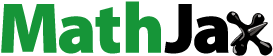
Abstract
In this paper, chitosan-graft-polyacrylamide (CS-g-PAAm) microspheres as drug delivery matrices of paracetamol were prepared by the emulsion crosslinking technique, using glutaraldehyde (GA) as a crosslinker. Graft copolymer of chitosan with acrylamide was synthesized using cerium (IV) ammonium nitrate (CAN). The microspheres formed had average particle sizes in the range of 78–252 μm. Paracetamol entrapment efficiency was found to vary between 31.89% and 72.61%, as determined by UV spectroscopy. Drug release in acidic and phosphate buffer solutions (pH 1.2 and 7.4) of the CS-g-PAAm microspheres was influenced by formulation factors such as the concentration of CS-g-PAAm, the paracetamol/polymer ratio (w/w), and the amount of crosslinker.
Introduction
Paracetamol (acetaminophen) is a popular nonsteroidal anti-inflammatory drug (NSAID) used widely in the treatment of pain and fever (CitationVijayakaran et al. 2014). It is usually administered orally, in tablet and liquid form. The absorption of paracetamol is rapid, and it is mainly absorbed in the small intestine. (CitationMiyazaki et al. 2003). The frequent dosing pattern shows that there is a need for developing a sustained- or controlled-release oral formulation (CitationGrassia et al. 2003). On the other hand, an overdose of paracetamol often leads to fatal liver damage, which manifests as localized centrilobular cell death (CitationLai and Tsiang 2005). Such problems can be overcome by formulation of controlled-release dosage forms of the drug. Controlled drug delivery products generally provide a more controlled rate of assumption of the drug by the body, improving its therapeutic action (CitationDuarte et al. 2006). For instance, Zhou et al. (CitationZhou et al. 2012) prepared chitosan (CS) CS-acetaminophen drug-loaded microspheres by the emulsification-crosslinking method, and investigated the sustained release of acetaminophen from the microspheres. CitationEnriquez et al. (2014) prepared a drug delivery system with polymeric microspheres, using acetaminophen and ibuprofen as model drugs, and found that the microsphere delivery system shows potential for the development of oral, extended-release pharmaceutical dosage forms. Fujimori et al. (CitationFujimori et al. 1995) prepared two types of magnetically responsive sustained-release tablets using acetaminophen as the model drug. They found that in the development of more efficient controlled release systems for oral formulations, the optimum combination of the rate of drug release from the systems and the control of gastric emptying time should be achieved.
Polymeric drug-loaded microspheres are one of the controlled drug delivery products specially prepared from biocompatible and biodegradable carbohydrate polymers, and have been extensively used in recent years in pharmaceutical and biomedical applications (CitationKumbar and Aminabhavi 2003, CitationGuerrero et al. 2010, CitationAL-Kahtani et al. 2014). The administration of medication via such formulations can be advantageous because of the injection or ingestion of the microspheres; they can be tailored for desired release profiles, and in some cases, can even provide organ-targeted release (CitationFreiberg and Zhu 2004). CS, used as an important biomaterial in the preparation of the microspheres, is a natural polysaccharide derived from alkaline deacetylation of chitin, and it is the second most abundant biopolymer after cellulose (CitationPeng at al. 2010). It is widely used in developing controlled release devices due to the bioadhesive, biocompatible, and biodegradable polymer properties (CitationAL-Kahtani Ahmed et al. 2009, CitationAngadi et al. 2010, CitationZhou et al. 2014). CitationAL-Kahtani Ahmed et al. (2009) developed glutaraldehyde (GA)-crosslinked pH-sensitive semi-interpenetrating network (IPN) microspheres based on CS and acrylamide-grafted hydroxyethyl cellulose (AAm-g-HEC), and investigated the release profile of diclofenac sodium (DS) from the microspheres in simulated gastric fluid of pH 1.2 for the initial 2 h, then in simulated intestinal fluid of pH 7.4, for 10 h. They found that although the release rate of the microspheres was initially fast, it gradually became slow. Zhou et al. (CitationZhou et al. 2014) prepared CS microspheres with different degrees of deacetylation by the water-in-oil emulsification method, and found that acetylated CS microspheres could be used as potential biocompatible and biodegradable embolic agents.
Despite various applications of CS, it is a weak base because of its insolubility in neutral or basic conditions; however, it is soluble in acidic pH media (pH < 6.5) (CitationSinha et al. 2004). This negative characteristic limits its usefulness in some applications (CitationJia et al. 2001). Therefore, the graft copolymerization of CS with vinyl monomers or other natural monomers is an effective way of improving its performance and solubility (CitationBulut 2015). Acrylamide (AAm) is widely used to prepare hydrogels designed for drug release (CitationPulat et al. 2014). Some studies on preparing anionic or cationic hydrogels have been found in the literature (CitationPulat and Ekşi 2006, CitationPulat et al. 2014). Also, terpolymers and copolymers of AAm offer many advantages, such as high permeability and high mechanical strength (CitationDowns et al. 1992, CitationAL-Kahtani Ahmed et al. 2009). Graft copolymers of CS-graft-polyacrylamide (CS-g-PAAm) have been widely prepared by first generating free radicals on the CS backbone using the chemical initiators (CitationKim et al. 1987, CitationKumbar et al. 2003, CitationAkbar Ali and Singh 2009, CitationYuan et al. 2010). Kumbar et al. (CitationKumbar et al. 2003) synthesized a graft copolymer of CS by a persulfate-induced free-radical reaction by grafting with polyacrylamide to modify CS, and then prepared microspheres for the controlled release of indomethacin from the copolymer.
