Abstract
Aquaglyceroporins (AQP3, AQP7, AQP9 and AQP10) encompass a subfamily of aquaporins that allow the movement of water and other small solutes, especially glycerol, through cell membranes. Adipose tissue constitutes a major source of glycerol via AQP7. We have recently reported that, in addition to the well-known expression of AQP7 in adipose tissue, AQP3 and AQP9 are also expressed in omental and subcutaneous fat depots. Moreover, insulin and leptin act as regulators of aquaglyceroporins through the PI3K/Akt/mTOR pathway. AQP3 and AQP7 appear to facilitate glycerol efflux from adipose tissue while reducing the glycerol influx into hepatocytes via AQP9 to prevent the excessive lipid accumulation and the subsequent aggravation of hyperglycemia in human obesity. This Extra View focuses on the control of glycerol release by aquaglyceroporins in the adipose tissue and briefly discusses the importance of glycerol as a substrate for hepatic gluconeogenesis, pancreatic insulin secretion and cardiac ATP production.
Aquaglyceroporins and Glycerol as Metabolic Substrate
Over the past four decades, evidence for the existence of physiological systems aimed at body weight and energy homeostasis has been provided.Citation1 The adipose tissue has been extensively investigated and has been revealed to act as an endocrine organ that secretes a large number of hormones, growth factors, enzymes, cytokines, complement factors and matrix proteins, collectively termed as adipokines.Citation2 Upper body excess adiposity is associated with increased incidence of metabolic disturbances, elevated risk of cardiovascular disease and premature death.Citation3–Citation5 Additionally, selective reduction of visceral adiposity with diet, exercise or bariatric surgery is accompanied by improvements in intermediary metabolism.Citation6,Citation7 In spite of the growing understanding of the fundamental biology of energy homeostasis, it is evident that crucial pathways have yet to be identified. Aquaglyceporins, integral plasma membrane proteins with proven transport and signaling capabilities, have emerged as key players in shifting the focus of obesity and insulin resistance development.Citation8,Citation9
Aquaporins are channel-forming integral membrane proteins that allow the movement of water across the cell membranes.Citation10,Citation11 These selective channels belong to the large superfamily of major intrinsic proteins (MIP) transmembrane channels and have been found in virtually every living organism, including eubacteria, archaea, fungi, protozoa, plants and animals. Both site-directed mutagenesis and high-resolution electron microscopy studies have revealed that the secondary structure of aquaporins contains six transmembrane α-helices with two highly conserved non-bilayer-spanning loops with the motif asparagine-proline-alanine.Citation12 The three-dimensional structure of aquaporins resembles an hourglass;Citation13,Citation14 within the lipid bilayer, aquaporins form a tetrameric quaternary structure, with each monomer defining a single pore.Citation14 To date, 13 mammalian aquaporins (AQP0-12) have been identified. Functional studies have defined a subgroup of aquaporins (AQP3, AQP7, AQP9 and AQP10) as aquaglyceroporins, given their permeability, not only to water, but also to small solutes, such as glycerol and urea.Citation8,Citation10
Circulating glycerol results from fat lipolysis, diet-derived glycerol or glycerol reabsorbed in the proximal tubules.Citation15–Citation17 Glycerol represents an important metabolite for the control of fat accumulation, as the carbon backbone of triacylglycerols (TAG) and for glucose homeostasis, given that glycerol constitutes the major substrate for hepatic gluconeogenesis during fasting.Citation16,Citation18 In addition, glycerol also serves as an energy substrate for pancreatic cells and cardiomyocytes via the glycerol-3-phosphate shuttle, a reaction that allows the NADH synthesized in the cytosol by glycolysis to contribute to the oxidative pathway in the mitochondria to generate ATP.Citation19 Thus, the regulation of glycerol transport by aquaglyceroporins contributes to the control of fat accumulation, glucose homeostasis, cardiac energy production and pancreatic insulin secretion, among other functions. The present Extra View focuses on advances in the metabolic and cardiac effects of aquaglyceroporins in rodents and humans.
