Abstract
In this study, we aimed to prepare the metal chelate affinity cryogels for the hemoglobin (Hb) depletion. Poly(2-hydroxyethyl methacrylate) (PHEMA) cryogels were selected as base matrix because of their blood compatibility, osmotic, chemical, and mechanical stability. Cryogels are also useful when working with the viscous samples such as blood, because of their interconnected macroporous structure. Iminodiacetic acid (IDA), the chelating agent, was covalently coupled with PHEMA cryogels after activation with the epichlorohydrin and then the Ni(II) ions were chelated to the IDA-bound cryogels. The depletion of the Hb from hemolysate was shown by SDS-PAGE.
Introduction
After the accomplishment of Human Genome Project, a new age has started in the era of life sciences; researches on proteins encoded by genes to further understand their biological functions increased exponentially. Proteomic technologies are applied to study function, organization, diversity, and dynamic state of a cell or whole tissue (Liumbruno et al. Citation2010, Mahn et al. Citation2010, Salgado et al. Citation2010, Uzun et al. Citation2013).
Erythrocytes (red blood cells) are the most abundant cells in vertebrates and they involve in a numerous processes by interacting with other red blood cells, endothelial cells, etc. Because of its important role in vertebrates, many researchers study them well. There are many diseases related with the red blood cells such as sickle cell anemia, malaria, type-2 diabetes, thalassemia, aplastic anemia, etc. (Bain et al. Citation1998). However, there are very few studies published on the proteomic studies of red blood cells (Baydemir et al. Citation2014, Derazshamshir et al. Citation2009, Liu et al. Citation2014).
As in the all proteomic studies of the blood plasma, high abundant proteins in erythrocytes, i.e., hemoglobin (Hb) and carbonic anhydrase, interfere with the analysis of low-abundant and disease-related proteins. Hb constitutes 95 % of the total cytosolic proteins of erythrocytes and masks observation and the detection of the low-abundant proteins in 2D gel electrophoresis. Thus, it is essential to remove these proteins prior to analyzing the cytosolic proteins of erythrocytes (Alvarez-Llamas et al. Citation2009, Baydemir et al. Citation2014, Bhattacharya et al. Citation2007, Goodman et al. Citation2007, Walpurgis et al. Citation2012). In the previous studies, metal chelate affinity chromatography has been investigated for its efficiency for Hb depletion and shown to be effective for increasing the number of detectable proteins (Akgol and Denizli Citation2004, Altıntaş et al. Citation2011, Bibi et al. Citation2013, Tetala et al. Citation2013).
Immobilized metal ion affinity chromatography (IMAC) is a widely used analytical and preparative separation method for many biomolecules (Akgol and Denizli Citation2004). It depends on the formation of stable metal chelate complexes with the metal ions and molecules bearing O, N, and S by ion–dipole interactions. Mostly used metal ions for this purpose are the transition metal ions such as Zn(II), Cu(II), Ni(II), Fe(II) and Co(II) incorporated with any metal chelating compound to the polymer matrix (Akgol and Denizli Citation2004, Bereli et al. Citation2008, Çimen and Denizli Citation2012).
Cryogels are supermacroporous gels, which can be prepared at subzero temperatures by the cryotropic gelation technique (Andac and Denizli Citation2014).
The solvent (water in this case) is frozen at this temperature and polymerization continues in non-frozen liquid phase. After completion of cryo-polymerization, melting ice crystals at room temperature form interconnected pores, which allows flow of mobile phase with minimal flow resistance. Macropore size within cryogels differs from hundreds of micrometers to only a few micrometers. Cryogels have also micropores that are formed in polymeric phase. The large pores offer advantages when working with viscous media such as blood, plasma, and plant or animal tissue extracts. They provide low-pressure drop, short diffusion path, and very short residence time for adsorption and elution (Baydemir and Denizli Citation2014, Percin et al. Citation2013).
In this study, poly(2-hydroxyethyl methacrylate) (PHEMA) cryogels were prepared and metal chelating agent iminodiacetic acid (IDA) was covalently coupled with the cryogels. Then, Ni(II) ion was investigated for its performance to remove Hb from red blood cell hemolysate.
Experimental
Materials
Hydroxyethyl methacrylate (HEMA), ammonium persulfate (APS), methylene bisacrylamide (MBA), and N,N,N′,N′-tetramethylene diamine (TEMED), Hb from bovine (with purity of 95–100%) were obtained from Sigma, St. Louis, MO (H-3760). Human plasma was also obtained from Sigma (Cat No.: P4639). All other chemicals were obtained from Merck AG (Darmstadt, Germany). Water used in the experiments was purified.
