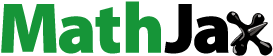
Abstract
Lung cancer is an invasive and progressive, fatal disease with few treatment choices and poor overall survival rates in nonsurgical stages. Leptin (LEP), an adipocyte derivative cytokine, participates in carcinogenesis. Increased amounts of systemic and pulmonary LEP indicate lung cancer. Curcumin (CUR) and silibinin (SIL) are herbal compounds which have many anticancer properties, but they have hydrophobic structures and low solubility in water. In this study, evaluated CUR–SIL dual drug-loaded poly (ɛ-caprolactone) [PCL]–co-poly ethylene glycol (PEG) magnetic nanoparticles (MNPs) were used to determine the inhibitory effect on LEP gene expression. The physicochemical properties of free and CUR–SIL-loaded PCL–PEG were fully characterized. The cytotoxic effect of CUR–SIL-loaded PCL–PEG magnetic nanoparticles was determined by MTT assay. Afterward, the inhibition of LEP gene expression was specified through real-time PCR. Results indicated that CUR–SIL cytotoxicity is time- and dose-dependent. CUR–SIL loaded MNPs showed the IC50 limit in lower concentrations in comparison to net CUR–SIL. CUR–SIL loaded MNPs reduced LEP expression more than net CUR–SIL. These results revealed the possibilities of CUR–SIL-loaded MNPs as a natural and effective antitumor drug delivery system to fight lung tumors.
Introduction
Bronchogenic carcinoma is the most prevalent type of cancer known, accounting for 1.37 million deaths annually (Siegel et al. Citation2014). Lung carcinoma sources the most cancer-related deaths in men and women (Anganeh et al. Citation2014, Chandolu and Dass Citation2013). Two basic types of lung tumor are small cell (SCLC) and non-SCLC (NSCLC) lung cancer (Badrzadeh et al. Citation2014). NSCLC accounts for at least 85% of all lung cancer cases, and lung adenocarcinoma is the most common type of NSCLC (Xiao et al. Citation2013). SCLC accounts for approximately 15% of new lung tumor cases detected annually (Badrzadeh et al. Citation2014). Determinations to characterize the structures of lung cancer by genetic and biochemical methods have increased the consideration of this disease and offered opportunities for the development of targeted therapies (Xiao et al. Citation2013).
The leptin (LEP) gene is located on chromosome 7q31.3 that bioactive protein, a 167-protein with a molecular mass of 16 kDa (Clément et al. Citation1998, Nejati-Koshki et al. Citation2013). First described as an adipocyte-derived hormone regulating energy metabolism and food intake, it is a pleiotropic hormone that plays both a proliferative and an anti-apoptotic role in several conditions, such as breast, gastric, and lung cancers (NSCLC) (Lai and Sun Citation2013). A complex interaction between cancer and immune inflammatory cells was presented by LEP, resulting in the development and progression of lung cancer (Clément et al. Citation1998). Previous studies have shown the expression of LEP in lung cancer (Lai and Sun Citation2013). LEP has been demonstrated to increase the proliferation, invasion, and migration of cancer cells and to contribute to the issue of VEGF (VanSaun Citation2013). LEP acts by binding to the extracellular domain of particular membrane receptors. LEP receptors are expressed in various tissues and cells, such as lung mucosal cells (Clément et al. Citation1998). Various polymorphisms of LEP and LEPR genes that are associated with lung cancer in humans have been identified (Clément et al. Citation1998). The binding of LEP to its receptor persuades the activation of different signaling pathways, including the JAK/STAT, MAPK, IRS, and SOCS3 signaling pathways, which facilitate its effects on cell proliferation and survival (Cirillo et al. Citation2008). There are several cure modalities for lung tumors, including chemotherapy, radiation, and surgery. Generally, early stage and localized lung tumors can be removed by surgical resection (Chandolu and Dass Citation2013). Recent treatment approaches are powerfully dependent on the type of malignancy and stage at the time of identification, but they often include a combination of surgery, chemotherapy, and/or radiation therapy (Babu et al. Citation2013). A first-line treatment choice for advanced-stage lung cancer is chemotherapy. It is often controlled intravenously where it circulates throughout the body, eventually locating and destroying tumorous and typical tissues (Babu et al. Citation2013).
Natural compounds derived from plant life have fewer side effects on the human body, such as neutropenia, thrombocytopenia, anemia, nausea, and hair loss, and are more effective in cancer chemotherapy (Nasiri et al. Citation2013, Song et al. Citation2014). Several natural agents such as turmeric and polyphenolic have attracted attention and have generally become a part of the human diet or traditional herbal medicines (Nasiri et al. Citation2013). CUR, a yellow dye the rhizome obtained from the turmeric rhizome curcuma longa, has many pharmacological applications such as potential chemo-preventive and chemotherapeutic activity by influencing diverse stages of carcinogenesis, including cell cycle arrest at all steps by increasing p53 and p21 expression. Furthermore, it inhibits tumor cell growth by stimulating the activation of casepase-3, casepase-7, and caspase-8 (Wu et al. Citation2010), differentiation and apoptosis in a series of tumors with low or no intrinsic toxicity (Mukerjee and Vishwanatha Citation2009, Rami and Zarghami Citation2013). CUR is a novel natural anticancer agent for NSCLC (Wu et al. Citation2010). It is a hydrophobic molecule and passes without problems through the plasma membrane into the cytosol (Pillai et al. Citation2004).