In previous work, CS-g-PAAm has been synthesized by CAN-induced free-radical graft polymerization, followed by the development of IPN microspheres of CS-g-PAAm and methylcellulose (MC) using glutaraldehyde (GA) as a crosslinker, under various preparation conditions (CitationBulut 2015). In this study, the aim is to design, characterize, and evaluate the CS-g-PAAm microspheres of paracetamol with controlled release properties, by grafting acrylamide onto CS to enhance the properties of the polymer matrix. CS-g-PAAm microspheres were prepared by a water-in-oil (W/O) emulsion crosslinking method using GA as a crosslinker, which is most commonly used to prepare polymeric drug-loaded microspheres. The CS-g-PAAm microspheres were characterized by various techniques, and the effect of various parameters on drug release was evaluated.
Materials and methods
Materials
The gift sample of paracetamol was kindly supplied by Atabay Co. (Turkey). CS (highly viscous) was purchased from Sigma Chemical Co. (St. Louis, USA). Liquid paraffin was supplied by Aklar Chemistry Co. (Turkey). Analytical reagent-grade samples of AAm, GA (25%, w/w) solution, Span® 80, acetic acid, hydrochloric acid, n-hexane, acetone, Na2HPO4, and NaH2PO4 were all purchased from Merck (Darmstadt, Germany). Cerium (IV) ammonium nitrate (CAN) was supplied by Sigma-Aldrich Chemical Co. (St. Louis, USA).
Synthesis of the graft copolymer of chitosan
The reaction of grafting CS with AAm, as described previously (CitationBulut 2015), was carried out under a nitrogen atmosphere in a constant temperature bath (50°C) (CitationKim et al. 1987, CitationJoshi and Sinha 2007, CitationAl-Karawia et al. 2011). Briefly, 2 g of CS was dissolved in 100 mL of a 2% (w/v) aqueous acetic acid solution in hot water at 50°C, with constant stirring. The solution of CS was degassed under a slow stream of nitrogen gas for 15 min. After that, AAm solution was added to the CS solution and mixed for 15 min under a slow stream of nitrogen gas. At the end of this period, CAN at the required concentration was added slowly to the reaction mixture. A continuous supply of nitrogen was maintained throughout the polymerization reaction period. The grafting reaction was carried out for 6 h at a temperature of 50°C. At the end of 6 h, the reaction solution was added into an excess amount of acetone to precipitate the polymer, which was then filtered through the suction. The copolymer formed was dried in a vacuum oven (Medcenter, Einrichtungen GmbH, Germany) at 50°C. The reaction scheme of the graft copolymerization was shown in a previous study (CitationBulut 2015). The percentage of grafting was calculated according to the following equation:
where Wo and Wg denote the weights of the ungrafted CS and grafted CS, respectively.
Preparation of microspheres
The CS-g-PAAm microspheres containing paracetamol were prepared by the emulsion crosslinking method, with 3 mL, 6 mL, and 9 mL of GA as a crosslinker. CS-g-PAAm (from 1%–2% (w/v) in 2% (w/v) aqueous acetic acid solution) and a known amount of paracetamol were mixed and stirred well for 12 h, until a homogenous solution was obtained. This solution was emulsified in light liquid paraffin in a ratio of 1/5 (v/v) containing 2% (w/v) Span® 80, by magnetic stirring (Nuve, Turkey) at 400 rpm for about 10 min. Then, different quantities of GA were added slowly to this emulsion, and stirring was continued for 2 h. The microspheres formed were collected by filtration, washed with n-hexane, and then dried completely in an oven (Memmert, Germany) at 40°C (CitationBulut and Dilek 2014). Unloaded microspheres were prepared in a similar way without paracetamol. A total of 11 formulations were prepared, and have been listed in .
Table I. Preparation conditions and characterization of the paracetamol-loaded microspheres.
Characterization
The graft copolymer was characterized in detail in a previous study (Bulut2015). Fourier transform infrared (FTIR) spectra of paracetamol, CS-g-PAAm copolymer, and empty and paracetamol-loaded CS-g-PAAm microspheres were recorded with a Perkin-Elmer BX-II (Germany) spectrometer in the range of 400–4000 cm− 1, using potassium bromide discs at ambient temperature. A differential scanning calorimeter (DSC) (Shimadzu, Japan) was used to obtain measurements. Each sample (5–8 mg) of (a) paracetamol, (b) paracetamol-loaded CS-g-PAAm microspheres, and (c) empty CS-g-PAAm microspheres was placed in an aluminum pan. An empty aluminum pan was used as a reference. The samples were heated from 30°C to 400°C, at a heating rate of 10°C/min, in a nitrogen atmosphere. Scanning electron microscopy (SEM) photographs of the microspheres were recorded using a LEO 1430 VP (Germany) scanning electron microscope to examine the surface structure of the microspheres at the required magnification at room temperature. The microspheres were deposited on a brass holder and sputtered with a thin coating of gold under vacuum. The particle size of the microspheres was measured using optical microscopy (Olympus CH20BIMF200, Japan). The results for mean particle size (μm) are shown in .
