Abstract
Diagnosis and treatment of lung cancer have been characterized with a variety of challenges. However, with the advancement in magnetic nanoparticle (MNP) technology, many challenges in the diagnosis and treatment of lung cancer are on the decline. The MNPs have led to many break-through in cancer therapy. This paper seeks to establish the role of MNPs in diagnosis and treatment of lung cancer. It proposes that the existing challenges in the diagnosis and treatment of lung cancer can be addressed through application of MNPs in the process.
Introduction
In spite of the extreme efforts in the last 50 years, it has only been feasible to slightly, but not considerably, lower the mortality of cancer (Anari et al. Citation2015). According to the estimates by the US National Cancer Institute, nanomedicine will verify to be trailblazing in the future prevention, diagnostic research, and treatment of cancer (Tiwari Citation2012).
Lung cancers result from complex, genetic, and epigenetic alterations characterized by stepwise malignant progression of cancer cells in associated with the accumulation genetic changes. Non-small cell lung cancer (NSCLC) accounts for around 85% of all lung cancers. Lung carcinoma (particularly, NSCLC) is the most important reason of cancer-related deaths in the United States and the second widespread cancer in both men and women and is by far the leading reason of cancer death worldwide (Munaweera et al. Citation2015, Tabatabaei Mirakabad et al. Citation2016).
Nanotechnology is probable to play a key role in cancer diagnosis by allowing the visualization of the cancer cell at an early step. Magnetic nanoparticles (MNPs) are a most important group of nano-materials with the potential to revolutionize current clinical therapeutic and diagnostic techniques. Apply of nanoparticles based on magnetic in medical uses is a new and highly interdisciplinary field offering great potential in therapeutic and diagnostic testing, in vitro, and in vivo (Ebrahimi Citation2016a, Tartaj et al. Citation2006). First medical applications used iron powder or magnetite directly in treatment methods. Recently, MNPs have become a very noticeable tool in cancer therapy. The nanoparticles including of nickel, cobalt, and iron can be guided by a magnetic field owing to their ferromagnetism. In biomedicine, mainly, iron oxide nanoparticles are being used ().
Figure 1. The role of magnetic drug targeting. Nanoparticles-based magnetic iron oxide are functionalized with a chemotherapeutic agent and injected directly into the arterial supply of the tumor (Dürr et al. Citation2013, Namiki et al. Citation2009)
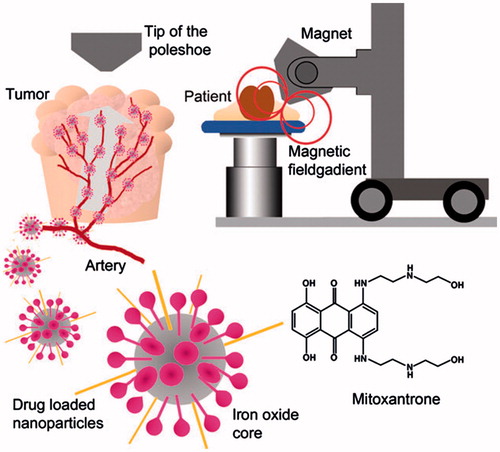
Currently, there are nanoparticles that are being applied successfully in the diagnosis of cancer and have allowed the health care practitioners to image the specific areas in the body where cancer resides. For instance, the use of magnetic particles together with the MRI scans has greatly helped in increasing the image contrast (Ebrahimi et al. Citation2016b). The contrast leads to the detection of various small cancer metastases that would otherwise be undetectable (Abdeen and Praseetha Citation2013). Also, the nanoparticles are used in the physical process of destroying the tumor tissues through the MNP hyperthermia have determined that nanoparticles have multiple applications in the diagnosis and treatment of different types of cancer (Colombo et al. Citation2012) ().