Preparation of PHEMA cryogels
Bulk polymerization technique was used to synthesize monolithic PHEMA cryogels as follows: 283 mg of MBA was dissolved in 15 mL deionized water and 1.3 mL of HEMA was added. The cryogelation was initiated in an ice bath by TEMED/APS [10% (w/v)] initiator pairs. The mixture was stirred for short time and then poured into plastic syringes (5 mL, id. 1.0 cm). After the mixtures in the syringes were frozen at −12 °C for 24 h, they were defrosted at room temperature. All cryogels obtained were washed in water and stored at +4 °C until further use.
IDA-attached PHEMA (PHEMA-IDA) cryogel
PHEMA cryogel was first activated with epichlorohydrin. For this, a solution of 18.5 mL epichlorohydrin, 172.5 mL NaOH (2 M), and 637 mg sodium borohydride passed through the cryogel column overnight at room temperature and then washed successively with water and 0.1 M acetic acid, respectively. In order to prepare the IMAC support material, IDA was used as a chelating agent. IDA was covalently attached to the cryogel in a continuous system as follows: 30 mL of 50 mg/mL of IDA solution was prepared in 2.0 M Na2CO3 at pH 11, using peristalting pump at 70 °C for 12 h. After immobilization reaction, IDA functionalized cryogels were washed with acetic acid (5%) and distilled water to remove the unreacted IDA.
Chelation of Ni(II)
Chelation of Ni(II) ion with IDA-attached PHEMA cryogels was prepared as follows: Ni(II) ions were prepared in different concentrations (10, 25, 50, and 100 ppm in 10 mM HEPES buffer solution), separately, and passed through the IDA-attached PHEMA cryogels in a continuous system for 2 h. Nitrate salts were used for the preparation of standard Ni(II) ion solutions. The Ni(II) concentration in the resulting solutions was determined with a graphite furnace atomic absorption spectrometer (AA800, Perkin Elmer, Bodenseewerk, Germany). The amounts of adsorbed were calculated by using the concentrations of the metal ions both in the initial and equilibrium solutions.
Metal leakage from the PHEMA cryogels was investigated with media pH (5.0–8.0), and also in a medium containing 1.0 M NaCl. It should also be noted that immobilized Ni(II)-containing cryogels were stored at 4 °C in the 0.1 M phosphate buffer (pH 7.4) with 0.02% sodium azide to prevent microbial contamination.
Characterization of PHEMA cryogels
The dried cryogel sample was weighed and then put into deionized water for 2 h. After the water absorption of the cryogel reached equilibrium, it weighed again and recorded. The amount of the water sorption of the cryogel sample was calculated as
where Wo and Ws are the weights of cryogel before and after uptake of water, respectively.
The cross-sectional surface morphology of the PHEMA-IDA-Ni(II) cryogel column was monitored using scanning electron microscopy (SEM) (JEOL JSM 5600 scanning electron microscope, Tokyo, Japan).
The specific surface area of cryogel was measured using Brunauer–Emmett–Teller (BET) model by multipoint analysis (Flowsorb II 2300 from Micromeritics Instrument Corporation, Norcross, GA).
FTIR spectra of dried and grinded PHEMA, PHEMA–IDA, and PHEMA–IDA–Ni(II) cryogel samples were obtained by using an FTIR spectrophotometer (FTIR 8000 Series, Shimadzu, Japan).
Blood compatibility studies
For the determination of the blood clotting time (CT), activated partial thromboplastin time (APTT), and prothrombin time (PT), PHEMA and PHEMA–IDA–Ni(II) cryogel columns were incubated with phosphate buffer solution (0.1 M. pH 7.4) for 24 h, at room temperature and then washed with 0.5 M NaCl solution and deionized water, respectively. Prior to use, all the samples and the reagents were preheated to 37.5 °C for 2 min. The 250 mg of dried and powdered cryogel sample was mixed with 0.1 mL of human plasma. All experiments were measured using semi-automatic blood coagulation analyzer (Tokra Medikal, Ankara, Turkey). The control samples were prepared using 50 mL of PBS and all measurements were repeated three times and the average of the results was reported (Baydemir and Denizli Citation2014, Yıldırım et al. Citation2013).
