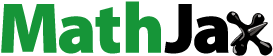
Abstract
The objective of the current investigation was to develop a novel biocomposite polymeric nanofiber for sublingual delivery of nicorandil in an attempt to reduce mucosal ulceration and to improve drug bioavailability. Polymeric nanofibers were achieved using vitamin B12 and a blend of hyaluronic acid and polyvinyl alcohol as polymeric constituents. The electrospinning method was used to prepare drug (nicorandil)-loaded nanofibers. The resulting nanofibers were characterized for morphology, drug loading, XRD, DSC, in vitro drug release, degree of swelling, and pharmacokinetic behavior. The prepared nanofibers were found to be uniform, non-beaded, and non-woven, with fiber diameter ranging from 200–450 nm. In vitro drug release substantiated the controlled release behavior of the developed formulation. Histopathology studies demonstrated no evidence of mucosal ulceration at the site of application. Pharmacokinetic studies established the preclinical safety and showed the maintenance of an effective therapeutic level for a prolonged period. The present investigation gives inputs showing that the biocomposite nanofiber assists as a perfect carrier system for sublingual delivery of anti-anginal drugs.
Introduction
Angina pectoris is a medical circumstance that happens when the heart takes a lower volume of oxygenated blood (CitationMaton 1997). Potassium channel openers are currently reflected as a significant drug class for hypertension and angina pectoris (CitationFuster and Kelly 2010). Nicorandil is the principal therapeutic particle that has a capability to hyperpolarize muscle tissues, and is an effective coronary vasodilator (CitationFrydman et al. 1989). Nicorandil seems to be active in all types of angina pectoris, including advanced coronary artery lesion (CitationChaudhary et al. 2015a). Nicorandil is an anti-anginal medicine that has the twin properties of a nitrate and K+ ATP channel agonist (CitationChaudhary et al. 2014). In humans, the nitrate exploits the nicorandil and expands the large coronary arteries at low plasma concentrations. At high plasma concentrations, nicorandil decreases coronary vascular opposition, which is related to enlarged K+ ATP channel opening (CitationChaudhary et al. 2015b). Nicorandil has some limitations like low bioavailability and slow onset of action (CitationGagandeep et al. 2014). Further, high turnover rate and mucosal ulceration are common side-effects associated with nicorandil (CitationGarg 2014). Different carrier systems have been developed for delivery of anti-anginal agents including liposomes (CitationWu et al. 2005), biodegradable particles (CitationGarg et al. 2015a), micelles (CitationGarg and Goyal 2012), dendrimers (CitationLee et al. 2005), artificial DNA nanostructures (CitationGarg and Goyal 2014b), nanoparticles (CitationGarg and Goyal 2014c), and nanogels (CitationHuang et al. 2014). However, none of the carrier systems successfully address the complications associated with nicorandil, that is, mucosal ulceration and delayed onset of action (CitationGarg and Goyal 2014a, CitationGarg et al. 2012a). Further, poor storage stability of nicorandil offers a steep challenge for formulation scientists to design an effective mechanism for the delivery of nicorandil (CitationGarg et al. 2014a, CitationGarg and Rath 2015). Recently, electrospun nanofibers have received special attention as drug delivery systems (CitationGarg et al. 2014b, Citation2014d) because of their distinct functional feature and easy fabrication techniques. The large surface-to-volume ratio (CitationGreiner and Wendorff 2007) and porous matrix (CitationGarg et al. 2014c) of nanofibers overcome the limitations of the poor drug payload which is generally associated with conventional systems (CitationGarg et al. 2015b). In addition, the microporous architecture (CitationGarg et al. 2011a) and physiochemical properties of polymers (CitationGarg et al. 2011b) offer ample flexibility to customize the drug-release profile (CitationGarg et al. 2012b). In addition to formulation variables, the drug-release kinetics can be modulated by adjusting the various process variables during the electrospinning process (CitationGarg et al. 2013, CitationGoyal et al. 2013a). Ranganath and Wang reported an encapsulation efficiency of paclitaxel of approximately 95% for PLGA nanofiber associates with a sustained release of paclitaxel over a period of 80 days (CitationRanganath and Wang 2008). In the present investigation, vitamin B12 and hyaluronic acid (HA) were selected in an attempt to minimize mucosal ulceration followed by oral administration of nicorandil. HA, being a component of the extracellular matrix, serves as a natural supplement for damaged tissue and facilitates quick recovery from a local injury and inflammation (CitationGoyal et al. 2013b, Citation2014a). Evidence further suggests that HA reduces the amount of pro-inflammatory cytotoxins, thereby showing its beneficial effects on inflammatory arthritis (CitationGoyal et al. 2014b, CitationHussain et al. 2014). In addition, the viscoelastic properties of HA ensure controlled release of entrapped drug with a prolonged retention time at the site of application (CitationJohal et al. 2014, CitationJoshi et al. 2014a). Recently, the beneficial effects of vitamin B12 in the treatment of mucosal ulceration have been established (CitationJoshi et al. 2014b, CitationKalia et al. 2014). Although the exact mechanism of action of vitamin B12 in the treatment of mucosal ulceration is not clear, it has been found that vitamin B12 restores the integrity of the mucosal lining and increases mucosal thickness, with decreased ulceration (CitationKataria et al. 2014, CitationKaur et al. 2015a). Sublingual administration of vitamin B12 may be beneficial in the treatment of mucosal ulceration, irrespective of the serum level of vitamin B12 (CitationKaur et al. 2014a, Citation2014b). In order to explore the beneficial effects of HA and vitamin B12, the present study was designed in an attempt to minimize nicorandil- induced mucosal ulceration following oral administration (CitationKaur et al. 2014c, Citation2014d). The aim of the present investigation was to prepare the polymeric nanofiber composite of vitamin B12, HA, and PVA as a formulation component for sublingual delivery of nicorandil. The developed formulation was analyzed for histopathologic and pharmacokinetic studies, to establish the therapeutic effectiveness of the developed formulation.