Figure 2. Multiple applications of nanoparticles in the diagnosis and treatment of different types of cancer (Colombo et al. Citation2012)
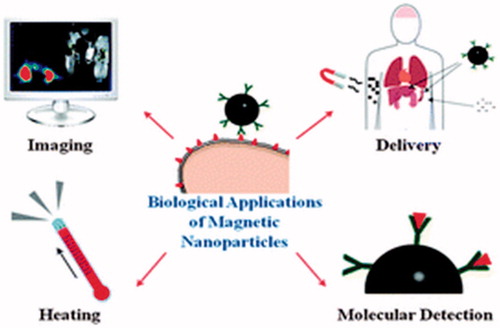
The technology uses the magnetic field to oscillate the iron oxide MNPs that creates heat (Abdeen and Praseetha Citation2013). The heat works in the form of kinetic energy to significantly reduce the size of the tumor or cancer tissue. Future research will have to focus on the pharmacokinetics of the MNPs to ensure that the particles can remain in the patient’s body for longer periods compared to the current period. Also, future research will focus on how the body can react positively to the MNPs (Fallahzadeh et al. Citation2010). One of the most pursued areas of investigates in the development of cancer treatment strategies is delivery of anticancer drugs by blend with functionalized superparamagnetic iron oxide nanoparticles (SPIONs) to their targeted site. SPION induced hyperthermia has also been used for localized killing of cancer cells. Despite their feasible biomedical apply, modification in gene expression profiles, interruption in iron homeostasis, oxidative stress, and changed cellular responses are some SPION-related toxicological aspects which necessitate owing deliberation (Anderson and Shive Citation2012, Mahmoudi et al. Citation2011, Soppimath et al. Citation2001).
Drug delivery
SPIONs have paying attention in the past decades because of their probable uses in tumor therapy, imaging, and drug delivery (McCarthy and Weissleder Citation2008, Petters et al. Citation2014, Salloum et al. Citation2009). MNPs appear to be extremely suitable for drug delivery. In fact, they can be prepared in diverse sizes and can be functionalized (surface-coated) in order to carry various molecules. Several issues need to be considered when using MNPs as carriers counting colloidal stability and biocompatibility (Faraji et al. Citation2010, Furlani Citation2010, Tartaj et al. Citation2003). The valuable use of MNPs for biomedical applications like targeted drug delivery depends on different factors associated to the size and magnetism of the biocompatible nanoparticles (Akbarzadeh et al. Citation2012a, Sun et al. Citation2008) ().
Figure 3. Scheme of the treatment strategy using magnetic nanoparticles (MNPs). Functionalized MNPs accumulate in the tumor tissues by the drug delivery system (DDS) (Sun et al. Citation2008)
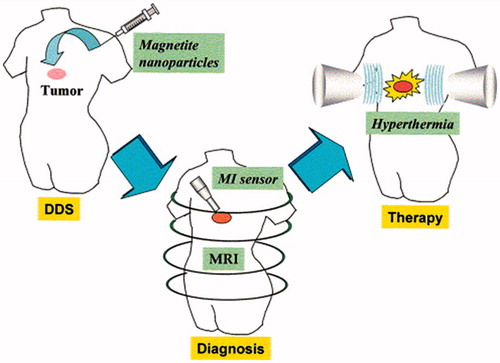
Nanoparticle based drug delivery systems may solve the insurmountable obstacle of treating neurological diseases: delivery across the blood–brain barrier (Nasrabadi et al. Citation2016, Kreuter Citation2001). But issues of possible embolization with MNPs aggregate in capillaries and the essential for large distances between the pathological site and external magnetic field still in present as a problem (Schroeder et al. Citation1998).
The most important problem on chemotherapy treatment is the non-specificity of the drugs, damaging both sick and healthy cells. To solve this problem, as you can see in , MNPs can be used to deliver the therapeutic agents to the desired target area, maintaining the particles at the exact location during the drug release, when combined with an exterior magnetic field.
Furthermore, a controlled drug delivery based on MNPs should be able to uphold drug levels inside the necessary concentration range for treatment, avoiding the over dosage and decreasing side effects (Lima-Tenório et al. Citation2015).
Direct injection of MNP into the tumor
Although most commercially available products using nanoparticles are found as contrast agents, recently milestones have been reached regarding anticancer therapy (Akbarzadeh et al. Citation2012b). Nowadays, the delivery of MNPs is based on a direct intra-tumoral injection, which restrictions the clinical application of this method (Gobbo et al. Citation2015). More development of tumor targeting by the SPIONs needs a particular surface coating, which can provide the specificity of these nanoparticles delivery to the tumor cells in vivo (Gupta and Gupta Citation2005, Maier-Hauff et al. Citation2011).