Adsorption–desorption studies
Adsorption of Hb from aqueous solutions
The Hb adsorption studies were carried out in a recirculation system. At the beginning of the each cycle, the cryogel column was prepared for the experiment by washing with deionized water and equilibrating with 0.1 M phosphate buffer (pH 7.4) for 30 min. Then, the Hb solution was pumped through the column under recirculation for 2 h. Effects of Hb concentration (varied between 0.01 and 0.08 mg/mL), flow rate (varied between 0.5 and 5 mL/min), pH (varied between 5.0 and 8.0), and ionic strength (varied between 0.01 and 1 M) on the adsorption amount were studied. The effect of Hb concentration onto maximum Hb adsorption amount was also performed for PHEMA plain cryogel column to investigate non-specific Hb adsorption amount onto PHEMA cryogel without Hb-specific IDA–Cu ligand. The Hb amounts in working solutions were monitored by spectrophotometer at 406 nm (UV-1601, Shimadzu, Japan).
The amount of adsorbed Hb was calculated using the following mass balance:
where q is the amount of adsorbed Hb (mg/g); Co and C are the initial and final concentrations of Hb, respectively (mg/mL); V is the volume of the solution (mL); m is the mass of the PHEMA cryogel (g). Each experiment was performed thrice and the average values were used.
Desorption and repeated use
The adsorbed Hb, desorbed from PHEMA–IDA–Ni(II) cryogel, was studied in continuous experimental setup using 0.1 M phosphate buffer (pH 8, include 0.5 M urea, 1 M NaCl) for 2.0 h, at room temperature. The Hb concentration in desorption solution was determined by spectrophotometer at 406 nm. The desorption ratio was calculated using the equation below and the reusability studies were performed by repeating adsorption–desorption cycle for 10 times using the same cryogel under the same conditions.
Hb depletion from human blood
Depletion of Hb from human blood was adapted from the following procedure (Derazshamshir et al. Citation2009). 10 mL of hemolysate solution was re-circulated through the PHEMA–IDA–Ni(II) cryogel for 2.0 h with 1.0 mL/min of flow rate at 20 °C. The amount of adsorbed Hb from hemolysate solution was measured by photometric method at Mesa Hospital (Ankara, Turkey). Sodium dodecyl sulfate polyacrylamide gel electrophoresis (SDS-PAGE) was used for imaging Hb depletion. Wide-range molecular weight marker (205–36.5 kDa, Wide Range Sigma Marker, Sigma) and 50 mg of samples were applied on 5–12% stacking solution. The gel was stained with colloidal Coomassie Blue method and monitored by ImageQuant 300 (Amersham, Piscataway, NJ).
FPLC studies
FPLC studies were performed using an AKTA–FPLC (Amersham Bioscience, Uppsala, Sweden) system equipped with a GE Healthcare column (10/10, 19-5001-01) that packed with PHEMA–IDA–Ni(II) cryogel column. Mobile phases A and B were prepared using 0.1 M phosphate buffers at pH 7.4 and at pH 8, include 0.5 M urea and 1 M sodium chloride, respectively. A linear gradient was applied at 1.0 mL/min flow rate (starting period: 100% of mobile phase A for 5 min; linear gradient: 0–100% B in 3.0 min, 100% eluent B in 7 min; 100% buffer A in last 10 min). All buffers and protein solutions were filtered before use. First of all the calibration studies were performed by applying the 1 mL of standard Hb solution to PHEMA–IDA–Ni(II) cryogel column, which was attached FPLC. Then the protein separation was performed at by applying 1 mL of hemolysate solution (1/6, diluted v/v) to FPLC column at room temperature and the absorbance was recorded at 280 nm. The void volume of the column was determined using KBr marker.
Capacity factor; k' = (tR − to)/to, ]tR: retention time of the protein; to: retention time of the void marker (KBr)], separation factor; α = k2′/k1′ (k2′: the capacity factor for Hb; k1′: capacity factor for other proteins), Resolution; Rs = 2 (tR,2 − tR,1)/(w2 + w1), (tR,1, tR,2: retention times of two adjacent peaks; w1, w2: widths of the two adjacent peaks at the baseline) and the total theoretical plate numbers N = 5.54 (tR/w0.5)2 (w0.5: peak width at the corresponding peak height fraction) were calculated to investigate the separation specifications of the PHEMA–IDA–Ni(II) cryogel column (Baydemir et al. Citation2014).