Material and methods
Hyaluronic acid used in this study was purchased from Shanghai Brightol international Co., Ltd. having the molecular weight of 1 × 106 gmol− 1. PVA and vitamin B12 were purchased from HiMedia Pvt. Ltd. Nicorandil was received as gift sample from United Biotech Pvt. Ltd, Baddi. All other reagents used were of analytical grade.
Preparation of drug- loaded nanofiber mats
A 2% (w/v) HA solution was prepared in distilled water at room temperature with continuous stirring for 3 h. A 10% (w/v) solution of PVA was prepared separately in distilled water. The HA and PVA were blended in different volume ratios ranging from 1/1 to 1/5 respectively, and were kept overnight under stirring at a constant speed till a clear homogeneous dispersion was obtained. The dispersions were kept under appropriate storage conditions till further use. The polymer solutions were placed into a syringe (5 mL) fixed to a needle tip (25 gauge, inner diameter 0.25 mm), and setup in the electrospinning apparatus (E-Nano, IIT Kanpur). A syringe pump was used for delivering the polymer solution at a rate of 200ul/hr. Electrospinning was conceded out at a voltage (10–15 kV, delivered directly by a high DC voltage power) and with a needle-to-collector distance of 15–20 cm. The collector was covered with a thick aluminum foil on which the nanofibers were collected. In the present work, the passive loading technique was used for drug-loading in the composite nanofiber. Briefly, a saturated dispersion of drug in preformed polymer solution was prepared, and the mixture was stirred for 3 h to obtain a clear homogeneous dispersion. Necessary precautions were taken to prevent continuous degradation during mixing. The solutions were electrospun using the above-defined optimized parameters. The drug-loaded nanofibers were collected from the aluminum foil (CitationMalik et al. 2014, CitationModgill et al. 2014b, CitationMorie et al. 2014, CitationSingh et al. 2014b).
Characterization of electrospun nanofiber
Morphology analysis
The morphology of both plain and medicated electrospun nanofibers was observed using a Hitachi S-4700 scanning electron microscope (Hitachi Company, Japan). In this technique, samples were fixed on metal ends using a double-sided adhesive tape and vacuum-coated through a gold sputter layer prior to consideration. The diameters and distributions of the electrospun fibers were examined from the SEM images using ImageJ analysis software (ImageJ, National Institutes of Health, USA). The SEM images were then used for the determination of average diameter of the fibers (CitationSharma et al. 2014c, CitationModgill et al. 2014a).
Fourier Transform Infrared (FTIR) analysis
IR Spectroscopy (Thermo Nicolet, Thermo Scientific, USA) was used to study drug–polymer interaction in a solid drug–polymer blend. The electrospun nanofiber was scratched into small parts and assorted with KBr to create sample pellets. Measurements were taken in a range of 800–4000 cm− 1 with a resolution of 2 cm− 1. Characteristic peaks observed were compared with a reference spectrum to depict any kind of interaction between drug and polymers (CitationKaur et al. 2014d, Citation2015b).
Differential scanning calorimetry (DSC)
In this technique, a weighed quantity of nanofiber was transferred into a crucible. The crucible was covered and then pressed using a pressing machine. The crucible was then fitted inside the instrument. DSC measurement was analyzed with a sample (3–5 g) heated from 0–25°C under a nitrogen atmosphere with a scanning speed of 100 C/min. The DSC thermogram so obtained was analyzed for the solid state chemistry of drug in composite polymeric nanofiber (CitationKaur et al. 2014e, Citation2014f).
X-ray diffraction (XRD)
XRD is an important analytical tool that has been used to predict the solid state of drug, which may be either crystalline or amorphous. X-ray characterization of plain nicorandil, unloaded composite nanofiber, and drug-loaded composite fiber was carried out at the 2θ value from 5–500 using the XRD instrument (CitationKaur et al. 2014g, Citation2014h).
Solubility profile
The saturation solubility of drug in the formulation developed was determined by the dynamic equilibrium method. Briefly, suitable quantities of formulations were added to the solvent, and this mixture was allowed to stand for 24 h. The solution was then filtered and quantitatively analyzed for the drug content using UV spectroscopy (CitationKaur et al. 2014i, Citation2014j).
Mucoadhesive test
The mucoadhesive detachment force plays a large role in determining the time duration of residence of the developed formulation at the application site. The Brookfield texture analyzer was used to determine the mucoadhesive detachment force of the nanofiber from goat mucosa. Firstly, goat mucosa was placed on one of the attachments of the device and nanofibers with 2 cm2 dimensions were stuck to the other attachment of the analyzer with the help of double-sided tape. Both of these were brought in contact by applying a weight of 1 kg for 15 s after base location. The force required for the fibers to detach from the mucosa was calculated (CitationKaur et al. 2014k).