Methods and materials
This study applies a review of literature as a method of understanding the application of MNPs in the diagnosis and treatment of lung cancer. It focuses on recent scholarly works in the areas of nanotechnology, MNPs, and lung cancer. Currently, these areas have attracted much scholarly interest, and there are many research works that focus on the areas. Sukumar et al. (Citation2013) identifies that 23% of cancer-related deaths result from lung cancer. They attribute the situation to lack of early diagnosis with about 50% of the cases identified in stage 4, which reduces chances of survival. In the United States, about 85% of the lung cancers are categorized as non-small cell (Hadjipanayis et al. Citation2010, Kimura et al. Citation2009, Sukumar et al. Citation2013). Since it is difficult to access the deeper parts of the lung to conduct conventional therapy, the treatment of lung cancer becomes more complicated (Munaweera et al. Citation2015). However, development in the area of nanotechnology is changing the situation. Nanotechnology has found application in the fields of biomedicine because it can control the delivery of drugs, provide great contrast in the magnetic resonance imaging (Akbarzadeh et al. Citation2012b) hyperthermia, and promote tissues and cell targeting. All these applications are useful in cancer therapy (Asin et al. Citation2012a, Daraee et al. Citation2015). The utility of the nanoparticles derives from their optical and magnetic properties. The optical and magnetic properties are due to the quantum confinement and large surface area effects that are present at the nanoscale (Asin et al. Citation2012a, Ding et al. Citation2013, Kong et al. Citation2012).
Magnetic hyperthermia, which involves the use of superparamagnetic materials to make heat through application of an exterior alternating magnetic fields (AMF), is a non-invasive method for lung tumor remove. The comparatively inert nature of the magnetic substances in the absence of magnetic field and their efficacy in the attendance of AMF make this procedure very appropriate for achieving an on-demand answer (Sadhukha et al. Citation2013).
Obaidat et al. (Citation2015) have noted that magnetic particle hyperthermia is useful as a local therapy for the removal of tumors from the body. The therapy uses the MNPs to heat the tumor cells and initiate a non-reversible damage to the tumor cells, as well as, the surrounding tissue (Gorjikhah et al. Citation2016, Obaidat et al. Citation2015). When MNPs reach the tumor, a magnet oscillates the nanoparticles to heat up the tumor to about 42 °C to 46 °C (Asin et al. Citation2012b, Obaidat et al. Citation2015). The temperature is slightly above the normal body temperature of 37 °C, but it is sufficient to damage the cells and prevent the damaging effects of the cancer cells. However, if it is difficult to reach the tumor, the treatment may not be plausible or effective (Asin et al. Citation2012b, Cervadoro et al. Citation2013, Gorjikhah et al. Citation2016, Landi Citation2013, Obaidat et al. Citation2015). Confirm that the challenge is mostly due to the low heat conversion rate. Many metals have magnetic properties, but, the Iron oxide nanoparticles are that preferred particles in cancer therapy (Rivas et al. Citation2012). The iron oxide is proven to be nontoxic and biocompatible. Such properties are important because they ensure that the patient is comfortable during the therapy. Furthermore, the iron oxide nanoparticles work great with the magnetic force to heat the target area in cancer therapy (Disch et al. Citation2012). The nanoparticles are given a special coating particularly the aminosilane before the particles are transmitted to the tumor (Rivas et al. Citation2012). The coating ensures that the nanoparticles move target tissue rather than spreading out to other parts of the body to cause other complications during the hyperthermia. Nanotechnology is an expanding niche that is revolutionizing cancer management and treatment (Babu et al. Citation2013). Precisely, the nanoscale drug delivery systems are promising in “successful formulation and enhancement of the therapeutic efficacy of many anticancer agents” (Babu et al. Citation2013, Jiang et al. Citation2013). Also, the nanoparticles have superior biocompatibility that ensures the protection of the nucleic acid from degradation and delivering the therapeutic genes to the cancer cells. This makes the nanoparticles ideal system for delivery (Akbarzadeh et al. Citation2012b). However, still, there are several challenges with the various nanoparticle-based therapies. Only a few of the therapies have indicated clinical success. It is difficult identifying the ideal drug delivery system for the various types of novel drugs with diverse physiological features and varying levels of therapeutic activities (Gudoshnikov et al. Citation2012, Gudoshnikov et al. Citation2013).