Results and discussion
Preparation of PHEMA–IDA–Me cryogels
For the preparation of PHEMA–IDA–Ni(II) cryogels, Ni(II) ions were used with different amounts of IDA attachment. As seen from , the highest metal loading onto PHEMA–IDA (IDA loading amount: 8.27) cryogel was obtained as 57.0 mg Ni(II) ion per gram PHEMA–IDA cryogel. Therefore, the following studies were implemented for PHEMA–IDA–Ni(II) cryogel at maximum Ni(II) loading amount.
Characterization studies
The newly synthesized PHEMA–IDA–Ni(II) cryogels have hydrophilic structures that they are water swollen; cross-linked polymeric structures. A pieces of dry, hydrophilic cross-linked PHEMA–IDA–Ni(II) cryogels was placed in water and then it absorbed the water molecules until reaching the equilibrium swollen state. At the equilibrium swollen state, water uptake ratio was found as 93% for PHEMA–IDA–Ni(II) cryogel.
The surface morphology of PHEMA–IDA–Ni(II) cryogel was investigated using scanning electron micrographs. The polymeric cryogel network constructed by macropores, which were formed in the freezing step of water molecules during the polymerization procedure. The presence of this interconnected supermacroporous structure within the cryogel morphology can be clearly seen in SEM photographs (). Cryogels gained low diffusional resistance of Hb molecules and facilitate mass transfer owing to interconnected macropores (10–200 μm in diameter). Furthermore, interconnected macropores enable to studying with high viscous samples blood (Arrua and Alvarez Igarzabal Citation2011).
Figure 2. (a) SEM photographs of PHEMA cryogels with different magnifications; (a-1) × 500, (a-2) × 1000; (b). The FTIR spectra of PHEMA (top side), PHEMA–IDA (middle side), and PHEMA–IDA–Ni(II) (bottom side) cryogels.
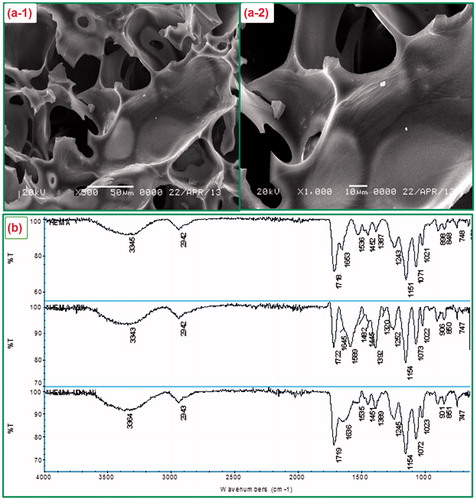
The physicochemical properties of PHEMA–IDA–Ni(II) are summarized in . The surface area of the cryogels was found as 24.7 m2/g by BET method. The interconnected bulk structure of cryogel provides low backpressures; however, it gives them lower surface areas compared with the other chromatographic supports. Eventually, lower surface area gives rise to small amounts of immobilized ligand and low sample capacities for affinity separations applications (Noir et al. Citation2007, Yao et al. Citation2007).
Table 1. (a) Physicochemical properties of the PHEMA cryogel; (b) Coagulation times of human plasma (reported in second)*; (c) Langmuir and Freundlich adsorption constants and correlation coefficients for PHEMA–IDA–Ni(II) cryogel.
As seen from , the characteristic peaks of PHEMA were observed at 3345 (O–H stretching), 2942 (C–H aliphatic), 1718 (C = O stretching), 1653-1536-1387 (amide I, amide II, amide III bands in MBA), and 1151 (C–O stretching) cm−1. The N–H stretching vibrations of PHEMA after epichlorohydrin activation and IDA attachment evidently increased in terms of intensity in the amide range of PHEMA–IDA spectrums. The amide I, amide II, and amide III stretchings at 1645, 1589, and 1392 cm−1 found in the FTIR spectrum of PHEMA–IDA showed a right shift with a frequency range of 1636, 1535, and 1389 cm−1 in the spectrum of PHEMA–IDA–Ni(II) due to the formation of N–Ni coordination. The right shift in following peak at 1245 cm−1 for the C–O stretching vibration in PHEMA–IDA spectrum also supports the O–Ni coordination in PHEMA–IDA–Ni(II) structure. It should be noted that no new peak was observed in the range of 4000–600 cm−1.