Tensile strength of nanofibers
Tensile strength is a mechanical property determining the ability of the developed formulation to resist wear and tear during transportation and handling. Nanofibers having 8 cm length and 4 cm width were placed in a vertical position along the axis of the Brookfield texture analyzer, with the help of clamps. The instrument was then allowed to run with a minimum sensitivity force of 3 gm/cm2 after base location. The force required to split the mat into two pieces was determined (CitationKumar et al. 2015, CitationMalik et al. 2014, Citation2015).
Drug entrapment efficiency
Drug entrapment efficiency of the electrospun nanofiber is a function of the polymer and drug solubility in polymeric solution. It is necessary to establish a scientific rationale and a design space to identify the multidimensional combination for optimized formulation. To calculate the entrapment efficiency, we cut a 1 cm2 area of drug-loaded nanofiber and dissolved it in the solvent in which drug and polymer were soluble. The quantity of drug in the solution was calculated by UV spectrophotometer using a calibration curve (CitationMarwah et al. 2014). The entrapment efficiency was calculated using the following equation:
Degree of Swelling
The degree of swelling is a parameter which provides information about the release behavior of the drug from the nanofiber mat. The degree of swelling of nanofiber mats was calculated using the following Equation 2. The test was carried out in the simulated salivary fluid (pH 6.8) at 37°C for up to 6 h (CitationPabreja et al. 2014).
where M is the weight of swollen nanofiber sample and Md is the dried mass of immersed sample in buffer medium.
In vitro drug release studies
Drug release from electrospun nanofiber mats was evaluated by placing a known area (1 × 1 cm2) of drug-loaded nanofiber into phosphate-buffered saline solution (PBS). The release studies were carried out at 37°C at 100 rpm in a thermostatic shaking incubator. Samples were taken at pre-determined intervals from the solution after 30, 60, 90, 120, 150, 180, 210, 240, 270, 300, 330, and 360 min. The amount of drug present in the samples was determined using a UV–visible spectrophotometer at a λmax of 262 nm followed by the addition of fresh media to maintain sink conditions. The % drug release was calculated using the standard curve (CitationRohilla et al. 2014a).
Ex vivo permeability study
The permeability study was performed to study the effect of composite nanofiber on the rate of mass transfers across the goat mucosa. Nanofiber of 1 × 1 cm2 area was cut and placed in the goat mucosal membrane; the membrane was tied with thread and placed in PBS 6.8 buffer medium. Samples were withdrawn from the medium at regular intervals for 6 h. This experiment was carried out using a Franz diffusion cell. The amount of drug present in the receptor compartment was measured using a UV–visible spectrophotometer at a λmax of 262 nm (CitationRohilla et al. 2014b).
In vivo evaluation
Pharmacokinetic studies
The pharmacokinetic study was performed in an animal model (Wistar rats). The study was approved by the institutional ethics committee. Animals were housed in separate cages and had no medication for 2 weeks prior to the study. The test patch containing the calculated animal dose was applied to the sublingual section of the oral cavity. A gentle pressure was applied with a finger for 30 s to ensure good adhesion to the sublingual mucosa. The blood samples were collected at predetermined intervals from the tail vein, centrifuged, and the serum separated and stored at −20°C until further analysis. Pharmacokinetic parameters of the developed patch were compared with the marketed formulation. Relevant pharmacokinetic parameters were determined and analyzed statistically (CitationSharma et al. 2014a, Citation2014b, Citation2015).
Histopathology studies
The histopathologic effect of the nanofiber mat was tested to study the effect of the developed formulation on local tissue. The tissue was fixed with 10% formalin, routinely processed, and embedded in paraffin. Paraffin sections were cut on glass slides and stained with hematoxylin and eosin. Cellular infiltration and tissue damage were recorded with a light microscope (CitationSharma et al. 2014c, CitationSingh et al. 2012, Citation2014c).
Statistical Analysis
Statistical analysis was performed using Prism. The experiments described in this article were run in triplicate. The data were analyzed using the standard deviation of three independent experiments in triplicate.
Results and discussion
Preparation and optimization of composite nanofiber
During the process of electrospinning, all the parameters which were involved in this process, including concentration of polymer, polymer ratio in case of composite fibers, applied voltage, flow rate, and tip-to-collection distance (TCD) were carefully optimized. In this study the polymer solution (HA, PVA) and vitamin B12-containing drugs in their respective solvents were electrospun under the applied electrical potential of 12 kv over a tip-to-collector distance of 18 cm and a flow rate of 200 ul/hr. Electrospinning of PVA yielded optimum fibers with an average diameter of 300–400 nm at 10% polymer concentration under the applied electric potential of 12 kv over a TCD of 18 cm. At low concentration of PVA, fiber density was very low, along with the formation of beads at regular intervals. As the concentration of PVA was increased, there was a gradual increase in the fiber density, with no droplet formation till a concentration of 10%, beyond which constant droplet formation was observed. The observation clearly indicated that with an increase in the PVA concentration, there is an increase in the electrical force exerted on the jet, which in turn increases the mass throughput but results in the formation of drops. However, 10% PVA provides optimum physical and electrical conditions under defined electrospinning conditions. It has been reported that HA alone in its aqueous solution cannot be electrospun. The major factor which hinders the process of electrospinning is its viscoelastic property. A literature survey suggests that HA can make smooth fibers when blended with certain excipients like PVA. In this study, PVA was used with HA, which modified the viscosity of HA; HA at very low concentrations (below 2%) was not sufficient to generate fibrous structures and resulted in formation of droplets, while HA beyond 2% became too viscous to form a jet. Thus, in the present study, the concentration of HA was fixed at 2% and a ratio of PVA (10%) was tried. It was observed that as the ratio of PVA was increased, there was a gradual increase in the fiber formation and the continuous jet was obtained. The optimum nanofibers were obtained at a ratio of 5.0/5.0 (HA/PVA) with fiber diameter of 200–350 nm under the applied electrical potential of 12 kv, and TCD of 18 cm at a flow rate of 200 ul/hour.
