed no difference from the sham group at 24 h, and also no difference between them. At 72 h after reperfusion, the TNF-α expression in the control group was the highest (110.2 pg/ml) compared with that in the sham (54 pg/ml), HES (45.45 pg/ml), and pPolyHb (52.2 pg/ml) groups.
Figure 2. Concentrations of inflammatory cytokines following treatment with different agents. Sprague-Dawley rats (n = 36) were randomized into four groups: sham (normal group), control (injury group), HES and pPolyHb (treatment groups), with 9 rats per group. Plasma from Sprague-Dawley rats was obtained at 6 h, 24 h and 72 h after reperfusion. A: Change of IL-1β level in different groups. Assays were performed in triplicate. *P < 0.05 compared with the sham group at 24 h. B: Change of TNF-α level. Assays were performed in triplicate. *P < 0.05 compared with the sham group at 24 h. #P < 0.05 compared with the control group at 6 h. C: Change of IL-10 level. Assays were performed in triplicate.*P < 0.05 compared with the sham group at 24 h. #P < 0.05 compared with sham group at 6 h.
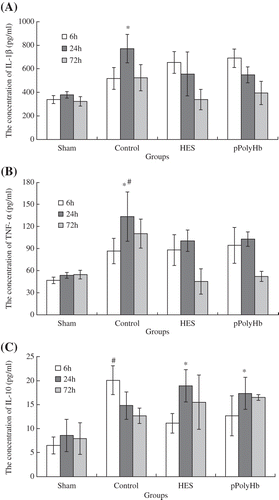
The effect of various conditions on IL-10 levels is presented in . The IL-10 concentration in the control group was the highest at 6 h after reperfusion (P < 0.05 vs sham at 6 h), but was lower at 24 h. In contrast, the concentration of IL-10 in the HES and pPolyHb groups was significantly elevated at 24 h (P < 0.05 vs sham at 24 h). The IL-10 concentration of the four groups were 7.91 pg/ml, 12.6 pg/ml, 15.5 pg/ml, and 16.48 pg/ml respectively, at 72 h after reperfusion.
Assessment of MDA and MPO
MDA, which is one of the products of lipid peroxidation, can be considered to directly reflect the level of tissue oxidation (CitationTu et al. 2013). The concentration of MDA at various time points is presented in . At 24 h after reperfusion, the MDA concentration in the control group was significantly higher than in the sham group (P < 0.05). The HES and pPolyHb groups showed no difference compared to the sham group. The MDA concentrations of the four groups at 24 h were 10.09 μM (sham), 17.98 μM (control), 12.11 μM (HES) and 11.91 μM (pPolyHb), suggesting that pPolyHb could reduce the production of MDA. The MPO level is a reflection of the extent of leukocyte infiltration, and an increase in MPO is correlated with serious damage. The activity of MPO is shown in . At 6 h after reperfusion, the MPO activity of the control group reached its highest level, and was statistically higher than in the sham group (P < 0.05). In contrast, the HES and pPolyHb groups showed no difference compared with the sham group, suggesting that pPolyHb may inhibit the activity of MPO.
Figure 3. The levels of MDA and MPO following the MCAO model. Sprague-Dawley rats (n = 36) were randomized into four groups: sham (normal group), control (injury group), and HES and pPolyHb (treatment groups). Brain tissue homogenates were collected at 6 h, 24 h, and 72 h after reperfusion. MDA and MPO levels were detected according to manufacturers’ protocols. Assays were performed in triplicate. A: MDA concentration in different groups. *P < 0.05 compared with the sham group at 24 h. B: MPO activities in different groups. #P < 0.05 compared with the sham group at 6 h.