Another natural agent is SIL, a natural, polyphenolic flavonoid prepared from milk thistle. It is a vital requirement for any positive long-term cancer chemoprevention strategy and has anti-inflammatory, anti-tumor (including prostate, lung, colon, breast, hepatocellular carcinoma, and so on) properties. It decreases lung tumor expression of vascular endothelial growth factor (VEGF) and has anti-proliferative and anti-oxidant properties with little or no toxicity into tumor tissue (Rami and Zarghami Citation2013, Singh et al. Citation2006). Apparently, CUR and SLN intensify each other’s inhibitory effects on LEP and its receptor, possibly through similar mechanisms (Nasiri et al. Citation2013). Combination therapy including two to three drugs increases the therapeutic effectiveness and decreases the dosage of each separate drug essential to produce an observable therapeutic response (Babu et al. Citation2013).
Previous research has shown that the antitumor capability of CUR is restricted by multi-drug resistance (Moorthi and Kathiresan Citation2013). CUR has been used in combination with natural bioenhancers such as piperine, quercetin, and SIL to overcome these limitations (Moorthi and Kathiresan Citation2013). Previous studies have concluded that the CUR–SLN combination intensifies each other’s inhibitory effects on LEP gene expression (Moorthi et al. Citation2014, Nejati-Koshki et al. Citation2013). In addition, the hydrophobic nature of the majority of cancer chemotherapeutics (such as CUR and SLN) makes them poorly water solvable, thus limiting their administration at high doses (Liptay et al. Citation2002). Approaches to expand targeted delivery of chemotherapeutics that will result in increased drug efficacy with improved pharmacological properties and the least toxicity to normal tissues remain a priority in tumor treatment (Liptay et al. Citation2002). An enhanced delivery of CUR and SIL nanoformulations has been demonstrated in a biologically active form to different tumor cells (Anganeh et al. Citation2014, Ebrahimnezhad et al. Citation2013, Yallapu et al. Citation2013). The main problem in tumor therapy, however, is the absence of specificity of chemotherapeutic drugs towards the tumor cells (Yang et al. Citation2012). Drug target delivery into cancer cells has been improved by encapsulating medicines in nanoparticles with particular features (Song et al. Citation2014) and diverting the drug from non-target tissues to improve toxicity and increase effectiveness while reducing the incidence and severity of side effects (Jia et al. Citation2012).
Today, nanoparticles are individually useful because of their small size, large surface area, and in vivo drug delivery characteristics which are widely used in therapeutic study. Moreover, they have shown great potential in disease diagnosis (Torchilin Citation2009, Yang et al. Citation2012). Over recent decades, magnetic nanoparticles (MNPs) have been considered in drug delivery systems because of their magnetic resonance imaging contrast agents, biodegradability, biocompatibility, heating mediators in hyperthermia cancer therapy, and high delivery efficiency with nanovectors for targeted delivery of drugs/genes into malignant tissue, etc. The incorporation of super-paramagnetic properties (MNPs) into biocompatible carriers stimulates the targeting capability of nanoparticles into cancerous tissue through the assistance of a magnetic resonance field in addition to having EPR, thereby reducing the systemic distribution of cytotoxic compounds in vivo, enhancing uptake at the target site, and resulting in effective treatment at lower doses. Magnetic iron oxide particles with no surface modifications have hydrophobic surfaces with large surface area-to-volume ratios. This leads to the agglomeration of particles and the formation of large groups, causing increased particle size. Pre-coating or surface engineering of MNPs reduces aggregation and stabilizes the NP suspension in vitro. Both synthetic and natural polymers have been employed to modify MNP surfaces (Salehi et al. Citation2015). Furthermore, iron oxide nanoparticles (IOPs) are the only magnetic nanoparticles approved for clinical usage by the US Food and Drug Administration (FDA) (Kliman et al. Citation1986). Several biocompatible and/or biodegradable polymeric nanoparticles are used for drug delivery, e.g. nanogels, micelles, nanofibers, nanoparticles, etc. (Salehi et al. Citation2014). Polymeric nanoparticles have received a lot of attention for their stability and the ease with which their surfaces can be modified (Feng et al. Citation2012). Poly (ɛ-caprolactone) [PCL] is a biodegradable polyester which has attracted attention in organized drug delivery for its non-toxicity. However, the application of PCL as a drug delivery system has disadvantages: it has a slow degradation rate (in vitro and in vivo) due to its high crystallinity and hydrophobicity, and the biodegradability of PCL can be increased by copolymerization such as poly(ethylene glycol) [PEG] (Zhang and Misra Citation2007). PEG is a non-toxic water solvable and non-immunogenic polymer. It has the ability to escape protein adsorption and attack by the reticuloendothelial system (RES); accordingly, PCL nanoparticle uptake by the RES are effectively reduced (Danafar et al. Citation2014, Zhang and Misra Citation2007).
In this contribution, for the first time, the inhibitory effect of CUR–SLN-loaded PCL–PEG magnetic nanoparticles on LEP gene expression in A549 human lung cancer cell lines in vitro was evaluated.
Materials and methods
Combinations and reagents
PEG , stannous 2-ethylhexanoate (Sn(Oct)2) and ɛ-Caprolactone (ɛ-CL) were procured from Merc(Germany), Silibinin (SIL) [2,3-dihydro-3-[4-hydroxy-3-methoxyphenyl]-2-[hydroxymethyl]-6-[3,5,7-trihydroxy-4-oxobenzopyran-2-yl]benzodioxin], Curcumin (CUR), MTT [3-(4,5-Dimethylthiazol-2-yl)-2,5-diphenyltetrazolium bromide] were prepared from Sigma (St. Louis, MO) . Fetal bovine serum (Invitrogen, Carlsbad, CA), Phenol-red free RPMI 1640 with l-glutamine were procured from Gibco (Carlsbad, CA), Penicillin G and Streptomycin (Applichem, Darmstadt, Germany), TRIZOL Reagent (Invitrogen, USA), First-Strand Synthesis kit (Fermentas, Ontario, Canada), and Syber Green-I reagent were purchased from Takara (Tokyo, Japan).