Lipogenesis and Lipolysis (AQP3 and AQP7)
Adipose tissue constitutes the most important source of plasma glycerol.Citation15 To date, AQP7 was considered the unique glycerol channel in the adipose tissue, but it has been recently shown that AQP3 and AQP9 represent novel additional pathways for the transport of glycerol in human adipocytes ().Citation20,Citation21 Furthermore, the existence of other yet-undiscovered glycerol facilitators cannot be discarded.Citation21,Citation22 Teleological reasons for the presence of several glycerol channels in adipocytes include the complex and redundant signaling network involved in the regulation of lipid and glucose metabolism. The redundancy of proteins exerting similar biological functions is usual in physiology, in particular with biologically critical processes, e.g., the main glucose transporter in insulin-sensitive tissues is GLUT4, but other glucose transporters GLUT1-12 are also expressed and active in liver, adipose tissue or skeletal muscle.Citation23 Aquaglyceroporins display different subcellular localization in murine 3T3-L1 adipocytes; while AQP3 is present in the plasma membrane and cytoplasm, AQP7 resides predominantly in the cytoplasm upon the lipid droplets, whereas AQP9 is constitutively expressed in the plasma membranes.Citation21 The main function of aquaglyceroporins in adipocytes is the control of glycerol uptake and release, two key steps for TAG synthesis (lipogenesis) and hydrolysis (lipolysis), respectively.Citation8 In this regard, aquaglyceroporins are regulated by lipogenic (mainly insulin) and lipolytic (exemplified by leptin and catecholamines) hormones ().Citation21
Under lipogenic conditions, such as overnutrition or lack of exercise, adipocytes synthesize TAG from the esterification of free fatty acids (FFA) with glycerol-3-phosphate. Several membrane proteins, including fatty acid-binding protein (FABP), fatty acid translocase (FAT, CD36) or fatty acid transporter protein (FATP), facilitate the FFA transport across the membrane.Citation24 In addition, lipoprotein lipase (LPL) (located on the surface of adipocytes) removes FFA from chylomicrons and very low-density lipoproteins.Citation25 The other metabolite required for TAG biosynthesis, glycerol-3-phosphate, derives from three metabolic sources: (1) glucose, since glycerol-3-phosphate constitutes a secondary metabolite of glycolysis;Citation8,Citation18 (2) lipolysis-derived glycerol, which is phosphorylated by glycerol kinase (GK)Citation26 and (3) aquaglyceroporin-mediated glycerol uptake ().Citation21 AQP9 appears to be the channel responsible for glycerol influx into adipocytes, since it is a constitutively expressed integral membrane protein not acutely regulated by vesicular trafficking.Citation21,Citation27 Insulin stimulates lipogenesis through the stimulation of glucose uptake after the translocation of glucose transporter 4 (GLUT4) to the plasma membrane as well as through the activation of LPL and, hence, through FFA uptake after binding to its receptor in adipocytes.Citation8 Under physiological conditions, insulin regulates the expression of aquaglyceroporins to either increase or decrease glycerol uptake and/or release from adipocytes.Citation17,Citation18 Insulin reportedly represses the expression of Aqp7 gene in murine adipocytes through negative insulin response elements (IRE) in its gene promoter.Citation24,Citation28 Interestingly, insulin increases the protein expression of AQP3, AQP7 and AQP9 in human adipocytes through the phosphatidylinositol-3-kinase (PI3K)/Akt/mammalian target of rapamycin (mTOR) signaling pathway,Citation21 suggesting divergent effects of insulin on the regulation of aquaglyceroporins in humans compared to rodents. Plausible explanations for these diverse effects of insulin in humans compared to rodents might be related to species-specific differences in the physiological relevance of aquaglyceroporins (mouse vs. human) based on the following facts: (1) glycerol becomes the major substrate for hepatic gluconeogenesis in rodents during fasting and post-absorptive states, while pyruvate constitutes the main substrate of the gluconeogenic pathway in humans;Citation15,Citation18 (2) the rare cases of human AQP3 and AQP7 deficiency identified so far do not resemble the same evident phenotypic characteristics observed in miceCitation29,Citation30 and (3) the expression of aquaglyceroporins is elevated in insulin-resistant states,Citation28,Citation31 resulting in increased plasma glycerol and hepatic glucose production in obese, insulin-resistant subjects.Citation21,Citation29