Determination of paracetamol content
The paracetamol content in the microspheres was evaluated by extraction of the drug. For this purpose, microspheres of a known amount were crushed in an agate mortar with a pestle, and the polymeric powder obtained was refluxed with 100 mL of distilled water for 4 h under ambient conditions to ensure the complete extraction of paracetamol from the microspheres. At the end of 4 h, the precipitated polymer was filtered and the clear solution was analyzed by a UV–Vis spectrophotometer (Shimadzu UV-1700 Pharma) at a wavelength of 243 nm, with distilled water as a blank. The entrapment efficiency (%) was calculated as
Swelling studies
The equilibrium swelling degree (ESD) of the empty microspheres was determined by the gravimetric measurement of the extent of their swelling at pH levels of 1.2 (0.1 N HCl) and 7.4 (phosphate buffer solution), at 37°C. On the other hand, equilibrium swelling studies of paracetamol-loaded microsphere formulations were performed at pH 1.2, at 37°C. To ensure complete equilibration, the microsphere samples were allowed to swell for 24 h, and then filtered. The excess surface-adhered liquid drops were removed by blotting with a soft tissue paper for a few seconds. The swollen microspheres were weighed with an electronic balance (Sartorius, Korea), and then dried in an oven at 40°C, until there was no change in the dried mass of the samples. The ESD (%) was calculated as (CitationIşıklan et al. 2011)
Drug release studies
Paracetamol release from microspheres with different paracetamol/polymer ratios (1/1, 1/3 and 1/5), different amounts of GA (2 mL, 3 mL, and 5 mL), and different concentrations of CS-g-PAAm (1%, 1.5%, and 2%) were investigated in 100 ml of 0.1 N HCl (pH 1.2) for the initial 2 h, and subsequently in phosphate buffer solution (pH 7.4), incubated in a shaking water bath (Daihan WSB-18, Korea) at a speed of 100 rpm at 37°C. At the end of 2 h, the pH of the paracetamol release medium was changed from 1.2 to 7.4. A quantity of 2 mL of the solution was withdrawn at specific time intervals and the paracetamol content was determined using an UV spectrophotometer at 243 nm. Experiments were performed in triplicate, to minimize the variational error. The average values were used for further data treatment and plotting. Equal volumes of fresh HCl or phosphate buffer solution were added to the release medium to maintain constant volume.
Results and discussion
DSC studies
The DSC curves for paracetamol (curve a), paracetamol-loaded CS-g-PAAm microspheres (curve b), and empty CS-g-PAAm microspheres (curve c) are displayed in . Paracetamol exhibits a sharp endothermic peak at 171°C, which is due to the melting of paracetamol, but there was no peak corresponding to paracetamol in the curve of paracetamol-loaded microspheres, suggesting that the drug in the microspheres was molecularly dispersed in the polymer matrix.
Fourier spectral studies
Infrared (IR) spectroscopy is a useful tool to confirm the chemical stability of the paracetamol in the microspheres and the presence of crosslinking in CS-g-PAAm matrix, and also to characterize the CS-g-PAAm copolymer. exhibits the FTIR spectra of the CS-g-PAAm (a), paracetamol (b), empty microspheres (c), and paracetamol-loaded CS-g-PAAm microspheres (d). The IR spectra of CS-g-PAAm (a) shows a broad band at around 3356 cm− 1 due to the O–H stretching vibration of the hydroxyl groups. The peaks at around 1667 cm− 1 (Amide I) and 1614 cm− 1 (Amide II) are due to grafted PAAm chains at CS, as reported in the previous study (CitationBulut 2015). Moreover, a new peak that appeared at 3193 cm− 1 corresponds to the bonded –NH stretching vibrations (CitationKumbar et al. 2003). These FTIR spectra demonstrate that acrylamide was successfully grafted onto the CS backbone.
Figure 2. FTIR results of CS-g-PAAm (a), paracetamol (b), CS-g-PAAm empty microspheres (c), and paracetamol-loaded CS-g-PAAm microspheres (d).