Preparation of superparamagnetic nanoparticles
Superparamagnetic nanoparticles were arranged by an improved chemical precipitation method. According to this method 3.1736 g of FeCl2·4H2O (0.016 mol) and 7.5684 g of FeCl3·6H2O (0.028 mol) were dissolved in 280 mL of deionized water. The mixed solution was stirred under nitrogen flow at 80 °C for 1 h. Then, 40 mL NH3·H2O (30% w/v) was added into the combination rapidly, stirred under nitrogen for an hour, and then cooled to room temperature (RT). The precipitated particles were washed six times with hot water. Afterward separated by magnetic decantation (Kliman et al. Citation1986).
Synthesis of triblock copolymers of PCL–PEG–PCL (CEC)
The triblock copolymers of PCL–PEG–PCL were synthesized by ring-opening polymerization of ɛ-CL using PEG 2000 as an initiator and Sn(Oct)2[stannous 2-ethylhexanoate] as a catalytic agent. PEG was added to and heated at 120c under nitrogen atmosphere for 5 h to dry. The ɛ-CL and Sn(Oct)2 were added into the round-bottomed flask under a nitrogen atmosphere and then polymerization reaction was completed at 140 °C with stirring for 5 h (). Initially allowed to cool at RT, the combination was liquefied in methylene dichloride and solution was precipitated into a surplus amount of cold ethanol for purification then the combination was filtered and the refinement process was continued three times. Then dried in vacuum oven to a persistent weight and kept in a desiccator until use.
Characterization
Fourier Transforms Infrared spectroscopy
The chemical structures of the blank and drug loaded nanoparticles were studied by FTIR spectroscopy (Shimadzu 8400, Kyoto, Japan) using KBr discs.
1H-NMR spectroscopy
The chemical structures of the copolymers were determined by 1H-NMR (Bruker spectra spin 400 MHz, Leipzig, Germany).
Scanning electron microscopy studies
The surface morphology, average diameter and particle size of the polymeric nanoparticles were assessed by a Field Emission Scanning Electron Microscope-Energy Dispersive Using X-Ray (FESEM-EDX), S4160 Hitachi, Japan. The powder sample was spread on a SEM stub and sputtered with gold. Particle size was obtained by measuring the diameters of at least 300 particles shown in SEM using image analysis software (Image-Pro plus 4.5; Media Cybernetics, Silver Spring, Washington D.C.).
Magnetism test
Magnetic properties of the synthesized nanoparticles were assessed with a vibrating-sample magnetometer (VSM – 4 inch, Daghigh Meghnatis Kashan Co., Kashan, Iran) at RT (Chang et al. Citation2011, YesilbagáTonga Citation2013). A magnet (Φ 17.5 × 20 mm, 5500 Oe) was utilized for the collection of magnetic particles.
Dual CUR–SIL-loaded PCL–PEG MNPs
Dual anticancer loaded PCL–PEG MNPs were prepared by w/o/w double emulsion method. Firstly, 200 mg powder of PCL–PEG copolymer and CUR, SIL (2–3 mg/ml) were dissolved in Methylene chloride (as oil phase). The mixture was added to 5 mg Fe3O4 that was liquefied in distilled water (as aqueous phase). the mixture was transferred to falcon tube for homogenization (Edmund Buhler HO 4AP, 10,000 rpm, 3 × 10 s) ,while adding 15 mL polyvinyl alcohol (obtained second aqueous solution) homogenized for 3–5 min on ice bath (for 15 min with 6000 rpm). The final mixture was ultra-centrifuged (Beckman, optima, TLX , 24,000 g) and lyophilized (Christa Alpha, Bear Country: Boone (BBW Bear Shifter Paranormal Romance) USA) for better packing of drugs in the nanoparticles.
Prepared pure nanoparticles (10% weight of the polymer) as the blank without any drugs according that way for studying different effect of MNPs such as: toxicity, degradability and side effects like that on treated and untreated cells (Badrzadeh et al. Citation2014).
Determination of drug loading and entrapment efficiency of drugs
The CUR–SIL content in the drug-loaded MNPs was determined using the ultraviolet spectrophotometer at λmax of 430 nm for CUR and 287 nm for SIL.
The drug entrapment efficiency and drug-loading content were calculated based on the following formula (Akbarzadeh et al. Citation2012):
(1)
(1)
(2)
(2)
Cytotoxic effect of CUR–SIL loaded MNPs on lung cancer cell line
Cell culture
A549 cell was cultured in RPMI 1640 (Gibco, USA) supplemented with fetal bovine serum 10%, 2 mg/ml bicarbonate, 0/08 mg/ml streptomycin, 0/05 mg/ml penicillin G and incubated at 37 °C in a humidified atmosphere containing 5% CO2.