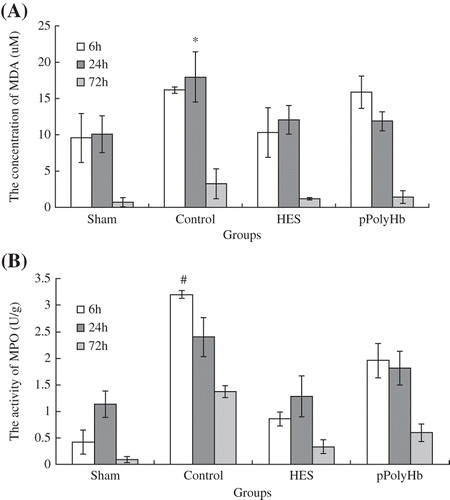
Discussion
pPolyHb is a kind of HBOC that shows promise for the treatment of blood loss. So far, it has been proven safe in blood exchange models (CitationZhu et al. 2011a, CitationZhu et al. 2011b). In this study we wanted to determine whether pPolyHb has any protective effect on transient focal cerebral I/R injury. We assessed the effect of pPolyHb on neurological scores and infarct volume (the fundamental criteria to demonstrate its protective role (CitationSeiffge et al. 2012)) as well as on the release of inflammatory cytokines, including IL-1β, TNF-α, and IL-10.
pPolyHb is able to carry and release oxygen whenever necessary. Moreover, the diameter of pPolyHb is only 20 nm, which is far smaller than that of red blood cells. Therefore, it can transport oxygen through narrowed vessels to ischemic regions and attenuate I/R injury. Our results demonstrate that pPolyHb can improve neurological performance and reduce the volume of cerebral infarction in rats suffering from I/R injury, indicating that pPolyHb has a protective effect on cerebral I/R injury.
Cerebral ischemia and reperfusion injury have been shown to increase lipid peroxidation and aggravate oxidative stress (CitationBriyal et al. 2014). Oxidative damage is particularly dangerous in the microcirculation because excess leakage of plasma components into the interstitium can disturb the fluid balance between blood and tissue and alter endogenous enzymes, which will mediate an inflammatory response. Inflammatory cytokines are important indicators of an inflammatory response. Proinflammatory cytokines facilitate the passage of leukocytes from the circulation into the tissues and initiate the cascade of inflammatory mediators, while anti-inflammatory cytokines block this process or at least suppress the intensity of the cascade. Our previous study demonstrated that pPolyHb significantly inhibits H2O2-mediated endothelial cell damage as well as apoptosis due to its anti-oxidative capacity (CitationZhang et al. 2012). In addition, our current study shows that pPolyHb can reduce MDA production, an indicator of oxidative injury, suggesting that pPolyHb may inhibit the inflammatory response via its attenuation of oxidative stress. Our current results also indicate that pPolyHb blocks the expression of proinflammatory cytokines, such as IL-1β and TNF-α, while promoting the expression of the anti-inflammatory cytokine IL-10. Furthermore, tissue MPO activity was tested to evaluate neutrophil infiltration. The results demonstrate that pPolyHb reduces the production of MPO after cerebral I/R injury. Thus, pPolyHb appears to be able to reduce oxidative damage and attenuate inflammatory responses.
Our current results suggest that pPolyHb has a protective effect on focal cerebral I/R injury. Further studies will need to be conducted to confirm these findings and better understand its protective mechanism in I/R injury.
Conclusion
We explored the role of a novel HBOC, porcine Polymerized Hemoglobin (pPolyHb), on focal cerebral I/R injury. pPolyHb was shown to ameliorate I/R injury, and also reduce the expression of inflammatory cytokines and markers of oxidative damage. These results support further therapeutic development of pPolyHb.
Acknowledgements
We acknowledge with thanks the grants from the National High-tech R&D Program (863 Program) (grant nos. 2012AA021902), National Natural Science Foundation of China (grant nos. 81102367), China Scholarship Council of the Ministry of Education, as well as grants from the Shaanxi Science and Technology Department (grant nos.2011KTCL03-23 and 2011 K12-03-10).
Declaration of interest
The authors report no conflicts of interest. The authors alone are responsible for the content and writing of the paper.
References
- Bian Y, Chang TMS. 2015. A novel nanobiotherapeutic poly-[hemoglobin-superoxide dismutase-catalase-carbonic anhydrase] with no cardiac toxicity for the resuscitation of a rat model with 90 minutes of sustained severe hemorrhagic shock with loss of 2/3 blood volume. Artif Cells Nanomed Biotechnol. 1–9.