Results and discussion
Cancer has always presented many difficulties during treatment. In most cases, the challenges are prompted by the late diagnosis of the tumor cells. The traditional methods of detecting cancer often detect cancer when it is already at the point where it is very difficult to treat the cancer especially when the traditional methods of treatment such as chemotherapy and surgery are used. The MNPs technology helps in detecting the tumor early and enables adequate treatment (Akbarzadeh et al. Citation2012b). The technology is commonly used to increase the contrast in the MRI scans (Baviskar et al. Citation2012, Hadjipanayis et al. Citation2010) and to specifically target the affected area by monitoring the tumor cells through surface engineering. It facilitates noninvasive targeting and monitoring the tumors in real time to detect small metastases that would otherwise be undetectable.
Other than the imaging purposes, the MNPs are used in MNP hypothermia therapy (Askari et al. Citation2015). The magnetic particles are targeted to a tissue, and the magnetic energy absorption of the nanoparticles ensures a localized heating of the targeted cell leading to the death of the cell (Rivas et al. Citation2012). As such, the increase in temperature of the nanoparticle can be used in killing the cancer cells. Iron oxide nanoparticles are biocompatible and nontoxic implying that they are very suitable for hyperthermia (Disch et al. Citation2012). There are several works that aim at optimizing the design of nanoparticles and provide the appropriate properties that would allow maximization of the heat generated (Rivas et al. Citation2012). Also, there is the need for an improved heating power rate. Higher rates of heating can ensure that the patient is given low doses of MNPs and a short time of stay of the nanoparticles in the body (Chung et al. Citation2016, Rivas et al. Citation2012).
However, there are concerns about the possibility of the direct nanoparticles reaching the damaged tissue (Rivas et al. Citation2012). While the external magnetic field can ensure that the nanoparticles flow to the target cells, the organic and inorganic molecules in the body can bind on the nanoparticles’ surface and trap them from reaching the target area (Gudoshnikov et al. Citation2012). But, recently an approach that could solve the problem was approved. The approach involves introducing iron oxide nanoparticles that are coated with aminosilane in treating solid brain tumors through hyperthermia therapy (Thakor and Gambhir Citation2013). The main challenge to the application of nanoparticles in the treatment of lung cancer lies in the low rate of energy conversion in the study, Cervadoro et al. (Citation2013) has identified that the heat absorption rate depends on various factors (Cervadoro et al. Citation2013). The factors include the nanoparticle characteristics like surface properties, size, and shape, and the functional conditions of the AMF such as the frequency and strength (Obaidat et al. Citation2015). The study suggests that the MNPs’ heating efficiency can be enhanced through proper design of the shape, surface, and composition selection. From the study, the table below represents the list of the specific absorption rate (SAR) values and different nanoparticle parameters (Obaidat et al. Citation2015) ().
Table1. Table of specific absorption rate (SAR) values and parameters of nanoparticles
Conclusion
Lung cancer is a form of cancer that requires early detection and effective treatment as a way of preventing the dangers of the disease. Because of the many challenges posted by the traditional methods of detecting and treating lung cancer, nanoparticles present the opportunity for ensuring the early detection and effective treatment. While there are significant barriers to the effective use of nanoparticles in the diagnosis and treatment of lung cancer, progress is being made, and the use of nanotechnology will be the best strategy for diagnosing and treating lung cancer.
Funding information
This work is funded by a 2015 grant Drug Applied Research Center, Tabriz University of Medical Sciences.
Disclosure statement
The authors report no declaration of interest. The authors alone are responsible for the content and writing of the paper.
References
- Abdeen S, Praseetha PK. 2013. Diagnostics and treatment of metastatic cancers with magnetic nanoparticles. J Nanomed Biother Discov. 3:115.
- Akbarzadeh A, Samiei M, Davaran S. 2012a. Magnetic nanoparticles: preparation, physical properties, and applications in biomedicine. Nanoscale Res Lett. 7:1–13.
- Akbarzadeh A, Samiei M, Joo SW, Anzaby M, Hanifehpour Y, Nasrabadi HT, Davaran S. 2012b. Synthesis, characterization and in vitro studies of doxorubicin-loaded magnetic nanoparticles grafted to smart copolymers on A549 lung cancer cell line. J Nanobiotechnol. 10:46–58.