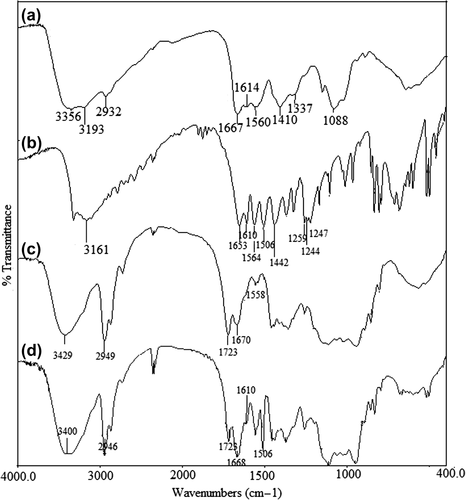
The FTIR spectra of paracetamol (b) shows characteristic bands, which are attributed to Ar–OH stretching at around 3326 cm− 1, Ar–H stretching at around 3161 cm− 1, C = O stretching at around 1653 cm− 1, ArC = H stretching at around 1610 cm− 1, N–H stretching at around 1564 cm− 1, CH3 stretching at around 1442 cm− 1, and phenyl–CH stretching vibrations at around 1259, 1244, and 1227 cm− 1 (CitationPatra et al. 2015). The chemical structure of paracetamol has been shown in . The spectra of paracetamol-loaded microspheres are different from the spectra of empty microspheres. Peaks in the paracetamol-loaded microspheres are sharper compared to those of the empty microspheres. Moreover, the characteristic bands of paracetamol observed at 1610 and 1506 cm− 1 appeared in the paracetamol-loaded microspheres, which indicates the chemical stability of paracetamol in the CS-g-PAAm polymeric microspheres. Additionally, a new peak in the spectra of the empty and drug-loaded microspheres, at 1723 cm− 1 (CitationAgnihotri and Aminabhavi 2006), is attributed to the presence of the unreacted GA, which further indicates the chemical stability of paracetamol in the presence of GA. In addition, in the spectra of the empty microspheres (c), a band at 1670 cm− 1 reveals the overlapping of the Amide I band of the CS-g-PAAm and the C = N stretching vibration band of the imine group. This status confirms the crosslinking reaction between free –NH2 groups of the CS-g-PAAm and the aldehyde groups of GA (CitationRokhade et al. 2007, CitationBulut 2015).
Evaluation of the shapes and surface morphology of the microspheres
The shapes and surface morphology of the microspheres were examined by SEM. SEM micrographs of (a) dried paracetamol-loaded microspheres at 1500x, (b) surface of the dried paracetamol-loaded microspheres at 30,000x, (c) dried empty microspheres at 960x, and (d) surface of the empty microspheres at 30,000x, taken at 1500x, 960x, and 30000x magnifications are shown in . As seen from the figure, the microspheres produced have almost a spherical shape. Empty microspheres show a smooth surface, whereas paracetamol-loaded microspheres show roughness in the surface.
Evaluation of entrapment efficiency and particle size of the microspheres
Many factors, such as the extent of crosslinking, composition of the drug and polymer, and concentration of copolymer, affect the entrapment efficiency (%) and particle size (μm). The results of studies on % entrapment efficiency, displayed in , show a decrease with increasing amount of the crosslinker added. The highest EE was observed as 70.20% for the microspheres prepared with 2 mL of GA and a paracetamol/polymer ratio of 1/5, while the minimum was 31.89% for the microspheres prepared with 5 mL of GA and a paracetamol/polymer ratio of 1/1. Such a decreasing trend is because the microspheres have a more rigid structure, with an increase in crosslink density, and hence, the volume of free space within the polymer structure decreases, leading to a reduction in EE. Similar results were also found in the literature. (CitationMundargi et al. 2008, CitationRamesh Babu et al. 2008). Mundargi et al. (CitationMundargi et al. 2008) studied the oral controlled release of ibuprofen from sequential IPN microspheres of poly(methacrylic acid) and poly(vinyl alcohol). They reported that as the concentration of crosslinking agent (GA) increases, EE decreases, due to the formation of a rigid microspheric structure. Also, in the microspheres, as the drug/polymer ratio increased from 1/5 to 1/1, the % EE decreased. Similar observations were also reported in a previous study (CitationBulut and Şanlı 2014). On the other hand, % EE increased with increasing CS-g-PAAm concentration. The highest EE was found to be 72.61%, at the CS-g-PAAm concentration of 2.0%.
The measured values of diameter of the microspheres produced as a result of changing paracetamol/polymer (w/w) ratio, amount of GA, and concentration of CS-g-PAAm are shown in . The particle size of the microspheres was measured using optical microscopy. The microspheres formed had average particle sizes, ranging from 78.0 μm to 252.0 μm in diameter. The results showed that in the formulations, the diameters of the microspheres showed a dependence on the extent of crosslinking, amount of drug present, and polymer concentration. For instance, with increasing amount of GA added, the particle size decreased due to the shrinkage of the polymeric microsphere. Banerjee et al. (CitationBanerjee et al. 2012) prepared IPN microspheres from sodium carboxymethyl cellulose (NaCMC) and poly(vinyl alcohol) (PVA) for oral controlled-release delivery of diclofenac sodium, and reported that particle size decreased with an increasing extent of crosslinking density, which may be due to rapid shrinking of the polymeric network during crosslinking. Also, with increasing amount of drug in the microspheres, the particle size of the microspheres decreased. A similar observation was also reported in the earlier study (CitationŞanlı et al. 2008). On the other hand, the diameter of the microspheres prepared with CS-g-PAAm concentrations of 1.5% and 2.0% was greater than that of the other microsphere formulations.
Drug release kinetics
The kinetics of drug release from the microspheres were tested by fitting the fraction release data Mt/M∞ using the empirical equations proposed by Ritger and Peppas (CitationRitger and Peppas 1987)
For the equation, Mt/M∞ is the fraction of drug released at time t; n is an empirical parameter characterizing the release mechanism; and k is a constant, incorporating the structural and geometrical character of the dosage form. Using the least squares procedure, the values of k, n, and correlation coefficient (r) are presented in . In spherical polymeric matrices, the dominant mechanism, if n ≤ 0.43, is Fickian diffusion (case I), if 0.43 < n < 0.85, anomalous or non-Fickian transport, and if n ≥ 0.85, case-II transport drug release. According to the data in , the n value ranged between 0.8044 and 0.9160, with a correlation coefficient close to 0.99, indicating that paracetamol release from the microspheres followed mainly case II transport, where drug release is governed by polymer relaxation processes (CitationPeppas 1985, CitationRitger and Peppas 1987).