MTT assay
MTT assay was used to evaluate the cytotoxic effect of CUR–SIL on A549 cells, after 24, 48, and 72 h treatments in vitro. Cells in the exponential phase of growth were cultivated in a 96 well culture plate at a density of 1000 cells/200 μl PRMI in each well. Cells were exposed to free PCL–PEG MNPs; CUR–SIL loaded MNPs and free CUR–SIL. Cells were treated with different concentrations of CUR–SIL (10, 20, 40, 60, 80, and 100) μm/ml for 24, 48 and 72 h in triplicate. Then, the medium of all wells was replaced by 50 λ MTT (2 mg/ml) solution to each well and incubated for 4.5 h in the dark, followed by the addition of 200 λ DMSO. Then 25 μl Sorensen’s glycine buffer (0.1 M glycine, 0.1 M NaCl, pH = 10.5) was added and absorbance of each well was read at 575 nm in15–30 min.
RNA extraction
According to the manufacturer’s instructions, Total RNA was extracted by the use of the TRIZOL Reagent. (Invitrogen, USA)
The cells were mixed with 1 ml of TRIZOL reagent in a clean RNase-free tube and then incubated for 5 min at RT.
After pipetting, 200 λ chloroform was added, shaken carefully for 5 min, and incubated for another 2–3 min.
The combination was centrifuged at 12,000 g for 15 min. The aqueous phase was removed to a clean RNase-free tube and total RNA was precipitated by addition 1 ml isopropyl alcohol and incubated for 10 min at RT. The pellet including total RNA was washed using 75% ethanol and centrifuged at 7500 g for 5 min, the RNA pellet was dissolved in 40 λTE buffer or DEPC water (10 min in 55 °C). At the end the application, quantity of total RNA was calculated based on OD260/280 (>1/8) ratio measurement by Nano drop, quality was evaluated by agarose gel electrophoresis (AGE) on 1.3% agarose. It was valued accuracy of RNA (28 s, 18 s, and 5 s) (Nasiri et al. Citation2013).
Complementary DNA synthesis
The First Strand cDNA synthesis Kit applied for synthesis Complementary DNA (cDNA). The concentration of 10 and 20 (μm/ml) of RNA extracted was used to synthesis cDNA.
1μg total RNA, 1 μl Oligo (dT) primers and l2 μl DEPC treated water was mixed on dry ice and incubated at 65 °C for 5 min and then stored on dry ice for 1 min. Reaction buffer of the Ingredients (4 μl reaction Buffer 5X, 2 μl dNTP Mix 10 mM, 1 μl RiboLock RNase Inhibitor (20 u/μl)) was added , After that, RNA was reverse-transcribed using 1 μl RevertAid-M-MuLV RT (200 u/μl) and incubated at 25 °C for 5 min and again incubated at 42 °C for 60 min. Reaction was done the using heating at 70 °C for 5 min. The reverse transcription reaction product can be directly used in PCR applications or stored at −20 °C for less than 7 days and −70 °C is recommended for longer storage (Li et al. Citation2007).
Real-time PCR assay
The synthesized cDNA was diluted 1:10 of cDNA was used as template for RT PCR.
RNA Expression levels were evaluated by quantitative real-time RT-PCR using the SYBR Green-I dye (Takara, Japan) by the Rotor-GeneTM 6000 system (Corbett research, Sydney, Australia).
The quality of real-time PCR reactions (qRT-PCR) completed as follows; cycle modified for two phases; Initial denaturation at 95 °C for 10 min, followed by cycles of denaturation at 95 °C for 10 s, annealing at 60 °C for 30 s and extension at 60 °C for 30 s.
Forward (F) and Reverse (R) Primer Sequences of LEP and β-actin Used in Real-Time PCR were:
Forward (F) primer Sequences of LEP: (5TGTCACCAGGATCAATGACA3), Reverse (R) Primer Sequences of LEP: (5GTCCAAACCGGTGACTTTCT3), Forward (F) primer Sequences of β-actin: (5TGGACTTCGAGCAAGAGATG3) Reverse (R) Primer Sequences of β-actin: (5GAAGGAAGGCTGGAAGAGTG3).
Statistical analysis
Statistical analysis was completed with SPSS 17.0 (University of Washington, USA). The differences in expression levels of LEP genes between the control and treated cells were calculated using ΔΔCT. P-value <0.05 were considered statistically significant.
Results
Characterization of the structure of CUR–SIL–MNPs
PCL–PEG–PCL tri-block copolymer was synthesized by ring-opening polymerization of ɛ-caprolactone in existence of PEG, whose hydroxyl end group initiated the reaction (). The successful synthesization of copolymers was proved by 1HNMR and FTIR spectroscopy.
1HNMR spectrum of PCL–PEG–PCL co-polymer
shows the 1H-NMR spectra of PCL–PEG–PCL copolymer. Peaks at 1.42, 1.62, 2.34, and 4.09 ppm are assigned to methylene protons of – (CH2)3–, –OCCH2–, and –CH2OOC– in PCL units, respectively. The sharp single peak at 3.66 ppm is attributed to the methylene protons of homosequences of the PEG oxyethylene units. The very weak peak at 4.3 ppm is attributed to the methylene proton of PEG.
FTIR spectrum analysis
The chemical structures of the Fe3O4 (), CUR (), SIL (), PCL–PEG–PCL MNPs () and CUR-SIL loaded PCL–PEG–PCL MNPs () were studied by FTIR spectroscopy.
Figure 3. The FTIR spectra of (a) Fe3O4, (b) CUR, (c) SIL, (d) PCL–PEG–PCL, (e) CUR–SIL loaded PCL–PEG–PCL MNPs.