- Anari E, Akbarzadeh A, Zarghami N. 2015. Chrysin-loaded PLGA-PEG nanoparticles designed for enhanced effect on the breast cancer cell line. Artif Cells Nanomed Biotechnol. [Epub ahead of print]. doi: 10.3109/21691401.2015.1029633.
- Anderson JM, Shive MS. 2012. Biodegradation and biocompatibility of PLA and PLGA microspheres. Adv Drug Deliv Rev. 64:72–82.
- Asin L, Ibarra MR, Tres A, Goya GF. 2012a. By magnetic, controlled cell death. Pharm Res. 29:1319–1327.
- Asin L, Ibarra MR, Tres A, Goya GF. 2012b. Controlled cell death by magnetic hyperthermia: effects of exposure time, field amplitude, and nanoparticle concentration. Pharm Res. 29:1319–1327.
- Askari S, Salehi R, Zarghami N, Akbarzadeh A, Rahmati-Yamchi M. 2015. The anticancer effects of biodegradable nanomagnetic dual natural components on the leptin gene expression in lung cancer. [Epub ahead of print]. doi: 10.3109/21691401.2015.1101000.
- Babu A, Templeton AK, Munshi A, Ramesh R. 2013. Nanoparticle-based drug delivery for therapy of lung cancer: progress and challenges. J Nanomater. 2013:863951.
- Baviskar DT, Amritkar AS, Chaudhari HS, Jain DK. 2012. Modulation of drug release from nanocarriers loaded with a poorly water soluble drug (flurbiprofen) comprising natural waxes. Die Pharmazie Int J Pharm Sci. 67:701–705.
- Cervadoro A, Giverso C, Pande R, Sarangi S, Preziosi L, Wosik J, Brazdeikis A, Decuzzi P. 2013. Design maps for the hyperthermic treatment of tumors with superparamagnetic nanoparticles. PLoS One. 8:e57332.
- Chung JH, Kim YK, Kim KH, Kwon TY, Vaezmomeni SZ, Samiei M, et al. 2016. Synthesis, characterization, biocompatibility of hydroxyapatite-natural polymers nanocomposites for dentistry applications. Artif Cells Nanomed Biotechnol. 44:277–284.
- Colombo M, Carregal-Romero S, Casula MF, Gutiérrez L, Morales MP, Böhm IB, Parak WJ. 2012. Biological applications of magnetic nanoparticles. Chem Soc Rev. 41:4306–4334.
- Daraee H, Pourhassanmoghadam M, Akbarzadeh A, Zarghami N, Rahmati-Yamchi M. 2015. Gold nanoparticle-oligonucleotide conjugate to detect the sequence of lung cancer biomarker. Artif Cells Nanomed Biotechnol. [Epub ahead of print]. doi:10.3109/21691401.2015.1031905.
- Ding W, Wang F, Zhang J, Guo Y, Ju S, Wang H. 2013. A novel local anti-colorectal cancer drug delivery system: negative lipidoid nanoparticles with a passive target via a size-dependent pattern. Nanotechnology. 24:375101.
- Disch S, Wetterskog E, Hermann RP, Wiedenmann A, Vainio U, Salazar-Alvarez G, Bergström L, Brückel T. 2012. Quantitative spatial magnetization distribution in iron oxide nanocubes and nanospheres by polarized small-angle neutron scattering. New J Phys. 14:013025.
- Dürr S, Janko C, Lyer S, Tripal P, Schwarz M, Zaloga J, Tietze R, Alexiou C. 2013. Magnetic nanoparticles for cancer therapy. Nanotechnol Rev. 2:395–409.
- Eatemadi A, Daraee H, Zarghami N, Yar HM, Akbarzadeh A. 2016a. Nanofiber: synthesis and biomedical applications. Artif Cells Nanomed Biotechnol. 44:111–121.
- Ebrahimi E, Akbarzadeh A, Abbasi E, Khandaghi AA, Abasalizadeh F, Davaran S. 2016a. Novel drug delivery system based on doxorubicin-encapsulated magnetic nanoparticles modified with PLGA-PEG1000 copolymer. Artif Cells Nanomed Biotechnol. 44:290–297.