Table II. Parameters of release kinetics of different formulations.
The diffusion coefficient (D) of water absorption or drug release through microspheres can be computed using the equation as follows (CitationAgnihotri and Aminabhavi 2006):
here, M∞ is the maximum drug release, r is radius of the dry microspheres, and ϴ is slope of the linear portion of the plot of Mt/M∞ vs. t1/2. The relationship between D and the formulation parameters is shown in . It is noticeable that the D values decreased by increasing the extent of crosslinking for all microsphere formulations, which was also in agreement with the release results. The D values also decreased with increasing paracetamol/polymer ratio.
In vitro drug release
To understand the in vitro release of paracetamol from CS-g-PAAm microspheres, the study was conducted successively under conditions of gastric pH (1.2) for the initial 2 h, then intestinal pH (7.4) for 4 h, at 37°C. Many parameters, such as extent of crosslinking, composition of the drug and polymer, and concentration of copolymer affected the paracetamol release from the microspheres. display the effect of these parameters in gastric (1.2) and intestinal (7.4) pH conditions, respectively. As seen from the Figures (4–10), the paracetamol release rate for all microsphere formulations was initially fast, but it gradually became more controlled.
Figure 4. Effect of amount of GA on paracetamol release. CS-g-PAAm concentration: 1%, paracetamol/polymer ratio: 1/1, exposure time to GA: 2 h.
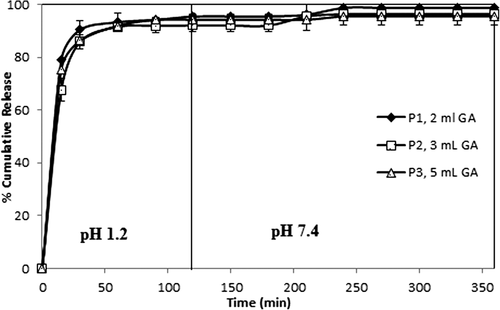
Figure 5. Effect of amount of GA on paracetamol release. CS-g-PAAm concentration: 1%, paracetamol/polymer ratio: 1/3, exposure time to GA: 2 h.
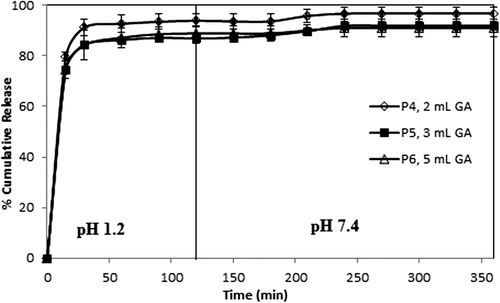
Figure 6. Effect of amount of GA on paracetamol release. CS-g-PAAm concentration: 1%, paracetamol/polymer ratio: 1/5, exposure time to GA: 2 h.
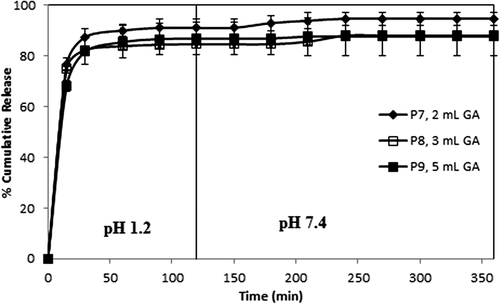
Figure 7. Effect of paracetamol/polymer ratio on paracetamol release. CS-g-PAAm concentration: 1%, amount of GA: 2 mL, exposure time to GA: 2 h.
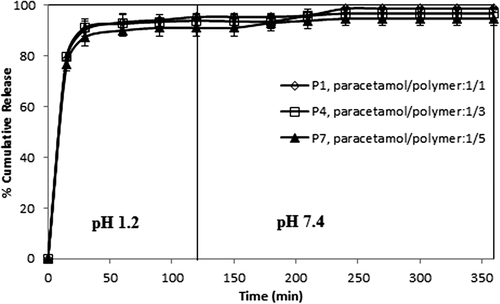
Figure 8. Effect of paracetamol/polymer ratio on paracetamol release. CS-g-PAAm concentration: 1%, amount of GA: 3 mL, exposure time to GA: 2 h.
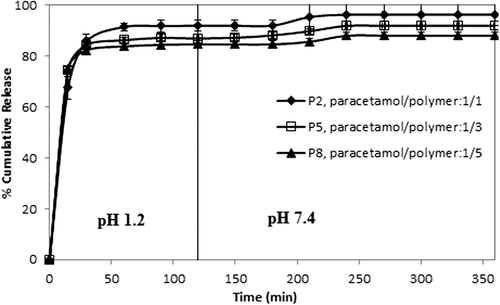
Effect of crosslinking agent on paracetamol release
The curves indicating the percent cumulative release vs time (min) with varying amounts of GA (with 1/1, 1/3, and 1/5 (w/w) paracetamol/polymer ratios and 2 h of crosslinking time) are displayed in , indicating that as the amount of the GA increased, cumulative paracetamol release decreased, along with a decrease in the burst effect. These decreases in the cumulative release are due to the formation of a more rigid polymeric structure; therefore, this status causes the formation of more small voids. Similar results were also reported in the literature (AL-Kahtani et al. 2009, CitationSwamy et al. 2013). In a study by Swamy et al., they investigated the release of enalapril maleate as a model drug (for hypertension) from the biodegradable CS-g-poly(methacrylamide) microspheres, and reported that the percentage of cumulative drug release of the microspheres prepared with 10 mL of GA was lower than that of microspheres prepared with 5.0 mL of GA.