- Briyal S, Shah S, Gulati A. 2014. Neuroprotective and anti-apoptotic effects of liraglutide in the rat brain following focal cerebral ischemia. Neuroscience. 281:269–281.
- Candelario-Jalil E. 2009. Injury and repair mechanisms in ischemic stroke: considerations for the development of novel neurotherapeutics. Curr Opin Investig Drugs. 10:644–654.
- Caswell JE, Strange MB, Rimmer DM 3rd, Gibson MF, Cole P, Lefer DJ. 2005. A novel hemoglobin-based blood substitute protects against myocardial reperfusion injury. Am J Physiol Heart Circ Physiol. 288:H1796–1801.
- Chang TMS. 1997. Blood Substitutes: Principles, Methods, Products and Clinical Trials. vol. 1. Karger, Basel (full text available for free online viewing at www.artcell.mcgill.ca).
- Doyle KP, Simon RP, Stenzel-Poore MP. 2008. Mechanisms of ischemic brain damage. Neuropharmacology. 55:310–318.
- Garcia JH, Wagner S, Liu KF, Hu XJ. 1995. Neurological deficit and extent of neuronal necrosis attributable to middle cerebral artery occlusion in rats Statistical validation. Stroke. 26:627–635.
- Han HS, Yenari MA. 2003. Cellular targets of brain inflammation in stroke. Curr Opin Investig Drugs (London, England: 2000). 4:522–529.
- Hofland J, Henny CP. 2007. Bloodless (liver) surgery? The anesthetist's view. Dig Surg. 24:265–273.
- Koizumi J, Yoshida Y, Nakazawa T, Ooneda G. 1986. Experimental studies of ischemic brain edema, I: a new experimental model of cerebral embolism in rats in which recirculation can be introduced in the ischemic area. Jpn J stroke. 8:1–8.
- Li T, Yu R, Zhang HH, Wang H, Liang WG, Yang XM, Yang CM. 2006. A method for purification and viral inactivation of human placenta hemoglobin. Artif Cells Blood Substitut Immobil Biotechnol. 34:175–188.
- Longa EZ, Weinstein PR, Carlson S, Cummins R. 1989. Reversible middle cerebral artery occlusion without craniectomy in rats. stroke. 20:84–91.
- Mahajan SK, Kashyap R, Sood BR, Jaret P, Mokta J, Kaushik NK, Prashar BS. 2004. Stroke at moderate altitude. JAPI. 52:699–702.
- Moore EE, Johnson JL, Cheng AM, Masuno T, Banerjee A, 2005. A Insights from studies of blood substitutes in trauma. Shock. 24: 197–205.
- Mukerji SS, Rainey RN, Rhodes JL, Hall AK. 2009. Delayed activin A administration attenuates tissue death after transient focal cerebral ischemia and is associated with decreased stress-responsive kinase activation. J Neurochem. 111:1138–1148.
- Peng B, Guo QL, He ZJ, Ye Z, Yuan YJ, Wang N, Zhou J. 2012. Remote ischemic postconditioning protects the brain from global cerebral ischemia/reperfusion injury by up-regulating endothelial nitric oxide synthase through the PI3K/Akt pathway. Brain Res. 1445:92–102.
- Pinar HU, Pinar A, Mavioğlu Ö, Yener N. 2015. Effect of hydroxyethyl starch 130/0.4 on ischemia-reperfusion determinants in minor lower extremity surgery with tourniquet application. J Clin Anesth. 27:105–110.
- Powanda DD, Chang TM. 2002. Cross-linked polyhemoglobin-superoxide dismutase-catalase supplies oxygen without causing blood brain barrier disruption or brain edema in a rat model of transient global brain ischemia-reperfusion. Artif Cells Blood Substit Immobil Biotechnol. 30:23–37.