- Ebrahimi E, Khandaghi A, Valipour F, Babaie S, Asghari F, Motaali S, et al. 2016b. In vitro study and characterization of doxorubicin-loaded magnetic nanoparticles modified with biodegradable copolymers. Artif Cells Nanomed Biotechnol. 44:550–558.
- Fallahzadeh S, Bahrami H, Akbarzadeh A, Tayarani M. 2010. High-isolation dual-frequency operation patch antenna using spiral defected microstrip structure. IEEE Antenn Wirel Propagat Lett. 9:122–124.
- Faraji M, Yamini Y, Rezaee M. 2010. Magnetic nanoparticles: synthesis, stabilization, functionalization, characterization, and applications. J Iranian Chem Soc. 7:1–37.
- Furlani EP. 2010. Magnetic biotransport: analysis and applications. Materials. 3:2412–2446.
- Gobbo OL, Sjaastad K, Radomski MW, Volkov Y, Prina-Mello A. 2015. Magnetic nanoparticles in cancer theranostics. Theranostics. 5:1249–1263.
- Gorjikhah F, Davaran S, Salehi R, Bakhtiari M, Hasanzadeh A, Panahi Y, Emamverdy M, Akbarzadeh A. 2016. Improving “lab-on-a-chip” techniques using biomedical nanotechnology: a review. Artif Cells Nanomed Biotechnol. [Epub ahead of print]. doi: 10.3109/21691401.2015.1129619.
- Gudoshnikov SA, Ya Liubimov B, Usov NA. 2012. Hysteresis losses in a dense superparamagnetic nanoparticle assembly. AIP Adv. 2:012143.
- Gudoshnikov SA, Liubimov BY, Sitnov YS, Skomarovsky VS, Usov NA. 2013. AC magnetic technique to measure specific absorption rate of magnetic nanoparticles. J Superconduct Novel Magn. 26:857–860.
- Gupta AK, Gupta M. 2005. Synthesis and surface engineering of iron oxide nanoparticles for biomedical applications. Biomaterials. 26:3995–4021.
- Hadjipanayis CG, Machaidze R, Kaluzova M, Wang L, Schuette AJ, Chen H, Wu X, Mao H. 2010. EGFRvIII antibody–conjugated iron oxide nanoparticles for magnetic resonance imaging–guided convection-enhanced delivery and targeted therapy of glioblastoma. Cancer Res. 70:6303–6312.
- Jiang L, Li X, Liu L, Zhang Q. 2013. Thiolated chitosan-modified PLA-PCL-TPGS nanoparticles for oral chemotherapy of lung cancer. Nanoscale Res Lett. 8:1–11.
- Kimura S, Egashira K, Chen L, Nakano K, Iwata E, Miyagawa M, et al. 2009. Nanoparticle-mediated delivery of nuclear factor κB decoy into lungs ameliorates monocrotaline-induced pulmonary arterial hypertension. Hypertension. 53:877–883.
- Kong M, Li X, Wang C, Ding C, Dong A, Duan Q, Shen Z. 2012. Tissue distribution and cancer growth inhibition of magnetic lipoplex-delivered type 1 insulin-like growth factor receptor shRNA in nude mice. Acta Biochim Et Biophys Sin. 44:591–596.
- Kreuter J. 2001. Nanoparticulate systems for brain delivery of drugs. Adv Drug Deliv Rev. 47:65–81.
- Landi GT. 2013. Simple models for the heating curve in magnetic hyperthermia experiments. J Magn Magn Mater. 326:14–21.
- Lima-Tenório MK, Gómez Pineda EA, Ahmad NM, Fessi H, Elaissari A. 2015. Magnetic nanoparticles: in vivo cancer diagnosis and therapy. Int J Pharm. 493:313–327.
- Mahmoudi M, Laurent S, Shokrgozar MA, Hosseinkhani M. 2011. Toxicity evaluations of superparamagnetic iron oxide nanoparticles: cell “vision” versus physicochemical properties of nanoparticles. ACS Nano. 5:7263–7276.
- Maier-Hauff K, Ulrich F, Nestler D, Niehoff H, Wust P, Thiesen B, et al. 2011. Efficacy and safety of intratumoral thermotherapy using magnetic iron-oxide nanoparticles combined with external beam radiotherapy on patients with recurrent glioblastoma multiforme. J Neurooncol. 103:317–324.