Drug loading in nanofiber mat
The major challenge in fabrication of a medicated nanofiber mat is the drug loading, as the physical and chemical properties of the drug vary considerably. In the present study, a passive drug loading technique has been used. Both polymers (HA/PVA) are found to be freely dispersed in water, whereas nicorandil is sparingly soluble in water but freely soluble in ethanol. Therefore, in the present study, in order to improve the drug payload, a saturated solution of drug in ethanol was prepared which was then mixed to the aqueous phases containing vitamin B12 and polymer mixtures, prior to electrospinning. Nonwoven, smooth, and discrete nanofibers were observed under the spinning parameters optimized for blank composite nanofiber. This method showed appreciable increase in drug loading, as the entrapment efficiency observed with this technique was 97%.
Fiber morphology
Electron micrographs of composite HA/PVA nanofiber under optimized spinning condition are shown in & . The electron photomicrograph illustrates that the optimized ratio of HA and PVA of 1/1 produced smooth, nonwoven, and discrete nanofibers with a fiber diameter range of 200–350 nm. The drug-loaded nanofiber mat demonstrated a slight increase in fiber diameter that could be attributed to increased solid content. Further, the addition of ethanol displaces water from the molecular site of the polymers and results in poor aspect ratio (length and diameter ratio), with a large fiber diameter. Further, the displacement of water from the polymeric network facilitates the evaporation of water under the specified spinning parameters resulting in instant aggregation of polymers, which leads to the formation of nanofibers with large diameter.
FTIR results
The IR spectra of nicorandil as well as those of their corresponding electrospun nanofibers are illustrated in & . The IR spectra were obtained and studied for any kind of interactions between their functional groups. The IR spectra of nicorandil showed a sharp peak at 3075 cm− 1 C–H (aromatic) stretching, 3243 cm− 1 N–H (amide) stretching, 1361 cm−1 NO2 stretching, 1590 C = C aromatic, and 1555 N–O asymmetric stretching. The observed peaks in the IR spectrum for drug-loaded nanofiber were found to be concordant with functional groups present in plain nicorandil. The IR spectra thus led to the inference that there is no interaction between drug and polymers in the drug-loaded composite nanofiber mat.
Differential scanning calorimetry (DSC)
The DSC thermograph of the drug () demonstrated the sharp melting point at 93°C, which indicates the crystalline nature of the drug. However, the endothermic peak at 92°C in drug-loaded nanofiber () indicated the transition in solid state of drug in the nanofiber mat. This could be related to the fabrication process, where a quick transformation of polymer solution into a solid state prevents the formation of stable crystal. Vrbata et al. reported that the higher solubility of diosmine in composite nanofiber (HPC, PVA and PEO) is related to its amorphous structure (CitationVrbata et al. 2014). Further, the wetting phenomenon of PVA helps to restore the drug properties in the polymer dispersion and eventually results a nano solid dispersion of drug in the developed composite nanofibrous mat.
X-ray diffraction (XRD)
The XRD result of plain drug and composite drug-loaded nanofiber is illustrated in & . XRD of drug-loaded nanofiber indicated a substantial broadening of XRD peaks at 2θ = 23.80 with a significant variation in peak intensity when compared to plain drug. Absences of intense peak with reduced peak intensity at the scanning angle of 23.080 clearly demonstrated phase transition of drug in the composite nanofiber. As described in an earlier section, the wetting phenomenon of PVA and the fabrication process largely contribute in the solid state transition of drug in the composite nanofiber. Li et al. absorbed higher solubility quercetin in ethyl cellulose nanofiber, which was related to its amorphous physical status in the nanofiber, and confirmed by X-ray diffraction study (CitationLi et al. 2013).
Drug release results
The result of the in vitro study, shown in , indicates a burst release of 28% after 30 min, which could be attributed to surface drug followed by a steady release over a period of 6 h. Controlled drug release is associated to viscoelastic behavior of the developed formulation. Moreover, the poor drug solubility in the biological fluid largely contributes to the controlled release behavior of the developed formulation, where the drug release follows a dissolution-mediated diffusion process, whereas the high intrinsic properties of the polymer matrix restrict the permeation of dissolution medium, which results in slow diffusion of entrapped drug (CitationYeo et al. 2010).