On the other hand, the results were supported by the swelling measurements, given in . As seen from , microspheres including higher amounts of GA showed lower swelling values as compared to microspheres including lower amounts of GA, due to the formation of a tighter network structure and decreased pore volume of the matrix (CitationBabu et al. 2007).
Table III. Equilibrium swelling degree (% ESD) for the empty microspheres.
Effect of the paracetamol/polymer ratio on paracetamol release
The drug/polymer ratio showed some small effects on paracetamol release, as shown in . For this purpose, formulations with different paracetamol/polymer ratios (1/1, 1/3, and 1/5) (w/w)) were chosen, with 2, 3, and 5 mL of GA for 2 h of crosslinking time. The release data in demonstrate that as the amount of drug in the microspheres increased, the release of paracetamol slightly increased. The values for maximum cumulative paracetamol release for the microspheres with a 1/1 paracetamol/polymer ratio, with different amounts of GA (2, 3, and 5 mL), were obtained as 98.70%, 96.33%, and 95.45, respectively. The release results can be explained by the fact that at the lower paracetamol content in the polymeric matrix, the release rates become slower, because of the availability of more free void spaces through which fewer drug molecules will be transported. Similar observations have been found in the literature and previous studies (CitationRamesh Babu et al. 2006; CitationBulut and Dilek 2014, CitationBulut 2015). For instance, our group (CitationBulut and Dilek 2014) previously investigated the controlled release of ibuprofen through pH-sensitive locust bean gum–alginate polymeric microspheres, and reported that cumulative drug release becomes rather higher with a high amount of drug molecules in the matrix. Also, a similar finding was reported for chlorothiazide-loaded semi-IPN network microspheres of CS-N,N′-dimethylacrylamide, studied by Ramesh Babu et al. (CitationRamesh Babu et al. 2008).
Swelling studies
Equilibrium swelling experiments were performed at a pH of 1.2 for paracetamol-loaded microsphere formulations, and the results have been presented in . As can be seen from the table, as the amount of drug in the microspheres was increased, swelling capacity of the microspheres increased. The empty microspheres and the microspheres containing lower amounts of drug displayed low swelling degrees. In other words, the presence of drug in the polymeric matrix played a vital role in swelling values. Similar results were obtained by Babu et al. (CitationRamesh Babu et al. 2008). On the other hand, increasing the amount of GA caused the decrease in swelling capacity of the microspheres because of intensive crosslinking (CitationPulat et al. 2011).
Effect of CS-g-PAAm concentration on paracetamol release
Three different concentrations of CS-g-PAAm (1%, 1.5% and 2.0%) were used for the preparation of the microspheres. The effect of the CS-g-PAAm concentration on the release of paracetamol is shown in . The results of the release study indicated that an increase in the concentration of CS-g-PAAm decreased the amount of drug release from the microspheres. CS has different types of hydrophilic functional groups, and an increase in the concentration of the microsphere preparation solution leads to an increase in the number hydrophilic functional groups. Therefore, this results in a decrease of paracetamol release due to the presence of more functional groups crosslinked with the GA. Isıklan et al. (CitationIşıklan et al. 2011) also found similar results in the study of itaconic acid-grafted alginate microspheres containing nifedipine.
Conclusions
In this study, the hydrophilic nature of acrylamide-grafted CS was utilized to develop biocompatible microspheres by an emulsion crosslinking technique, using GA as a crosslinking agent. Paracetamol, a nonsteroidal anti-inflammatory drug, was successfully encapsulated into the microspheres, with the highest percentage of 72.61. Paracetamol-loaded microspheres were obtained with a narrow size distribution, with sizes ranging from 78 μm to 252 μm, with an almost spherical structure. The release of paracetamol from the microspheres varied depending upon the pH of the medium, the amount of GA, paracetamol/polymer ratio, and concentration of CS-g-PAAm. The release of paracetamol was found to be much higher at a pH of 1.2 compared to that at a pH of 7.4. Drug release from the microspheres occured in two steps: a burst release in about the first hour, probably due to the high aqueous solubility of paracetamol, and a controlled release in the later hours, due to paracetamol–polymer interaction. Drug release became more controlled with an increase in the extent of GA and concentration of CS-g-PAAm, and a decrease in the paracetamol/polymer ratio. The highest paracetamol release percentage was obtained as 98.70% for the paracetamol/polymer ratio of 1/1, GA amount of 2 mL, crosslinking time of 2 h, and CS-g-PAAm concentration of 1%, at the end of 6 h. The n values calculated for the release of drug from the microspheres indicated that paracetamol release followed case II transport, in which drug release is governed by polymer relaxation processes.
Declaration of interest
The author is responsible for the content and writing of the article. The author is grateful to the Afyon Kocatepe University Scientific Research Foundation for their support, and to Sanovel Company for the supply of the drug (paracetamol).