Blood compatibility studies
Biomaterials can be described as any materials interacting with living systems for use in medical devices or in particular applications for biological systems. All biomaterials have to meet the certain criteria prior to use for living systems. Depending on desired end use the blood compatibility, tissue compatibility, carcinogenicity, toxicity, and mechanical stability tests must be performed using developed biomaterials (Ergün et al. Citation2012). If a material contact with blood, first the small molecules are floated on surface (i.e., water, ions); this event is followed by adsorption of plasma proteins onto surface. The amount and the type of protein affect the coagulation mechanisms deeply. In order to estimate the blood compatibility of PHEMA and PHEMA–IDA–Ni(II) cryogels, in vitro coagulation times (CT), APTT that used for the bioactivity of intrinsic blood coagulation factors and PT, which was studied to estimate the extrinsic blood coagulation factors on surface of synthesized cryogels tests, were carried out. summarizes the CT, APTT, and PT test data. It is obvious that all the coagulation times for both of the PHEMA and PHEMA–IDA–Ni(II) cryogels were decreased when compared with control plasma. Due to the fact that these decreases can be tolerable by the body, it can be concluded that the blood compatibility of synthesized cryogels were good and the obtained results are nearly the same with the related literature (Denizli Citation1999,Citation2002, Denizli and Pişkin Citation1995).
Adsorption of Hb from aqueous solutions
Protein adsorption onto specific adsorbents is pH dependent. Hb adsorption studies were carried out using 0.1 M phosphate buffer in its buffering ranges. shows Hb adsorption capacity in phosphate buffer at different pH values. In these pH ranges, maximum Hb adsorption amount was observed at pH 7.4 as 4.15 mg Hb/g PHEMA–IDA–Ni(II) cryogels. Maximum adsorption capacity was obtained lower in other pH regions.
Figure 3. (a) Effect of pH on Hb adsorption amount. 0.1 M phosphate buffer (pH 7.4), flow rate: 0.5 mL/min, CHb: 0.05 mg/mL, mdry cryogel: 0.41 g. T: 20 °C; (b) Effect of flow rate on Hb adsorption onto cryogels. 0.1 M phosphate buffer (pH 7.4), T: 20 °C, flow rate: 0.5 mL/min, CHb: 0.05 mg/mL, mdry cryogel: 0.41 g; (c) Effect of ionic strength on Hb adsorption amount; 0.1 M phosphate buffer (pH 7.4), flow rate:0.5 mL/min, 0.05 mg/mL, mdry cryogel: 0.41 g, t: 2 h, T: 20 °C; (d) Adsorption–desorption cycle showing the reusability potential of a column. 0.1 M phosphate buffer (pH 7.4), flow rate: 0.5 mL/min, CHb: 0.05 mg/mL, mdry cryogel: 0.41 g, t: 2 h, T: 20 °C.
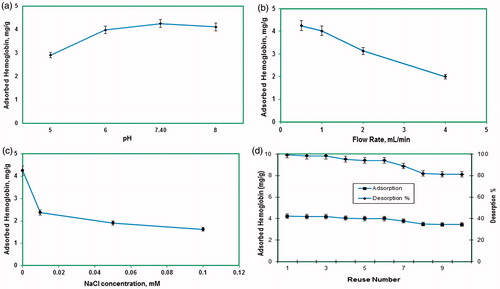
The Hb adsorption amount onto the PHEMA–IDA–Ni(II) cryogels was determined using 0.1 M pH 7.4 phosphate buffer at different flow rates. As estimated, the Hb adsorption amount of the cryogels was decreased by increasing flow rate from 0.5 to 4.0 mL/min. At lower flow rates, Hb molecules can diffuse slowly into the macrospores and took more time to interact with the specific binding sites in the inner parts of the cryogel. As a consequence of that, the best adsorption amount was obtained at low flow rate of 0.5 mL/min for all of the synthesized cryogel columns ().
The effect of ionic strength on Hb adsorption amount onto PHEMA–IDA–Ni(II) was investigated using NaCl salt in equilibrium Hb solutions. shows that the maximum Hb adsorption amount was decreased for all PHEMA–IDA–Ni(II) cryogel columns, by increasing NaCl concentration in initial Hb samples. For PHEMA–IDA–Ni(II), this decrease was recorded from 4.15 to 1.72 mg/g. As known well, the ionic interactions have an important role for the recognition and binding processes. For the Hb–metal ion interactions, the NaCl’s counter ions interact with the Hb molecules via charge–charge interactions and mask the binding sites (Derazshamshir et al. Citation2009). As a result of this behavior, the interaction between the Hb molecules with the binding metal sites was weaken and naturally the Hb adsorption amount was decreased.