Drug permeability results
The permeability of drug from the composite nanofiber across the goat mucosa is represented in . The permeability study indicated that the drug concentration gradient plays an important role in the drug permeability across the membrane. Mucoadhesion of the developed system ensures the retention of the carrier system near its absorption window over a prolonged period, serving as a rate-limiting factor which determines permeability. Further, the wetting phenomenon and membrane-spanning property of PVA largely contribute in the permeability of drug from the developed composite nanofiber mat. The drug concentration was found to be 71% after 9 h, which indicates better drug permeability found in the composite nanofiber mat.
Mucoadhesive study
The results of mucoadhesive study demonstrate that there is a significant difference in the mucoadhesive detachment force between the film and nanofiber patch. This could be attributed to the surface area, where the nanofiber allows molecular entanglement of the carrier system with local mucosal lining. Singh et al. reported that the nanofiber, due to its unique functional features, exhibits excellent mucoadhesiveness to achieve a high residence time at the site of application (CitationSingh et al. 2014a). In addition, the nanoscale structure of the developed carrier system significantly decreases the contact angle and therefore facilitates the formation of a hydrogen bond. Further, there is no significant difference in mucoadhesive detachment force observed between drug-loaded nanofiber and plain nanofiber. However, a slight decrease in mucoadhesive force in drug-loaded nanofiber may be related to the surface of the drug, which results in the reduction of surface hydrophobicity and in turn ends with a poor mucoadhesive detachment force. Shows the mucoadhesive strength in gm/cm2 of composite nanofiber, drug-loaded composite nanofiber, and composite polymeric film developed using equal volume ratios similar to that present in optimized composite nanofiber.
Table I. Mucoadhesive strength of different formulations.
Tensile strength of nanofiber
A nanofiber having 8 cm length and 4 cm width was placed in a vertical position along the axis of the Brookfield texture analyzer with the help of clamps. This test is performed to find out how much force is required to break down the nanofiber and gives us an idea about the mechanical property of the nanofiber. The developed nanofiber patch showed good tensile strength, which revealed that the nanofiber possesses enough flexibility and can easily bear water flux. The nonwoven and aligned nanofiber scaffolds were successfully utilized for tendon repair because of the higher tensile properties and desired mechanical requirements (CitationDavis et al. 2009). The force recorded to break down the drug-loaded composite nanofiber was found to be 65 g.
Degree of swelling
Release of the drug from the nanofiber mat depends upon the degree of swelling, as it determines the release behavior of the drug from the nanofibrous mat. The graphic representation of the degree of swelling of blank and drug-loaded nano fibers at different time intervals is shown in . The swelling of unloaded nanofiber is found to be higher than that of drug-loaded nanofiber. This could be attributed to the higher surface area of plain nanofiber associated with smaller fiber diameter. Moreover, the partial congealing of the polymer in the drug-loaded nanofiber restricts the water permeability. This allows controlled release of drug from the polymeric nanofiber matrix. However, the swelling index of both polymeric nanofiber and drug-loaded nanofiber was decreased after 4 h, indicating the erosion of the polymer. The drug-loaded polymeric nanofiber shows slows erosion rate, which could be attributed to a net decrease in water flux in the presence of a hydrophobic drug in the drug-loaded composite nanofiber.
Results of pharmacokinetic studies
The pharmacokinetic study clearly demonstrates a comparable therapeutic efficacy of the developed formulation. The developed formulation demonstrated a longer t1/2 of 3.7 h over marketed formulations which possess a t1/2 of 0.99 h. This could be attributed to higher drug absorption and controlled release behavior of the developed formulation. Jakab and Hamori et al. observed a drastic change in pharmacokinetic profile for both water and insoluble drugs using the Eudragit 5100 nanofiber followed by intraduodenal administration (CitationJakab and Hamori 1988). The developed formulation exhibited a Cmax comparable with that of the marketed formulation, which clearly substantiated that the sublingual route with rich vasculature and thin epithelial layer serves as a potential route for drug absorption. Further, the unique functional features of the developed carrier system facilitate the drug absorption by retaining the carrier system over an extended period at the site of administration ().
Table II. Comparative pharmacokinetic parameters of the tested formulation.
Histopathology study
The results of the histopathology studies of different formulations are illustrated in . The histopathology study suggested that the developed nanofiber formulation shows no evidence of inflammation, whereas plain drug and marketed formulation demonstrated extensive tissue damage. This could be attributed to HA, because the presence of HA reduced the level of pro-inflammatory cytokines. Further, vitamin B12 maintains the integrity of the mucous membrane. This inhibits the chemotoxins and thus helps to minimize the mucous ulceration induced by nicorandil.
Conclusion
The composite nanofiber comprising of HA, PVA, and vitamin B12 can be successfully used to counter the adverse consequences associated with the oral administration of nicorandil. The histopathology study concluded that there is no evidence of mucosal ulceration following sublingual administration of nicorandil. The pharmacokinetic study demonstrated a therapeutic effectiveness of the developed formulation comparable with that of the marketed formulation. The present investigation thus gives an impetus for the developed formulation to be successfully utilized for the sublingual delivery of anti-anginal drugs.
Acknowledgements
The authors Dr. Goutam Rath and Dr. Amit K Goyal are thankful to the Department of Biotechnology (DBT), New Delhi (F.No. 102/IFD/SAN/2577/2011-2012 dated September 28, 2011) for providing financial assistance to carry out research.