- Rittoo D, Gosling P, Simms MH, Smith SRG, Vohra RK. 2005. The effects of hydroxyethyl starch compared with gelofusine on activated endothelium and the systemic inflammatory response following aortic aneurysm repair. Eur J Vasc Endovasc Surg. 30:520–524.
- Ritz MF, Curin Y, Mendelowitsch A, Andriantsitohaina R. 2008. Acute treatment with red wine polyphenols protects from ischemia-induced excitotoxicity, energy failure and oxidative stress in rats. Brain Res. 1239:226–234.
- Roger VL, Go AS, Lloyd-Jones DM, Adams RJ, Berry JD, Brown TM, et al. 2011. Heart disease and stroke statistics—2011 update a report from the American Heart Association. Circulation. 123:e18–e209.
- Seiffge DJ, Lapina NE, Tsagogiorgas C, Theisinger B, Henning RH, Schilling L. 2012. Improvement of oxygen supply by an artificial carrier in combination with normobaric oxygenation decreases the volume of tissue hypoxia and tissue damage from transient focal cerebral ischemia. Exp Neurol. 237:18–25.
- Shimbo D, Abumiya T, Shichinohe H, Nakayama N, Kazumata K, Houkin K. 2014. Post-ischemic intra-arterial infusion of liposome-encapsulated hemoglobin can reduce ischemia reperfusion injury. J Brain research. 1554:59–66.
- Standl T, Freitag M, Burmeister MA, Horn EP, Wilhelm S, Am Esch JS. 2003. Hemoglobin-based oxygen carrier HBOC-201 provides higher and faster increase in oxygen tension in skeletal muscle of anemic dogs than do stored red blood cells. J Vasc. Surg. 37:859–865.
- Tsubota H, Marui A, Esaki J, Bir SC, Ikeda T, Sakata R. 2010. Remote postconditioning may attenuate ischaemia–reperfusion injury in the murine hindlimb through adenosine receptor activation. Eur J Vasc Endovasc Surg. 40:804–809.
- Tu Q, Wang R, Ding B, Zhong W, Cao H. 2013. Protective and antioxidant effect of Danshen polysaccharides on cerebral ischemia/reperfusion injury in rats. Int J Biol Macromol. 60:268–271.
- Wang Q, Peng Y, Chen S, Gou X, Hu B, Du J, Xiong L. 2009. Pretreatment with electroacupuncture induces rapid tolerance to focal cerebral ischemia through regulation of endocannabinoid system. Stroke. 40:2157–2164.
- Wu W, Yang Q, Li T, Zhang P, Zhou R, Yang C. 2009. Hemoglobin-based oxygen carriers combined with anticancer drugs may enhance sensitivity of radiotherapy and chemotherapy to solid tumors. Artif Cells Blood Substit Immobil Biotechnol.37:163–165.
- Zhang W, Yan K, Dai P, Tian J, Zhu H, Chen C. 2012. A Novel Hemoglobin-Based Oxygen Carrier, Polymerized Porcine Hemoglobin, Inhibits H2O2-Induced Cytotoxicity of Endothelial Cells. Artif Organs. 36:151–160.
- Zhao H, Ren C, Chen X, Shen J. 2012. From rapid to delayed and remote postconditioning: the evolving concept of ischemic postconditioning in brain ischemia. Curr Drug Targets. 13:173.
- Zhou XQ, Zeng XN, Kong H, Sun XL. 2008. Neuroprotective effects of berberine on stroke models in vitro and in vivo. Neurosci Lett. 447:31–36.
- Zhu HL, Dang XD, Yan KP, Dai PG, Ma J, Li Y, et al. 2011a. Pharmacodynamic Study of Polymerized Porcine Hemoglobin (pPolyHb) in a Rat Model of Exchange Transfusion. Artif Cells Blood Substit Immobil Biotechnol. 39:119–26.
- Zhu HL, Yan KP, Dang XJ, Huang H, Chen E, Chen B, et al. 2011b. Immune safety evaluation of polymerized porcine hemoglobin (pPolyHb): a potential red blood cell substitute. Artif Cells Blood Substit Immobil Biotechnol.39:398–405.