Hb adsorption amount onto PHEMA–IDA–Ni(II) cryogel columns were investigated by increasing Hb concentration in buffer solution. The Hb adsorption amount increased; by increasing equilibrium Hb concentration in initial samples and at 0.05 mg/mL equilibrium Hb concentration all binding sites were occupied by Hb molecules and saturation was occurred. At higher Hb concentrations no more specific interaction can be observed between Hb molecules and metal ions, due to the saturation of the binding sites. The maximum Hb adsorption capacity was investigated for all metal ion ligands but the higher Hb adsorption capacity was obtained for PHEMA–IDA–Ni(II) column and it was found as 4.25 mg/g polymer on the average. According to the control experiments, the plain PHEMA cryogel column adsorbed 0.46 mg/g Hb, which shows that the non-specific interaction between PHEMA and Hb can be neglected ().
Figure 4. Adsorption behavior of Hb onto PHEMA–IDA–Ni(II) cryogel and modeling by Langmuir and Freundlich adsorption isotherms. Phosphate buffer (pH 7.4, 0, 1 M), flow rate: 0.5 mL/min, mdry cryogel: 0.41 g, t: 2 h, T: 20 °C.
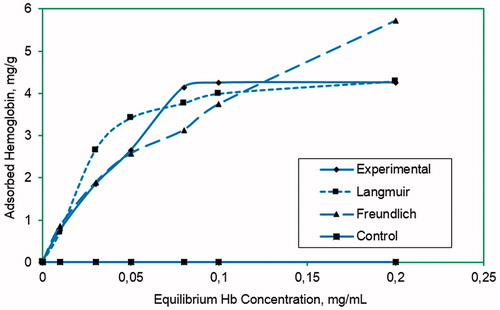
Adsorption behavior of continuous system was evaluated by calculating Langmuir and Freundlich adsorption isotherms given by following equation. For the adsorption system obtained in this study, Langmuir adsorption system can be applied because of the linear plot obtained from equilibrium data for Hb. Langmuir adsorption model can be formulized as given below:where Q stands for the amount of Hb (mg/g) adsorbed, Ceq stands for equilibrium concentration (mg/mL) of Hb, b stands for Langmuir constant (mL/mg), and Qmax stands for the maximum adsorption capacity (mg/g) of cryogels. Linear form of the equation in above can be given as
From the evaluation of data obtained from the experiments in this study, maximum adsorption capacity (Qmax) of cryogels for Hb was estimated. The calculated correlation coefficient (R2) for this adsorption system was 0.99 at pH 7.4. For the affinity system in this study, the Langmuir adsorption system is quite applicable. From the data obtained, theoretical adsorption capacity (Qmax) and the Langmuir adsorption constants were calculated as 4.44 and 17.31 mg/g at pH 7.4, respectively.
The Freundlich adsorption model is another adsorption system commonly used for the estimation of adsorption behavior. This adsorption model is used for especially heterogeneous surface as compared with the Langmuir model, which is for homogenous adsorption surface. According to this model, adsorption process should be reversible and completed on multilayer. Freundlich adsorption model can be formulized as given below:
where KF and n are Freundlich constants. Linear form of the raw equation can be given as
The intercept and the slope of the plot obtained for lnCeq versus lnQeq are lnKF and ‘n’, respectively.
The adsorption behavior of PHEMA cryogels can be explained best by Langmuir adsorption model because of higher correlation coefficient and so thus higher linearity. Langmuir and Freundlich adsorption model constants, n and KF, and the correlation coefficients are given in .
Table 2. Chromatographic separation data from human red blood cell hemolysate.
SDS-PAGE analysis of red blood cell fractions
Depletion of Hb from human blood with PHEMA–IDA–Ni(II) cryogel was visualized by SDS-PAGE ().
Figure 5. SDS-PAGE visualization for Hb depletion from human hemolysate. 5–12% SDS-PAGE, Lane 1: Hb standard, Lane 2: wide-range Sigma marker, Lane 3: crude sample of hemolysate, Lane 4: treated hemolysate with PHEMA–IDA–Ni(II) cryogel, Lane 5: Hb elution from the cryogel column.