- McCarthy JR, Weissleder R. 2008. Multifunctional magnetic nanoparticles for targeted imaging and therapy. Adv Drug Deliv Rev. 60:1241–1251.
- Munaweera I, Shi Y, Koneru B, Saez R, Aliev A, Di Pasqua AJ, Balkus KJ Jr. 2015. Chemoradiotherapeutic magnetic nanoparticles for targeted treatment of nonsmall cell lung cancer. Mol Pharm. 12:3588–3596.
- Namiki Y, Namiki T, Yoshida H, Ishii Y, Tsubota A, Koido S, et al. 2009. A novel magnetic crystal-lipid nanostructure for magnetically guided in vivo gene delivery. Nat Nanotechnol. 4:598–606.
- Nasrabadi HT, Abbasi E, Davaran S, Kouhi M, Akbarzadeh A. 2016. Bimetallic nanoparticles: Preparation, properties, and biomedical applications. Artif Cells Nanomed Biotechnol. 44:376–380.
- Obaidat IM, Issa B, Haik Y. 2015. Magnetic properties of magnetic nanoparticles for efficient hyperthermia. Nanomaterials. 5:63–89.
- Petters C, Irrsack E, Koch M, Dringen R. 2014. Uptake and metabolism of iron oxide nanoparticles in brain cells. Neurochem Res. 39:1648–1660.
- Pilehvar-Soltanahmadi Y, Akbarzadeh A, Moazzez-Lalaklo N, Zarghami N. 2015. An update on clinical applications of electrospun nanofibers for skin bioengineering. Artif Cells Nanomed Biotechnol. [Epub ahead of print]. doi:10.3109/21691401.2015.1036999.
- Rivas J, Bañobre-López M, Piñeiro-Redondo Y, Rivas B, López-Quintela MA. 2012. Magnetic nanoparticles for application in cancer therapy. J Magn Magn Mater. 324:3499–3502.
- Sadhukha T, Wiedmann TS, Panyam J. 2013. Inhalable magnetic nanoparticles for targeted hyperthermia in lung cancer therapy. Biomaterials. 34:5163–5171.
- Salloum M, Ma R, Zhu L. 2009. Enhancement in treatment planning for magnetic nanoparticle hyperthermia: optimization of the heat absorption pattern. Int J Hyperthermia. 25:309–321.
- Schroeder U, Sommerfeld P, Ulrich S, Sabel BA. 1998. Nanoparticle technology for delivery of drugs across the blood-brain barrier. J Pharm Sci. 87:1305–1307.
- Soppimath KS, Aminabhavi TM, Kulkarni AR, Rudzinski WE. 2001. Biodegradable polymeric nanoparticles as drug delivery devices. J Control Release. 70:1–20.
- Sukumar UK, Bhushan B, Dubey P, Matai I, Sachdev A, Packirisamy G. 2013. Emerging applications of nanoparticles for lung cancer diagnosis and therapy. Int Nano Lett. 3:1–17.
- Sun C, Lee JSH, Zhang M. 2008. Magnetic nanoparticles in MR imaging and drug delivery. Adv Drug Deliv Rev. 60:1252–1265.
- Tabatabaei Mirakabad FS, Akbarzadeh A, Milani M, Zarghami N, Taheri-Anganeh M, Zeighamian V, Badrzadeh F, Rahmati-Yamchi M. 2016. A Comparison between the cytotoxic effects of pure curcumin and curcumin-loaded PLGA-PEG nanoparticles on the MCF-7 human breast cancer cell line. Artif Cells Nanomed Biotechnol. 44:423–430.
- Tartaj P, Morales MDD, Veintemillas-Verdaguer S, Gonzalez-Carreno T, Serna CJ. 2003. The preparation of magnetic nanoparticles for applications in biomedicine. J Phys D Appl Phys. 36:R182–R197.
- Tartaj P, Morales MP, Veintemillas-Verdaguer S, Gonzalez-Carreño T, Serna CJ. 2006. Synthesis, properties and biomedical applications of magnetic nanoparticles. Handb Magn Mater. 16:403–482.
- Thakor AS, Gambhir SS. 2013. Nanooncology: the future of cancer diagnosis and therapy. CA Cancer J Clin. 63:395–418.
- Tiwari M. 2012. Nano cancer therapy strategies. J Cancer Res Ther. 8:19–22.