In circumstances of negative energy balance, such as fasting or exercise, TAG are hydrolyzed to glycerol and FFA by the adipose triglyceride lipase (ATGL) as well as the hormone-sensitive lipase (HSL) and released into the bloodstream. Catecholamines (noradrenaline and adrenaline) regulate lipolysis through lipolytic β-adrenoceptors (β1, β2 and β3) and anti-lipolytic α2-adrenoceptors.Citation32 The β-adrenoceptors couple to Gs proteins that activate adenylate cyclase, leading to an increase in cAMP production, which is followed by activation of protein kinase A (PKA) that induces HSL phosphorylation and translocation from the cytosol to the lipid droplets, thereby increasing lipolysis (). AQP3 and AQP7 facilitate glycerol efflux from murine 3T3-L1 adipocytes in response to the β-adrenergic agonist isoproterenol-induced lipolytic stimulus via its translocation from the cytosolic fraction (AQP3) or lipid droplets (AQP7) to the plasma membrane.Citation21,Citation33–Citation35 Short-term treatment with isoproterenol induces AQP3 and AQP7 translocation without changes in their expression, whereas long-term stimulation with isoproterenol reduces the expression of Aqp7 in murine 3T3-L1 cells.Citation21,Citation36 Another lipolytic factor, such as the adipocyte-derived hormone leptin, has been also shown to repress AQP7 protein expression via the PI3K/Akt/mTOR signaling cascade,Citation21 suggesting a negative feedback regulation in lipolytic states to restrict glycerol release from fat cells. Moreover, a recent study has shown that carboxymethyl chitin, a mucopolysaccharide with proposed anti-obesity properties, stimulates lipolysis by increasing HSL and AQP7 expression levels in 3T3-L1 adipocytes.Citation37 AQP7 appears to be one of the main channels for glycerol release from adipocytes. In this sense, it has been shown that adipocytes of Aqp7-deficient mice exhibit a higher intracellular glycerol content than those of wild-type mice.Citation38,Citation39 In humans, the only subject homozygous for the AQP7 missense mutation (G264V) identified so far exhibited normal body weight and TAG concentrations, but he did not show an exercise-induced increase in glycerol release in spite of elevated plasma noradrenaline concentrations.Citation29
Human obesity is associated with an altered expression profile of aquaporins in adipose tissue.Citation20,Citation40–Citation43 Fat depot-specific differences in the gene expression of AQP7 in human obesity have been reported. Thus, an overexpression of AQP7 in omental adipose tissue suggests an increase in the overall lipolytic capacity, while a repression of AQP7 in subcutaneous fat points to the promotion of an intracellular glycerol accumulation and a progressive adipocyte hypertrophy.Citation20,Citation21,Citation40–Citation42 Human obesity-associated T2D is related to an additional alteration of the adipose expression profile of AQP3, AQP7 and AQP9 compared to obese normoglycemic subjects. These findings suggest that the regulation of aquaglyceroporins in human adipose tissue is more related to insulin resistance than to obesity.Citation21 Interestingly, it has been shown that the administration of the insulin-sensitizing agents thiazolidinediones (TZD), e.g., pioglitazone and rosiglitazone, augments the expression of adipose AQP7 in rodents without increasing glycerol release from adipocytes.Citation44,Citation45 This apparent paradox results from the parallel increase of GK expression and activity induced by TZD in adipocytes, favoring the recycling of glycerol for TAG synthesis instead of stimulating the release of glycerol to the bloodstream.Citation26,Citation45 Therefore, the undesirable increase of adipose AQP7 expression in insulin-resistant states, plausibly leading to elevated plasma glycerol levels and, consequently, to hepatic gluconeogenesis, can be overcome by an increased glycerol recycling in adipocytes using TZD.