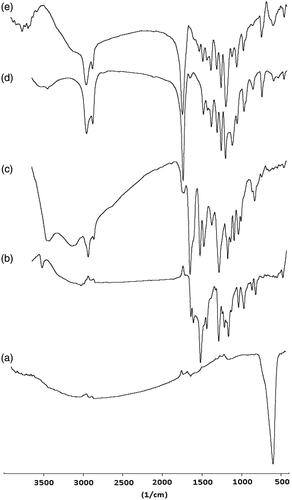
shows the IR spectra of Fe3O4. The observation bands at 572 cm−1 in the spectrum of Fe3O4 nanoparticles associated with the stretching vibration mode of the Fe–O bond. The peak at 3643 cm−1 is attributed to the stretching vibrations of OH, which is assigned to OH absorbed by Fe3O4 nanoparticles.
At CUR displayed the characteristic intensities of free hydroxyl group (O–H) at 3450 cm−1, the aromatic C=C str at 1590 and 1500 cm−1, C = O str at 1628 cm−1, aromatic C–O str at 1250 cm−1, aliphatic C–O str at 1180 cm−1 and =C–H bending vibrations at 980 and 900 cm−1. The bands at 1427 and 1316 cm−1 corresponding to the vibrational mode of C−O appeared at low frequency due to enolization and intermolecular H-bonding.
shows the IR spectra of SIL. The appearance of band in the region 3431 cm−1 is associated with H-bonded phenolic OH stretching at the expense of the free hydroxyl band. The C–H stretching vibration of the aliphatic section is manifested through the strong peak at 2854–2925 cm−1. A strong band at 1640 cm−1 is assigned to C=O stretch. Other principle peaks are at 1512 cm−1 and 1464 cm−1 for C=C ring stretching and 1364 cm−1 for symmetric bending vibration of CH3 group also the peak at 1273 cm−1 represents the stretching vibration of C–O–C while 1162 cm−1, 1082 cm−1, and 1028 cm−1 for in plane aromatic C–H and C–C bending and 823 cm−1 for out of plane =C–H bending and 640 cm−1 are the absorption bands of out of plane for C = C of benzene ring.
shows FT-IR spectra of PCL–PEG–PCL triblock copolymers. The absorption peak at 3438 cm−1 shows that there was a terminal hydroxyl group in the PCL–PEG–PCL and the absorption band at 1726 cm−1 was attributed to the C=O stretching vibrations of the ester carbonyl group. The peaks at 1105 and 1245 cm−1 were assigned to the characteristic C–O–C stretching vibration of repeated –OCH2CH2 units of PEG and the stretching vibrations of the –COO– bonds, respectively. All the C–H stretching bonds are centered at 2887 and 2945 cm−1.
represented the FT-IR spectra of CUR-SIL loaded PCL–PEG–PCL MNPs. The observation bands at 445 and 572 cm−1 in the spectrum proved the presence of Fe3O4. Signals at 3687–3887 cm−1 correspond to the characteristic absorption of the CUR, SIL, and stretching vibrations of OH, which is assigned to OH absorbed by Fe3O4 nanoparticles. The bands at 729, 842 and 959 cm−1 were attributed to the bending vibrations of the C–H bond of alkene groups in CUR and SIL. Peaks are at 1512 cm−1 and 1464 cm−1 were attributed to the aromatic C=C stretching of CUR and SIL. The absorption band at 1726 cm−1 was attributed to the C = O stretching vibrations of the ester carbonyl group in PCL–PEG–PCL. All data shows the accurate loading of CUR–SLN in PCL–PEG–PCL MNPs.
VSM test
The magnetic properties of the nanoparticles were analyzed by vibrating sample magnetometry (VSM) at RT. shows the hysteresis loops of the samples. The saturation magnetization was found to be 2.2 emu/g for Fe3O4–PCL–PEG–SIL–CUR, less than the pure Fe3O4 nanoparticles (30 emu/g). With the large saturation magnetization, the Fe3O4–PCL–PEG–SIL–CUR could be separated from the reaction medium rapidly and easily in a magnetic field. In addition, there was no hysteresis in the magnetization with both remanence and coercivity being zero, suggesting that these magnetic nanoparticles were super-paramagnetic. When the external magnetic field was removed, the magnetic nanoparticles could be well dispersed by gentle shaking. These magnetic properties were critical in the applications of the biomedical and bioengineering fields.
SEM micrographs
The SEM micrographs of Fe3O4 and PCL–PEG coated Fe3O4 nanoparticles are shown in . Observing the photograph (5a), the Fe3O4 nanoparticles were uniform spherical nanoparticles with mean diameter of about 15–25 nm. After coating of Fe3O4with PCL–PEG copolymer, the size of particles was changed to be 20–100 nm (5b) and lost its uniformity in morphology and aggregates were observed.
Drug loading and Encapsulation efficiency
Encapsulation efficiency was defined as the weight percentage of CUR–SIL incorporated into PCL–PEG–PCL MNPs. When the weight ratio of CUR–SIL to PCL–PEG–PCL MNPs was 1/20, the encapsulation efficiency was approximately 85% and loading content was 0.388 mg (DL% = 1.08%).
Cytotoxicity study of CUR–SIL-loaded PCL–PEG–PCL MNPs on A549 (in vitro)
Cytotoxicity of biomaterials was evaluated by MTT assay. Absorbance is an important linear relationship with cell numbers in MTT assay (). In this research to evaluate the cytotoxic effect of free CUR–SLN and CUR–SLN loaded PCL–PEG–PCL MNPs, A549 cell line was treated with different concentration (10–120 μM) of free CUR–SLN and CUR–SLN loaded PCL–PEG–PCL MNPs for 24, 48 and 72 h. The results indicated that the cytotoxicity of CUR–SLN loaded PCL–PEG–PCL MNPs on the A549 lung cancer cell line were time and dose dependent. IC50 value for net CUR–SLN and CUR–SLN loaded PCL–PEG–PCL MNPs after 24 h treatment with A549 cell lines were 59.7 ± 0.01 and 42.8 ± 0.01 μM, respectively (). After 48 h treatment IC50 value of net CUR–SLN (24.4 ± 0.03) () and especially CUR–SLN loaded PCL–PEG–PCL MNPs (9.5 ± 0.01 μM) decreased dramatically after 72 h. () PCL–PEG–PCL MNPs and methanol 1% showed an absorbance value equivalent of 97and 98% of control, respectively. It submits that PCL–PEG–PCL MNPs and methanol 1% have very low effect on the cells.