References
- Agnihotri SA, Aminabhavi TM. 2006. Novel interpenetraitng network chitosan-poly(ethylene oxide-g-acrylamide) hydrogel microspheres for the controlled release of capecitabine. Int J Pharm. 324:103–115.
- Akbar Ali SK, Singh RP. 2009. An ınvestigation of the flocculation characteristics of polyacrylamide-grafted chitosan. J Appl Polym Sci. 114:2410–2414.
- AL-Kahtani Ahmed A, Naik HSB, Sherigara BS. 2009. Synthesis and characterization of chitosan-based pH-sensitive semi-interpenetrating network microspheres for controlled release of diclofenac sodium. Carbohydr Res. 344:699–706.
- AL-Kahtani Ahmed A, Sherigara BS. 2014. Controlled release of diclofenac sodium through acrylamide grafted hydroxyethyl cellulose and sodium alginate. Carbohydr Polym. 104:151–157.
- Al-Karawia AJM, Al-Qaisia ZHJ, Abdullaha HI, Al-Mokarama AMA, Al-Heetimi DTA, 2011. Synthesis, characterization of acrylamide grafted chitosan and its use in removal of copper (II) ions from water. Carbohydr Polym. 83:495–500.
- Angadi SC, Manjeshwar LS, Aminabhavi TM. 2010. Interpenetrating polymer network blend microspheres of chitosan and hydroxyethyl cellulose for controlled release of isoniazid. Int J Bio Macromol. 47:171–179.
- Babu VR, Sairam M, Hosamani KM, Aminabhavi TM. 2007. Preparation of sodium alginate–methylcellulose blend microspheres for controlled release of nifedipine. Carbohydr Polym. 69:241–250.
- Banerjee S, Siddiqui L, Bhattacharya SS, Kaity S, Ghosh A, Chattopadhyay P, et al. 2012. Interpenetrating polymer network (IPN) hydrogel microspheres for oral controlled release application. Int J Bio Macromol. 50:198–206.
- Bulut E, Dilek M. 2014. Development and characterization of pH-sensitive locust bean gum-alginate microspheres for controlled release of ibuprofen. J Drug Del Sci Tech. 24:613–619.
- Bulut E, Şanlı O. 2014. Optimization of release conditions of Alzheimer's drug donepezil hydrochloride from sodium alginate/sodium carboxymethyl cellulose blend microspheres. J Macromol Sci Part B: Phy. 53:902–917.
- Bulut E. 2015. Ibuprofen microencapsulation within acrylamide-grafted chitosan and methylcellulose interpenetrating polymer network microspheres: synthesis, characterization, and release studies. Artif Cells Nanomed Biotechnol. DOI: https://doi.org/10.3109/21691401.2015.1011802.
- Downs EC, Robertson NE, Riss TL, Plunkett ML. 1992. Calcium alginate beads as a slow-release system for delivering angiogenic molecules In Vivo and In Vitro. J Cell Physiol. 152:422–429.
- Duarte ARC, Costa MS, Simplicio AL, Cardoso, MM. Duarte, CMM. 2006. Preparation of controlled release microspheres using supercritical fluid technology for delivery of anti-inflammatory drugs. Int J Pharm. 308:168–174.
- Enriquez GG, Orawiec BA, Rizvi SAA, Do DP. 2014. Formulation development and in vitro evaluation of oral extended release capsules containing biodegradable microspheres. J Nanomed Nanotech. 5:208: DOI: https://doi.org/10.4172/2157-7439.1000208.
- Freiberg S, Zhu XX. 2004. Polymer microspheres for controlled drug release, Review. Int J Pharm. 282:1–18.
- Fujimori J, Machida Y, Tanaka S, Nagai T. 1995. Effect of magnetically controlled gastric residence of sustained release tablets on bioavailability of acetaminophen. Int J Pharm. 119:47–55.
- Grassia M, Voinovich D, Moneghini M, Franceschinis E, Perissutti B, Filipovic-Grcic J. 2003. Preparation and evaluation of a melt pelletised paracetamol/stearic acid sustained release delivery system. J Control Release. 88:381–391.
- Guerrero S, Teijón C, Muñiz E, Teijón JM, Blanco MD. 2010. Characterization and in vivo evaluation of ketotifen-loaded chitosan microspheres. Carbohydr Polym. 79:1006–1013.
- Işıklan N, İnal M, Kurşun F, Ercan G. 2011. pH responsive itaconic acid grafted alginate microspheres for the controlled release of nifedipine. Carbohydr Polym. 84:933–943.
- Jia Z, Shen D, Xu W. 2001. Synthesis and antibacterial activities of quaternary ammonium salt of chitosan. Carbohydr Res. 333:1–6.
- Joshi JM, Sinha VK. 2007. Ceric ammonium nitrate induced grafting of polyacrylamide onto carboxymethyl chitosan. Carbohydr Polym. 67:427–435.
- Kim K-H, Kim K-S, Shin J-S. 1987. Graft copolymerization of chitosan and acrylamide by using ceric ammonium nitrate initiator. Polymer (Korea). 11:133–138.