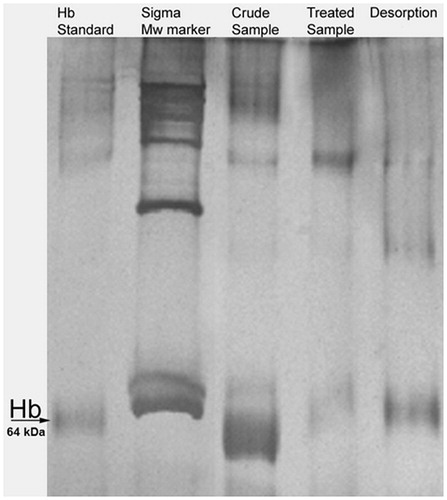
As clearly seen from , the hem group of denatured standard Hb solution in Lane 1 was observed around 64 kDa range, which was indicated with black Hb arrow. The bulky Hb band was observed for untreated sample in Lane 3, while this band disappeared after treatment of hemolysate sample with PHEMA–IDA–Ni(II) cryogel. The elution from PHEMA–IDA–Ni(II) cryogel was repeated two times and results showed that these two elutions contained Hb.
Desorption and reusability
Hb-adsorbed PHEMA cryogel was desorbed with 0.1 M phosphate buffer (pH 8, including 0.5 M urea, 1 M sodium chloride) for 2.0 h at room temperature in a continuous system. The final Hb concentration in desorption medium was determined by spectrophotometer at 406 nm. In order to test the reusability of the cryogel, Hb adsorption–desorption procedure was repeated 10 times by using the same cryogel. At the end of 10 adsorption–desorption cycles, there was no remarkable reduce in the adsorption capacity. Recovery of Hb was calculated as 81% ().
FPLC studies
As shown in , separation of Hb and the other proteins in human hemolysate was observed at 11.07 and 4.38 min, respectively. As can be seen clearly, it is well fitted with the peak of the standard Hb solution (10.06 min), which was loaded to FPLC column for both calibration and investigation the retention time of the standard Hb. The tR, N, k′, Rs values are given in . Rs value was calculated as 1.41 for other proteins in human hemolysate, respectively. Because the Rs value should be >1.0 for a good resolution of two peaks in such a chromatography system the results for the resolution of Hb–other proteins in human hemolysate can be accepted as good resolution values.
Conclusion
The depletion of the high abundant protein studies has a crucial importance for proteomic studies, which gained great attention in recent years especially in diagnosis and therapy. In our study, we developed a PHEMA-based Ni(II) chelated affinity cryogels (PHEMA–IDA–Ni(II)) for the Hb depletion from human blood. The depletion was achieved selectively up to 4.25 mg/g PHEMA–IDA–Ni(II) cryogel. As a conclusion, in this study we reported the newly synthesized PHEMA–IDA–Ni(II) cryogel column and showed it depletes Hb from human blood successfully and selectively, we believe that PHEMA–IDA–Ni(II) cryogels offered a new future promising strategy for Hb depletion.
Declaration of interest
The authors report no declarations of interest. The authors alone are responsible for the content and writing of the paper.
References
- Akgol S, Denizli A. 2004. Novel metal-chelate affinity sorbents for reversible use in catalase adsorption. J Mol Catal B. 28:7–14.
- Altıntaş EB, Türkmen D, Karakoç V, Denizli A. 2011. Hemoglobin binding from human blood hemolysate with poly(glysidyl methacrylate) beads. Colloids Surf B. 85:235–240.
- Alvarez-Llamas G, Cuesta F, Barderas MG, Darde VM, Zubiri I, Caramelo C, Vivanco F. 2009. A novel methodology for the analysis of membrane and cytosolic sub-proteomes of erythrocytes by 2-DE. Electrophoresis 30:4095–4108.
- Andac M, Denizli A. 2014. Affinity recognition-based polymeric cryogels for protein depletion studies. RSC Adv. 4:31130–31141.
- Arrua RD, Alvarez Igarzabal CI. 2011. Macroporous monolithic supports for affinity chromatography. J Sep Sci. 34:16–17.
- Bain J, Amos RJ, Bareford D, Chapman C, Davies SC, Old JM, Wild BJ. 1998. (Working party of the General Haematology, Task Force of the British Committee for Standards in Haematology) (1998) The laboratory diagnosis of haemoglobinopahies. Br J Haematol. 101:783–792.
- Baydemir G, Andaç M, Perçin I, Derazshamshir A, Denizli A. 2014. Molecularly imprinted composite cryogels for hemoglobin depletion studies from human blood. J Mol Recogn. 27:528–536.
- Baydemir G, Denizli A. 2014. Heparin removal from human plasma using molecular imprinted cryogels. Artif Cells Nanomed Biotechnol 43:403–412.
- Bereli N, Andaç M, Baydemir G, Say R, Galaev IY, Denizli A. 2008. Protein recognition via ion-coordinated molecularly imprinted supermacroporous cryogels. J Chromatogr A 1190:18–26.