Quantitative real-time PCR (qPCR)
The levels of LEP genes expressions were evaluated by Real-Time PCR. Changes in LEP expressions level between the control and treated A549 cells were normalized to β-actin mRNA levels and then calculated by the 2-ΔΔct method. Real-time PCR results showed a significant decrease in LEP expression in the treated cells compared to the control cells (P-value < 0.05).
Real-time PCR data analysis indicated that by increasing amount of net CUR–SLN and CUR–SLN loaded PCL–PEG–PCL MNPs, LEP mRNA level expression would be decreased. CUR–SLN loaded PCL–PEG–PCL MNPs decreased LEP expression more than net CUR–SLN ().
Discussion
Currently, the development of new antitumor drug delivery systems is a main subject in cancer research. Targeted drug delivery is necessary for antitumor drug performance and to avoid side effects on usual cells (Anganeh et al. Citation2014). Nanoparticles can cross soft tissue and organ gaps and be taken up by cells, thereby improving potential applications in cancer treatment (Danafar et al. Citation2014). Recently, evidence has emerged that precise combinations of phytochemicals may be more effective in defending against cancer than isolated compounds (Nejati-Koshki et al. Citation2013). CUR (a biologically active component of turmeric) and SIL (a naturally occurring flavone) have been used as herbal medications for the treatment of inflammatory disorders, cancer, acquired immune deficiency syndrome, and other diseases for a long time. Problems with their use are that they are poorly soluble in water and simply degraded by the body. Therefore, CUR and SIL cannot be used through the intravenous path where they could possibly exert a maximal pharmacy-logical influence (Danafar et al. Citation2014). Results of the current study show that this thermo-sensitive magnetic nanoparticle has a high drug-loading capacity and that this system is promising for application in targeted smart anticancer drug delivery.
To improve their bioavailability and water solubility, different copolymers have been synthesized and evaluated. Danafar et al. synthesized CUR-loaded PCL–PEG–PCL triblock copolymers 74 nm in size and with 88 ± 3.32% encapsulation efficiency (Danafar et al. Citation2014). CUR-loaded PLGA–PEG was synthesized at a size of 55 ± 6 nm, and encapsulation efficiency was 84.5% (Anganeh et al. Citation2014). CUR-loaded NIPAAm-MAA nanoparticles with a size of 40–50 nm and drug-loading capacity (DL%) of 89.6% were produced (Li et al. Citation2007) along with other nanoparticles that were SIL-loaded PLGA–PEG–Fe3O4 with a size of 25–75 nm (Ebrahimnezhad et al. Citation2013).
The present study considered the in vitro behavior of CUR–SLN loaded magnetic nanoparticles modified with PCL–PEG–PCL MNPs for targeted and controlled drug delivery applications. FT-IR, 1H NMR, and SEM analyses specified CUR and SLN loading on PCL–PEG–FE3O4. CUR–SLN-loaded PCL–PEG–PCL MNPs were 20–100 nm in size.
The CUR–SLN loaded MNPs showed high loading content, and encapsulation efficiency was approximately 85%. In this research, different concentrations of CUR–SLN-loaded PCL–PEG–PCL MNPs were used in three different time intervals (24, 48, and 72 h) for MTT assay to determine their cytotoxic effect in the A549 cell line. In previous studies, values for the IC50 of SIL, CUR, and a combination of them of 110, 30, and 20 μM, respectively, were obtained (Nejati-Koshki et al. Citation2013). In this research, however, the IC50 values of CUR–SLN-loaded PCL–PEG–PCL MNPs were dramatically decreased after 24, 48, and 72 h of treatment on A549 cell lines (IC50 < 10 μM after 48 and 72 h).
MTT assay showed that CUR–SLN-loaded PCL–PEG–PCL MNPs inhibited LEP in a dose- and time-dependent manner.
The mitogenic, transforming, or migration-induced properties of LEP have been discovered in several cell types such as smooth muscle cells, normal and neoplastic colon cells, and normal and malignant mammary epithelial cells (Nejati-Koshki et al. Citation2013). It has been widely reported by previous studies that LEP expression increased in the bronchial mucosa of chronic obstructive pulmonary disease patients in association with airway inflammation and airflow obstruction; lung tumor is an inflammation-related disease. The production of immune-inflammatory cytokines was increased by LEP stimulation, such as VEGF, interleukin-6, and prostaglandin (PGE2), Furthermore, LEP stimulation activated the JAK/STAT3, PI3K/AKT, and MEK1/2 signaling paths, which contributed to the production of VEGF, IL-6, and PGE2.
LEP supports the immune escape of lung cancer by prompting pro-inflammatory cytokines and resistance to apoptosis (Shen et al. Citation2009). CUR and SLN as well as their combination inhibited the expressions of LEP in lung cancer via the induction of apoptosis (Pillai et al. Citation2004), and they caused a down-regulation of telomerase gene expression in cancer cells (Nejati-Koshki et al. Citation2013). Moreover, they inhibit lung tumor angiogenesis as a chemo-preventive agent for suppressing lung tumor progression (Singh et al. Citation2006, Yin et al. Citation2013).