Hepatic Gluconeogenesis and TAG Synthesis (AQP9)
The liver plays a central role in glycerol metabolism, since it is responsible for 70–90% of the whole-body glycerol metabolism.Citation15 AQP7 represents the main gateway for the delivery of fat-derived glycerol, while glycerol enters hepatocytes via AQP9, where it is converted into glycerol-3-phosphate, a precursor of gluconeogenesis and a direct source for TAG synthesis ().Citation8,Citation18 Pyruvate also constitutes a source for glycerol-3-phosphate by the enzymatic activity of pyruvate carboxylase (PC) and phosphoenolpyruvate carboxykinase (PEPCK).Citation15 Insulin inhibits gluconeogenesis by reducing the activity of PEPCK as well as by blocking glucogenolysis (i.e., the conversion of glycogen polymers to glucose monomers).Citation18 AQP9 constitutes the most important glycerol channel in hepatocytes and is localized at the sinusoidal plasma membrane that faces the portal vein.Citation46–Citation48 However, AQP3 and AQP7 are also expressed in human hepatic cells, thereby demanding the need for a functional explanation for the redundancy of diverse glycerol channel in the same tissue.Citation21,Citation49
The coordinated regulation of adipose and hepatic aquaglyceroporins is required for the control of whole-body glucose homeostasis and lipid accumulation in rodents and humans.Citation21,Citation28,Citation42 In this sense, Aqp3-deficient mice develop nephrogenic diabetes insipidus,Citation50 Aqp7 deletion leads to obesityCitation22,Citation38,Citation39 and Aqp9 deficiency favors the onset of diabetes.Citation51 Insulin represses adipose Aqp7 and hepatic Aqp9 gene expression through the negative IRE in their gene promoters in rodents.Citation24,Citation28,Citation52 Murine Aqp7 deficiency is associated with a failure of the antilipolytic action of insulin, due to impaired IRS-1-associated PI3K activity in adipocytes as well as a defective insulin-induced inhibition of hepatic gluconeogenesis, a result of low IRS-2-associated PI3K activity in hepatocytes.Citation38 Contrary to what is observed in rodents, the rare cases of human AQP3 and AQP7 deficiency do not resemble the phenotypic characteristics observed in transgenic knockout mice. The individuals carrying a homozygous mutation in AQP3 and AQP7 are neither obese nor diabetic.Citation29,Citation30 The regulation of aquaglyceroporins induced by insulin in human hepatocytes also appears to be different from the control that takes place in mice, since insulin upregulates the expression of aquaglyceroporins in human hepatic cells.Citation21 Insulin-mediated elevation of AQP3 and AQP7, two glycerol channels surrounding the lipid droplets, may reflect an increased intrahepatocellular TAG content, given that insulin induces hepatic steatosis through the activation of the expression of lipogenic genes.Citation53 On the other hand, insulin-induced AQP9 upregulation might favor glycerol influx into hepatocytes.Citation21 Obese patients with T2D exhibit a decreased hepatic AQP9, compatible with a compensatory mechanism aimed at reducing the glycerol entry into hepatocytes and further enhancing the development of hyperglycemia.Citation21,Citation42,Citation43
Non-alcoholic fatty liver disease (NAFLD) is characterized by TAG accumulation in hepatocytes and ranges from simple fatty liver (steatosis) to non-alcoholic steatohepatitis (NASH) and to cirrhosis (irreversible, advanced scarring of the liver).Citation54 AQP9 has been proposed as a pharmacological therapy for NAFLD-NASH.Citation54 In this sense, our group provided evidence that hepatic AQP9 mRNA was negatively correlated with intrahepatic lipid content in obese patients.Citation21 Nevertheless, Miranda and colleagues did not find any relationship between AQP9 expression and the degree of hepatic steatosis or fibrosis in patients with morbid obesity.Citation43 In line with this observation, no apparent histological abnormalities were shown in hepatic sections of transgenic Aqp9 knockout mice.Citation51 These contradictory observations raise some questions as regards the involvement of AQP9 in the etiopathology of NAFLD-NASH and deserve future studies to outline its potential contribution. Hepatocellular ballooning is one of the histological hallmarks of alcoholic steatohepatitis, while it is much less common in NASH. Acetaldehyde, an oxidation product derived from ethanol by the action of the hepatic enzyme alcohol dehydrogenase, induces a rapid increase in glycerol uptake and cell size in rat hepatocytes via AQP9, but prolonged exposure to acetaldehyde reduces AQP9 expression, suggesting a negative feedback regulation.Citation55 Exposure to ethanol, in spite of not modifying AQP9 expression, enhances the activity of GK and PEPCK, leading to increased formation of glycerol-3-phosphate, thereby contributing to alcoholic fatty liver.Citation55
Pancreatic Insulin Secretion (AQP7)