Declaration of interest
The authors report no declarations of interest. The authors alone are responsible for the content and writing of the paper.
References
- Chaudhary S, Garg T, Murthy R, Rath G, Goyal AK. 2015a. Development, optimization and evaluation of long chain nanolipid carrier for hepatic delivery of silymarin through lymphatic transport pathway. Int J Pharm. 485:108–121.
- Chaudhary S, Garg T, Murthy RS, Rath G, Goyal AK. 2014. Recent approaches of lipid-based delivery system for lymphatic targeting via oral route. J Drug Target. 22:871–872.
- Chaudhary S, Garg T, Rath G, Murthy RR, Goyal AK. 2015b. Enhancing the bioavailability of mebendazole by integrating the principles solid dispersion and nanocrystal techniques, for safe and effective management of human echinococcosis. Artif Cells Nanomed Biotechnol. 1–6.
- Davis DA, Hamilton A, Yang J, Cremar LD, Van Gough D, Potisek SL, et al. 2009. Force-induced activation of covalent bonds in mechanoresponsive polymeric materials. Nature. 459:68–72.
- Frydman AM, Chapelle P, Diekmann H, Bruno R, Thebault JJ, Bouthier J, et al. 1989. Pharmacokinetics of nicorandil. Am J Cardiol. 63:J25–J33.
- Fuster V, Kelly B. 2010. Committee on preventing the global epidemic of cardiovascular disease: meeting the challenges in developing countries; institute of medicine. Promoting Cardiovascular Health in the Developing World: A Critical Challenge to Achieve Global Health. Washington (DC), US: National Academies Press.
- Gagandeep, Garg T, Malik B, Rath G, Goyal AK. 2014. Development and characterization of nano-fiber patch for the treatment of glaucoma. Eur J Pharm Sci. 53:10–6.
- Garg T. 2014. Current nanotechnological approaches for an effective delivery of bio-active drug molecules in the treatment of acne. Artif Cells Nanomed Biotechnol. 1–8.
- Garg T, Bhandari S, Rath G, Goyal AK. 2015a. Current strategies for targeted delivery of bio-active drug molecules in the treatment of brain tumor. J Drug Target. 1–23.
- Garg T, Goyal AK. 2012. Iontophoresis: Drug Delivery System by Applying an Electrical Potential Across the Skin. Drug Deliv Lett. 2:270–280.
- Garg T, Goyal AK. 2014a. Biomaterial-based scaffolds–current status and future directions. Expert Opin Drug Deliv. 11:767–89.
- Garg T, Goyal AK. 2014b. Liposomes: Targeted and Controlled Delivery System. Drug Deliv Lett. 4:62–71.
- Garg T, Goyal AK. 2014c. Medicated Chewing Gum: Patient Compliance Oral Drug Delivery System. Drug Deliv Lett. 4:72–78.
- Garg T, Goyal AK, Arora S, Murthy R. 2012a. Development, Optimization & Evaluation of Porous Chitosan Scaffold Formulation of Gliclazide for the Treatment of Type-2 Diabetes Mellitus. Drug Deliv Lett. 2:251–261.
- Garg T, Kumar A, Rath G, Goyal AK. 2014a. Gastroretentive drug delivery systems for therapeutic management of peptic ulcer. Crit Rev Ther Drug Carrier Syst. 31:531–57.
- Garg T, Rath G. 2015. Development, optimization and evaluation ofelectrospun nanofibers: tool fortargeted vaginal delivery of antimicrobials against urinary tract infections. Curr Drug Deliv.
- Garg T, Rath G, Goyal AK. 2014b. Ancient and advanced approaches for the treatment of an inflammatory autoimmune disease-psoriasis. Crit Rev Ther Drug Carrier Syst. 31:331–64.
- Garg T, Rath G, Goyal AK. 2014c. Biomaterials-based nanofiber scaffold: targeted and controlled carrier for cell and drug delivery. J Drug Target. 1–20.
- Garg T, Rath G, Goyal AK. 2014d. Comprehensive review on additives of topical dosage forms for drug delivery. Drug Deliv.
- Garg T, Rath G, Goyal AK. 2015b. Inhalable chitosan nanoparticles as antitubercular drug carriers for an effective treatment of tuberculosis. Artif Cells Nanomed Biotechnol. 1–5.
- Garg T, Singh O, Arora S, Murthy R. 2011a. Dendrimer—A novel scaffold for drug delivery. Int J Pharm Sci Rev Res. 7:211–220.
- Garg T, Singh O, Arora S, Murthy R. 2011b. Patented Microencapsulation Techniques And Its Application. J Pharm Res. 4:2097–2102.
- Garg T, Singh O, Arora S, Murthy R. 2012b. Scaffold: a novel carrier for cell and drug delivery. Crit Rev Ther Drug Carrier Syst. 29:1–63.
- Garg T, Singh S, Goyal AK. 2013. Stimuli-sensitive hydrogels: an excellent carrier for drug and cell delivery. Crit Rev Ther Drug Carrier Syst. 30:369–409.
- Goyal AK, Rath G, Garg T. 2013a. Nanotechnological Approaches for Genetic Immunization. In: Erdmann VA, Barciszewski hab, Eds. DNA and RNA Nanobiotechnologies in Medicine: Diagnosis and Treatment of Diseases. 67–120. Berlin Heidelberg: Springer-Verlag.