- Kumbar SG, Aminabhavi TM. 2003. Synthesis and characterization of modified chitosan microspheres: Effect of the grafting ratio on the controlled release of nifedipine through microspheres. J Appl Polym Sci. 89:2940–2949.
- Kumbar SG, Soppimath KS, Aminabhavi TM. 2003. Synthesis and characterization of polyacrylamide-grafted chitosan hydrogel microspheres for the controlled release of indomethacin. J Appl Polym Sci. 87:1525–1536.
- Lai M-K, Tsiang RC-C. 2005. Microencapsulation of acetaminophen into poly(L-lactide) by three different emulsion solvent-evaporation methods. J Microencapsul. 22:261–274.
- Miyazaki S, Endo K, Kawasaki N, Kubo W, Watanabe H, Attwood D. 2003. Oral sustained delivery of paracetamol from in situ gelling xyloglucan formulations. Drug Dev Ind Pharm. 29:113–119.
- Mundargi RC, Patil SA, Kulkarni PV, Mallikarjuna NN, Aminabhavi TM. 2008. Sequential interpenetrating polymer network hydrogel microspheres of poly(methacrylic acid) and poly(vinyl alcohol) for oral controlled drug delivery to intestine. J Microencap. 25:228–240.
- Patra CN, Swain S, Mahanty S, Panigrahi KC. 2015. Design and characterization of aceclofenac and paracetamol spherical crystals and their tableting properties. Powder Tech. 274:446–454.
- Peng H, Xiong H, Li J, Xie M, Liu Y, Bai C, Chen L. 2010. Vanillin cross-linked chitosan microspheres for controlled release of resveratrol. Food Chemy. 121:23–28.
- Peppas NA. 1985. Analysis of Fickian non Fickian drug release from polymers. Pharm Acta Helv. 60:110–111.
- Pulat M, Ekşi H. 2006. Determination of swelling behavior and morphological properties of poly(acrylamide-co-itaconic acid) and poly(acrylic acid-co-itaconic acid) copolymeric hydrogels. J Appl Polym Sci. 102:5994–5999.
- Pulat M, Oytun Akalın G, Demirkol Karahan N. 2014. Lipase release through semi-interpenetrating polymer network hydrogels based on chitosan, acrylamide, and citraconic acid. Artif Cells Nanomed Biotechnol. 42: 121–127.
- Pulat M, Tan N, Kaynak Onurdağ F. 2011. Swelling dynamics of IPN hydrogels including acrylamide-acrylic acid-chitosan and evaluation of their potential for controlled release of piperacillin-tazobactam. J Appl Polym Sci. 120: 441–450.
- Ramesh Babu V, Krishna Rao KSV, Sairam M, Naidu BVK, Hosamani KM, Aminabhavi TM. 2006. pH sensitive interpenetrating network microgels of sodium alginate-acrylic acid for the controlled release of ibuprofen. J Appl Polym Sci. 99:2671–2678.
- Ramesh Babu V, Hosamani KM, Aminabhavi TM. 2008. Preparation and in-vitro release of chlorothiazide novel pH-sensitive chitosan-N,N-dimethylacrylamide semi-interpenetrating network microspheres. Carbohydr Polym. 71:208–217.
- Ritger PL, Peppas NA. 1987. A simple equation for description of solute release. II. Fickian and anomalous release from swellable devices. J Control Rel. 5:37–42.
- Rokhade AP, Patil SA, Aminabhavi TM. 2007. Synthesis and characterization of semi-interpenetrating polymer network microspheres of acrylamide grafted dextran and chitosan for controlled release of acyclovir. Carbohydr Polym. 67:605–613.
- Sinha VR, Singla AK, Wadhawan S, Kaushik R, Kumria R., Bansal K, Dhawan S. 2004. Chitosan microspheres as a potential carrier for drugs. Review Int J Pharm. 274:1–33.
- Swamy BY, Prasad CV, Prabhakar MN, Rao KC, Subha MCS, Chung I. 2013. Biodegradable Chitosan-g-Poly(methacrylamide) Microspheres for Controlled Release of Hypertensive Drug. J Polym Environ. 21:1128–1134.
- Şanlı O, Biçer E, Işıklan N. 2008. In vitro release study of diltiazem hydrochloride from poly(vinyl pyrrolidone)/sodium alginate blend microspheres. J Appl Polym Sci. 107:1973–1980.
- Vijayakaran K, Kesavan M, Kannan K, Sankar P, Tandan SK, Sarkar SN. 2014. Arsenic decreases antinociceptive activity of paracetamol: Possible involvement of serotonergic and endocannabinoid receptors. Environ Toxicol Pharmacol. 38:397–405.
- Yuan B, Shang Y, Lu YB, Qin Z, Jiang Y, Chen A, et al. 2010. The Flocculating Properties of Chitosan-graft Polyacrylamide Flocculants (I)—Effect of the Grafting Ratio. J Appl Polym Sci. 117: 1876–1882.
- Zhou H-R, Zhang J-Y, Jiang H. 2012. The preparation and characteristics of chitosan-acetaminophen drug-loading micropheres. Adv Mater Res. 586:161–165.
- Zhou X, Kong M, Cheng XJ, Feng C, Li J, Li JJ, Chen XG. 2014. In vitro and in vivo evaluation of chitosan microspheres with different deacetylation degree as potential embolic agent. Carbohydr Polym. 113:304–313.