In this study, for the first time, the effect of CUR–SLN-loaded PCL–PEG–PCL MNPs on LEP gene expression in the treatment of lung cancer was investigated. Results indicate that the combination of CUR–SLN-loaded PCL–PEG MNPs had a higher inhibitory effect on LEP gene expression than pure CUR–SLN on the A549 lung cancer cell line. This inhibition was dose- and time-dependent.
Conclusion
Nanomedicine is improving to offer new perspectives to manage hard-to-treat diseases. With today’s anticancer drug delivery systems, the critical goal of cancer healing has become to slay as many cancer cells as possible while leaving the healthy cells unaffected. In the current study, the triblock PCL–PEG–PCL MNPs successfully synthesized by the method of ring-opening polymerization and were used to encapsulate the dual anticancer agents CUR and SIL, which can be used to develop new and effective drug delivery systems to fight lung tumors. Cytotoxicity studies demonstrated that the PCL–PEG–PCL MNPs had no cytotoxicity and were biocompatible, while CUR–SLN-loaded MNPs enhanced their cytotoxicity in the A549 cell line in vitro. This study assessed CUR–SLN-loaded MNPs and net CUR–SLN on LEP gene expression in lung cancer cell lines with quantitative real-time PCR. Results showed that CUR–SLN-loaded MNPs can decrease the expression of LEP in the A549 cell line. Recent work has shown that CUR–SLN-loaded MNPs modified with PCL–PEG-PCL triblock copolymers have potent time- and dose-dependent antigrowth effects in an A549 cell line.
Acknowledgements
The authors would like to thank Medical Biotechnology, Faculty of Advanced Medical Sciences of Tabriz University of Medical Sciences for all the support provided.
Declaration of interest
The authors’ statement has no conflicts of interest in this article content.
References
- Akbarzadeh A, Mikaeili H, Zarghami N, Mohammad R, Barkhordari A, Davaran S. 2012. Preparation and in vitro evaluation of doxorubicin-loaded Fe3O4 magnetic nanoparticles modified with biocompatible copolymers. Int J Nanomed. 7:511.
- Anganeh MT, Mirakabad FST, Izadi M, Zeighamian V, Badrzadeh F, Salehi R, et al. 2014. The comparison between effects of free curcumin and curcumin loaded PLGA–PEG on telomerase and TRF1 expressions in calu-6 lung cancer cell line. Int J Biosci. 4:134–145.
- Babu A, Templeton AK, Munshi A, Ramesh R. 2013. Nanoparticle-based drug delivery for therapy of lung cancer: progress and challenges. J Nanomater. 2013:14.
- Badrzadeh F, Akbarzadeh A, Zarghami N, Yamchi MR, Zeighamian V, Tabatabae FS, et al. 2014. Comparison between effects of free curcumin and curcumin loaded NIPAAm-MAA nanoparticles on telomerase and PinX1 gene expression in lung cancer cells. Asian Pac J Cancer Prev. 15:8931–8936.
- Badrzadeh F, Rahmati-Yamchi M, Badrzadeh K, Valizadeh A, Zarghami N, Farkhani SM, et al. 2014. Drug delivery and nanodetection in lung cancer. Artif Cells Nanomed Biotechnol. 11:1–17.
- Chandolu V, Dass CR. 2013. Treatment of lung cancer using nanoparticle drug delivery systems. Curr Drug Discov Technol. 10:170–176.
- Chang B, Sha X, Guo J, Jiao Y, Wang C, Yang W. 2011. Thermo and pH dual responsive, polymer shell coated, magnetic mesoporous silica nanoparticles for controlled drug release. J Mater Chem. 21:9239–9247.
- Cirillo D, Rachiglio AM, la Montagna R, Giordano A, Normanno N. 2008. Leptin signaling in breast cancer: an overview. J Cell Biochem. 105:956–964.
- Clément K, Vaisse C, Lahlou N, Cabrol S, Pelloux V, Cassuto D, et al. 1998. A mutation in the human leptin receptor gene causes obesity and pituitary dysfunction. Nature. 392:398–401.
- Danafar H, Davaran S, Rostamizadeh K, Valizadeh H, Hamidi M. 2014. Biodegradable m-PEG/PCL core–shell micelles: preparation and characterization as a sustained release formulation for curcumin. Adv Pharm Bull. 4:501–510.
- Ebrahimnezhad Z, Zarghami N, Keyhani M, Amirsaadat S, Akbarzadeh A, Rahmati M, et al. 2013. Inhibition of hTERT gene expression by silibinin-loaded PLGA–PEG–Fe3O4 in T47D breast cancer cell line. Bioimpacts 3:67.
- Feng R, Song Z, Zhai G. 2012. Preparation and in vivo pharmacokinetics of curcumin-loaded PCL–PEG–PCL triblock copolymeric nanoparticles. Int J Nanomed. 7:4089.
- Jia Y, Yuan M, Yuan H, Huang X, Sui X, Cui X, et al. 2012. Co-encapsulation of magnetic Fe3O4 nanoparticles and doxorubicin into biodegradable PLGA nanocarriers for intratumoral drug delivery. Int J Nanomed. 7:1697.
- Kliman HJ, Nestler JE, Sermasi E, Sanger JM, Strauss III JF. 1986. Purification, characterization, and in vitro differentiation of cytotrophoblasts from human term placentae. Endocrinology. 118:1567–1582.