- Goyal G, Garg T, Malik B, Chauhan G, Rath G, Goyal AK. 2013b. Development and characterization of niosomal gel for topical delivery of benzoyl peroxide. Drug Deliv.
- Goyal G, Garg T, Rath G, Goyal AK. 2014a. Current nanotechnological strategies for an effective delivery of drugs in treatment of periodontal disease. Crit Rev Ther Drug Carrier Syst. 31:89–119.
- Goyal G, Garg T, Rath G, Goyal AK. 2014b. Current nanotechnological strategies for treating glaucoma. Crit Rev Ther Drug Carrier Syst. 31:365–405.
- Greiner A, Wendorff JH. 2007. Electrospinning: a fascinating method for the preparation of ultrathin fibers. Angew Chem Int Ed Engl. 46:5670–5703.
- Huang CC, Liao ZX, Chen DY, Hsiao CW, Chang Y, Sung HW. 2014. Injectable cell constructs fabricated via culture on a thermoresponsive methylcellulose hydrogel system for the treatment of ischemic diseases. Adv Healthc Mater. 3:1133–1148.
- Hussain T, Garg T, Goyal AK, Rath G. 2014. Biomedical applications of nanofiber scaffolds in tissue engineering. J Biomater Tissue Eng. 4:600–623.
- Jakab R, Hamori J. 1988. Quantitative morphology and synaptology of cerebellar glomeruli in the rat. Anat Embryol. 179:81–88.
- Johal HS, Garg T, Rath G, Goyal AK. 2014. Advanced topical drug delivery system for the management of vaginal candidiasis. Drug Deliv. 1–14.
- Joshi D, Garg T, Goyal AK, Rath G. 2014a. Advanced drug delivery approaches against periodontitis. Drug Deliv. 1–15.
- Joshi D, Garg T, Goyal AK, Rath G. 2014b. Development and characterization of novel medicated nanofibers against periodontitis. Curr Drug Deliv.
- Kalia V, Garg T, Rath G, Goyal AK. 2014. Development and evaluation of a sublingual film of the antiemetic granisetron hydrochloride. Artif Cells Nanomed Biotechnol. 1–5.
- Kataria K, Sharma A, Garg T, Goyal AK, Rath G. 2014. Novel technology to improve drug loading in polymeric nanofibers. Drug Deliv Lett. 4:79–86.
- Kaur G, Garg T, Rath G, Goyal AK. 2015a. Archaeosomes: an excellent carrier for drug and cell delivery. Drug Deliv. 1–16.
- Kaur M, Garg T, Narang RK. 2014a. A review of emerging trends in the treatment of tuberculosis. Artif Cells Nanomed Biotechnol. 1–7.
- Kaur M, Garg T, Rath G, Goyal AK. 2014b. Current nanotechnological strategies for effective delivery of bioactive drug molecules in the treatment of tuberculosis. Crit Rev Ther Drug Carrier Syst. 31:49–88.
- Kaur M, Malik B, Garg T, Rath G, Goyal AK. 2014c. Development and characterization of guar gum nanoparticles for oral immunization against tuberculosis. Drug Deliv.
- Kaur N, Garg T, Goyal AK, Rath G. 2014d. Formulation, optimization and evaluation of curcumin-beta-cyclodextrin-loaded sponge for effective drug delivery in thermal burns chemotherapy. Drug Deliv. 1–10.
- Kaur P, Garg T, Rath G, Goyal AK. 2015b. In situ nasal gel drug delivery: A novel approach for brain targeting through the mucosal membrane. Artif Cells Nanomed Biotechnol. 1–10.
- Kaur P, Garg T, Rath G, Murthy RS, Goyal AK. 2014e. Development, optimization and evaluation of surfactant-based pulmonary nanolipid carrier system of paclitaxel for the management of drug resistance lung cancer using Box-Behnken design. Drug Deliv. 1–14.
- Kaur P, Garg T, Rath G, Murthy RS, Goyal AK. 2014f. Surfactant-based drug delivery systems for treating drug-resistant lung cancer. Drug Deliv. 1–12.
- Kaur P, Garg T, Vaidya B, Prakash A, Rath G, Goyal AK. 2014g. Brain delivery of intranasal in situ gel of nanoparticulated polymeric carriers containing antidepressant drug: behavioral and biochemical assessment. J Drug Target. 1–12.
- Kaur R, Garg T, Das Gupta U, Gupta P, Rath G, Goyal AK. 2014h. Preparation and characterization of spray-dried inhalable powders containing nanoaggregates for pulmonary delivery of anti-tubercular drugs. Artif Cells Nanomed Biotechnol. 1–6.
- Kaur R, Garg T, Malik B, Gupta UD, Gupta P, Rath G, Goyal AK. 2014i. Development and characterization of spray-dried porous nanoaggregates for pulmonary delivery of anti-tubercular drugs. Drug Deliv. 1–6.
- Kaur R, Garg T, Rath G, Goyal AK. 2014j. Advanced aerosol delivery devices for potential cure of acute and chronic diseases. Crit Rev Ther Drug Carrier Syst. 31:495–530.
- Kaur V, Garg T, Rath G, Goyal AK. 2014k. Therapeutic potential of nanocarrier for overcoming to P-glycoprotein. J Drug Target. 1–12.