- Bhattacharya D, Mukhopadhyay D, Chakrabarti A. 2007. Hemoglobin depletion from red blood cell cytosol reveals new proteins in 2-D gel-based proteomics study. Proteomics Clin Appl . 1:561–564.
- Bibi NS, Singh NK, Dsouza RN, Aasim M, Fernandez-Lahore M. 2013. Synthesis and performance of megaporous immobilized metal-ion affinity cryogels for recombinant protein capture and purification. J Chromatogr A. 1272:145–153.
- Çimen D, Denizli A. 2012. Immobilized metal affinity monolithic cryogels for cytochrome c purification. Colloids Surf B Biointerfaces. 93:29–35.
- Denizli A, Pişkin E. 1995. Heparin immobilized polyhydroxyethylmethacrylate microbeads for cholesterol removal: a preliminary report. J Chromat B. 670:157–161.
- Denizli A. 1999. Heparin-Immobilized Poly(2-Hydroxyethylmethacrylate based microspheres. J Appl Polym Sci. 74:655–662.
- Denizli A. 2002. Preparation of immune-affinity membranes for cholesterol removal from human plasma. J Chrom B. 772:357–367.
- Derazshamshir A, Baydemir G, Andac M, Say R, Galaev IY, Denizli A. 2009. Molecularly imprinted PHEMA-based cryogel for depletion of hemoglobin from human blood macromol. Chem Phys. 2101:657–668.
- Ergün B, Baydemir G, Andaç M, Yavuz H, Denizli A. 2012. Ion imprinted cryogels for in vitro removal of iron from beta-thalassemic human plasma. J Appl Polym Sci. 125:254–262.
- Goodman SR, Kurdia A, Ammann L, Kakhniashvili D, Daescu O. 2007. The human red blood cell proteome and interactome. Exp Biol Med. 232:1391–1408.
- Liu Y, Gu Y, Li M, Wei Y. 2014. Protein imprinting over magnetic nanospheres via a surface grafted polymer for specific capture of hemoglobin. N J Chem. 38:6064–6072.
- Liumbruno GD, Alessandrd A, Grazzini G, Zolla L. 2010. Blood-related proteomics. J Proteomics. 73:483–507.
- Mahn A, Reyes A, Zamorano M, Cifuentes W, Ismail M. 2010. Depletion of High abundant proteins in blood plasma by hydrophobic interaction chromatography for proteomic analysis. J Chromatogr B. 878:1038–1044.
- Noir M, Le Plieva F, Hey T, Guieyse B, Mattiasson B. 2007. Macroporous molecularly imprinted polymer/cryogel composite systems for the removal of endocrine disrupting trace contaminants. J Chromatogr A. 1154:158–164.
- Percin I, Baydemir G, Ergün B, Denizli A. 2013. Macroporous PHEMA-based cryogel discs for bilirubin removal. Artif Cells Nanomed Biotechnol. 41:172–177.
- Salgado FJ, Vazquez S, Iglesias A, Perez-Diaz A, Mera-Varela A, Arias P, Nogueira M. 2010. Application of thiophilic chromatography to deplete serum immunoglobulins in sample preparation for bidimensional electrophoresis. Anal Chim Acta. 658:18–31.
- Tetala KKRKS, Levisson M, Stamatialis DF. 2013. A metal ion charged mixed matrix membrane for selective adsorption of hemoglobin. Sep Purif Technol. 115:20–26.
- Uzun L, Armutcu C, Biçen O, Ersöz A, Say R, Denizli A. 2013. Simultaneous depletion of immunoglobulin G and albumin from human plasma using novel monolithic cryogel columns. Colloids Surf B Biointerfaces 112:1–8.
- Walpurgis K, Kohler M, Thomas A, Wenzel F, Geyer H, Schanzer W, Thevis M. 2012. Validated hemoglobin-depletion approach for red blood cell lysate proteome analysis by means of 2D PAGE and Orbitrap MS. Electrophoresis. 33:2537–2545.
- Yao K, Shen S, Yun J, Wang L, Chen F, Yu X. 2007. Protein adsorption in supermacroporous cryogels with embedded nanoparticles. Biochem Eng J. 36:139–146.
- Yıldırım A, Özgür E, Bayındır M. 2013. Impact of mesoporous silica nanoparticle surface functionality on hemolytic activity, throm bogenicity and non-spesific protein adsorption. J Mater Chem. 1:1909–1920.