- Lai Q, Sun Y. 2013. Human leptin protein induces proliferation of A549 cells via inhibition of PKR-Like ER kinase and activating transcription factor-6 mediated apoptosis. Yonsei Med J. 54:1407–1415.
- Li L, Ahmed B, Mehta K, Kurzrock R. 2007. Liposomal curcumin with and without oxaliplatin: effects on cell growth, apoptosis, and angiogenesis in colorectal cancer. Mol Cancer Ther. 6:1276–1282.
- Liptay MJ, Grondin SC, Fry WA, Pozdol C, Carson D, Knop C, et al. 2002. Intraoperative sentinel lymph node mapping in non-small-cell lung cancer improves detection of micrometastases. J Clin Oncol. 20:1984–1988.
- Moorthi C, Kathiresan K. 2013. Curcumin–piperine/curcumin–quercetin/curcumin–silibinin dual drug-loaded nanoparticulate combination therapy: a novel approach to target and treat multidrug-resistant cancers. J Med Hypotheses Ideas. 7:15–20.
- Moorthi C, Kumar CS, Kathiresan K. 2014. Synergistic anti-cancer activity of curcumin and bio-enhancers combination against various cancer cell lines. Int J Pharm Pharm Sci. 6:901–903.
- Mukerjee A, Vishwanatha JK. 2009. Formulation, characterization and evaluation of curcumin-loaded PLGA nanospheres for cancer therapy. Anticancer Res. 29:3867–3875.
- Nasiri M, Zarghami N, Koshki KN, Mollazadeh M, Moghaddam MP, Rahmati M. 2013. Curcumin and silibinin inhibit telomerase expression in T47D human breast cancer cells. Asian Pac J Cancer Prev. 14:3449–3453.
- Nejati-Koshki K, Akbarzadeh A, Pourhasan-Moghadam M, Joo S. 2013. Inhibition of leptin and leptin receptor gene expression by silibinin–curcumin combination. Asian Pac J Cancer Prev. 14:6595–6599.
- Pillai GR, Srivastava AS, Hassanein TI, Chauhan DP, Carrier E. 2004. Induction of apoptosis in human lung cancer cells by curcumin. Cancer Lett. 208:163–170.
- Rami A, Zarghami N. 2013. Comparison of inhibitory effect of curcumin nanoparticles and free curcumin in human telomerase reverse transcriptase gene expression in breast cancer. Adv Pharm Bull. 3:127.
- Salehi R, Hamishehkar H, Eskandani M, Mahkam M, Davaran S. 2014. Development of dual responsive nanocomposite for simultaneous delivery of anticancer drugs. J Drug Target. 22:327–342.
- Salehi R, Rasouli S, Hamishehkar H. 2015. Smart thermo/pH responsive magnetic nanogels for the simultaneous delivery of doxorubicin and methotrexate. Int J Pharm. 487:274–284.
- Shen Y, Wang Q, Zhao Q, Zhou J. 2009. Leptin promotes the immune escape of lung cancer by inducing proinflammatory cytokines and resistance to apoptosis. Mol Med Rep. 2:295–299.
- Siegel R, Ma J, Zou Z, Jemal A. 2014. Cancer statistics, 2014. CA Cancer J Clin. 64:9–29.
- Singh RP, Deep G, Chittezhath M, Kaur M, Dwyer-Nield LD, Malkinson AM, et al. 2006. Effect of silibinin on the growth and progression of primary lung tumors in mice. J Natl Cancer Inst. 98:846–855.
- Song C-H, Liao J, Deng Z-H, Zhang J-Y, Xue H, Li Y-M, et al. 2014. Is leptin a predictive factor in patients with lung cancer? Clin Biochem. 47:230–232.
- Torchilin V. 2009. Multifunctional and stimuli-sensitive pharmaceutical nanocarriers. Eur J Pharm Biopharm. 71:431–444.
- VanSaun MN. 2013. Molecular pathways: adiponectin and leptin signaling in cancer. Clin Cancer Res. 19:1926–1932.
- Wu S-H, Hang L-W, Yang J-S, Chen H-Y, Lin H-Y, Chiang J-H, et al. 2010. Curcumin induces apoptosis in human non-small cell lung cancer NCI-H460 cells through ER stress and caspase cascade-and mitochondria-dependent pathways. Anticancer Res. 30:2125–2133.
- Xiao K, Jiang J, Guan C, Dong C, Wang G, Bai L, et al. 2013. Curcumin induces autophagy via activating the AMPK signaling pathway in lung adenocarcinoma cells. J Pharmacol Sci. 123:102–109.
- Yallapu MM, Ebeling MC, Khan S, Sundram V, Chauhan N, Gupta BK, et al. 2013. Novel curcumin-loaded magnetic nanoparticles for pancreatic cancer treatment. Mol Cancer Ther. 12:1471–1480.
- Yang H-W, Hua M-Y, Liu H-L, Huang C-Y, Wei K-C. 2012. Potential of magnetic nanoparticles for targeted drug delivery. Nanotechnol Sci Appl. 5:73.
- YesilbagáTonga G. 2013. Characterization of surface ligands on functionalized magnetic nanoparticles using laser desorption/ionization mass spectrometry (LDI-MS). Nanoscale. 5:5063–5066.
- Yin H, Zhang H, Liu B. 2013. Superior anticancer efficacy of curcumin-loaded nanoparticles against lung cancer. Acta Biochim Biophys Sin. 45:634–640.
- Zhang J, Misra R. 2007. Magnetic drug-targeting carrier encapsulated with thermosensitive smart polymer: core–shell nanoparticle carrier and drug release response. Acta Biomater. 3:838–850.