- Kumar A, Garg T, Sarma GS, Rath G, Goyal AK. 2015. Optimization of combinational intranasal drug delivery system for the management of migraine by using statistical design. Eur J Pharm Sci. 70C: 140–151.
- Lee CC, Mackay JA, Fréchet JM, Szoka FC. 2005. Designing dendrimers for biological applications. Nat Biotechnol. 23:1517–1526.
- Li X-Y, Li Y-C, Yu D-G, Liao Y-Z, Wang X. 2013. Fast disintegrating quercetin-Loaded drug delivery systems fabricated using coaxial electrospinning. Int J Mol Sci. 14:21647–21659.
- Malik R, Garg T, Goyal AK, Rath G. 2014. Polymeric nanofibers: targeted gastro-retentive drug delivery systems. J Drug Target. 1–16.
- Malik R, Garg T, Goyal AK, Rath G. 2015. Diacerein-Loaded novel gastroretentive nanofiber system using PLLA: Development and in vitro characterization. Artif Cells Nanomed Biotechnol. 1–9.
- Marwah H, Garg T, Goyal AK, Rath G. 2014. Permeation enhancer strategies in transdermal drug delivery. Drug Deliv. 1–15.
- Maton A. 1997. Human biology and health. 3rd ed. Prentice Hall. ISBN 10: 0134234359, ISBN 13: 9780134234359.
- Modgill V, Garg T, Goyal AK, Rath G. 2014a. Permeability study of ciprofloxacin from ultra-thin nanofibrous film through various mucosal membranes. Artif Cells Nanomed Biotechnol. 1–6.
- Modgill V, Garg T, Goyal AK, Rath G. 2014b. Transmucosal Delivery of Linagliptin for the Treatment of Type- 2 Diabetes Mellitus by Ultra-Thin Nanofibers. Curr Drug Deliv.
- Morie A, Garg T, Goyal AK, Rath G. 2014. Nanofibers as novel drug carrier - An overview. Artif Cells Nanomed Biotechnol. 1–9.
- Pabreja S, Garg T, Rath G, Goyal AK. 2014. Mucosal vaccination against tuberculosis using Ag85A-loaded immunostimulating complexes. Artif Cells Nanomed Biotechnol. 1–8.
- Ranganath SH, Wang C-H. 2008. Biodegradable microfiber implants delivering paclitaxel for post-surgical chemotherapy against malignant glioma. Biomaterials. 29:2996–3003.
- Rohilla R, Garg T, Bariwal J, Goyal AK, Rath G. 2014a. Development, optimization and characterization of glycyrrhetinic acid-chitosan nanoparticles of atorvastatin for liver targeting. Drug Deliv. 1–8.
- Rohilla R, Garg T, Goyal AK, Rath G. 2014b. Herbal and polymeric approaches for liver-targeting drug delivery: novel strategies and their significance. Drug Deliv. 1–17.
- Sharma A, Garg T, Aman A, Panchal K, Sharma R, Kumar S, Markandeywar T. 2014a. Nanogel-an advanced drug delivery tool: Current and future. Artif Cells Nanomed Biotechnol. 1–13.
- Sharma AK, Garg T, Goyal AK, Rath G. 2015. Role of microemuslsions in advanced drug delivery. Artif Cells Nanomed Biotechnol. 1–9.
- Sharma R, Garg T, Goyal AK, Rath G. 2014b. Development, optimization and evaluation of polymeric electrospun nanofiber: A tool for local delivery of fluconazole for management of vaginal candidiasis. Artif Cells Nanomed Biotechnol. 1–8.
- Sharma R, Singh H, Joshi M, Sharma A, Garg T, Goyal AK, Rath G. 2014c. Recent advances in polymeric electrospun nanofibers for drug delivery. Crit Rev Ther Drug Carrier Syst. 31:187–217.
- Singh B, Garg T, Goyal AK, Rath G. 2014a. Recent advancements in the cardiovascular drug carriers. Artif Cells Nanomed Biotechnol. 1–10.
- Singh H, Sharma R, Joshi M, Garg T, Goyal AK, Rath G. 2014b. Transmucosal delivery of Docetaxel by mucoadhesive polymeric nanofibers. Artif Cells Nanomed Biotechnol.
- Singh K, Arora N, Garg T. 2012. RFID: a trustable security tool in pharmaceutical industry. Am J Pharm Tech Res. 2:113–127.
- Singh O, Garg T, Rath G, Goyal AK. 2014c. Microbicides for the treatment of sexually transmitted HIV infections. Journal of Pharmaceutics. 1–18.
- Vrbata P, Berka P, Stránská D, Doležal P, Lázníček M. 2014. Electrospinning of diosmin from aqueous solutions for improved dissolution and oral absorption. Int J Pharm. 473:407–413.
- Wu Y, Yang W, Wang C, Hu J, Fu S. 2005. Chitosan nanoparticles as a novel delivery system for ammonium glycyrrhizinate. Int J Pharm. 295:235–245.
- Yeo M, Lee H, Kim G. 2010. Three-dimensional hierarchical composite scaffolds consisting of polycaprolactone, β-tricalcium phosphate, and collagen nanofibers: fabrication, physical properties, and in vitro cell activity for bone tissue regeneration. Biomacromolecules. 12:502–510.