Circulating glucose concentrations constitute the most physiologically relevant influence on the regulation of pro-insulin biosynthesis and insulin secretion in pancreatic β-cells.Citation56 Nonetheless, glycerol is also metabolized in the pancreas and shows the potential to stimulate insulin production and secretion.Citation57 AQP7 seems to embody the unique aquaglyceroporin that mediates the rapid entry of glycerol into β pancreatic cells of mice and rats, being localized in pancreatic islets but not in the pancreatic ducts or the acinar pancreas.Citation58–Citation60 The increase in intracellular glycerol and the consequent activation of GK activity, in turn, stimulate the pro-insulin mRNA and insulin secretion, probably through their participation in the glycolysis and glycerol-phosphate shuttle activities in β cells ().Citation57,Citation58 The glycerol-3-phosphate shuttle pathway constitutes a mechanism that allows the NADH synthesized in the cytosol by glycolysis to contribute to the oxidative phosphorylation pathway in the mitochondria to generate ATP.Citation19 Two enzymes are mainly involved in this shuttle, the cytosolic and the mitochondrial glycerol-3-phosphate dehydrogenases (GPD).Citation61 The cytosolic GPD converts dihydroxyacetone phosphate (DHAP) to glycerol-3-phosphate by oxidizing one molecule of NADH to NAD+. In turn, glycerol-3-phosphate can be reconverted to DHAP by the mitochondrial GPD by reducing one molecule of FAD+ to FADH2. The electrons from FADH2 feed into the oxidative phosphorylation pathway at coenzyme Q, thereby producing ATP. The raise in the cytoplasmic ATP/ADP ratio produced during glycolysis and the glycerol-phosphate shuttle closes the KATP channels and depolarizes the plasma membrane, allowing the opening of voltage-dependent calcium channels and a rapid influx of Ca2+.Citation56,Citation60,Citation62 The consequent increase in cytosolic Ca2+ triggers the exocytosis of insulin-containing secretory granules.Citation60 In this sense, Aqp7-deficient mice show elevated glycerol content and increased GK activity in β cells, thereby promoting an increase in islet TAG levels.Citation58 Moreover, Aqp7-knockout mice show hyperinsulinemia and increased pancreatic insulin-1 and insulin-2 transcript levels.Citation38,Citation58 In addition, Aqp7 deficiency is associated with higher basal and high glucose-induced insulin secretion, probably as a consequence of the increased pancreatic intracellular glycerol content.Citation38,Citation58 Interestingly, the increased insulin secretion of Aqp7-deficient mice is accompanied by a reduction in the islet cell number and total β cell mass, indicating a more efficient insulin biosynthesis and secretion in these cells.
Diabetes occurs when there is inadequate insulin production in response to the body's demand for the hormone.Citation56 Type 1 diabetes is associated with absolute insulin deficiency, which occurs when β cells are destroyed by autoimmunity. In insulin-resistant states, such as obesity and type 2 diabetes, expansion of the β-cell mass occurs in response to the increased demand, but diabetes does not occur unless there is concomitant β-cell dysfunction.Citation56 AQP7, which controls the pancreatic intracellular glycerol content, appears to play a key role in regulating pro-insulin biosynthesis and insulin secretion.Citation58–Citation60 Human obesity and obesity-associated T2D have been associated with an altered gene expression profile of AQP7 in insulin-sensitive tissues, such as adipose tissue and liver.Citation21,Citation40–Citation43 Therefore, further studies analyzing the expression or regulation of AQP7 in pancreatic β cells are needed to shed light on its contribution to the development of T2D.
Energy Production in Skeletal Muscle and Heart (AQP7)
Glycerol levels in the interstitial fluid of human skeletal muscle are much higher than plasma levels and approach the concentrations found in adipose tissue.Citation63 AQP7 is the aquaglyceroporin responsible for glycerol uptake in murine type 2 myofibers,Citation33 while both AQP3 and AQP7 constitute the glycerol gateways in human type I and type II myofibers.Citation64,Citation65 Glycerol is converted to glycerol-3-phosphate by GK and it is readily used for TAG synthesis, but it can also be diverted to form glycolytic intermediates that are, in turn, converted to glycogen or lactate.Citation63 Given the elevated glycerol levels in the interstitial fluid, it seems plausible that changes in aquaglyceroporins and/or GK activity in skeletal muscle may exert important influences on fuel deposition in this tissue.
The heart requires FFA and glucose for energy production in order to maintain its contractility.Citation66 The oxidation of FFA supplies 60–90% of myocardial ATP, while glucose and lactate provide 10–40% of the cardiac energy production.Citation19,Citation66 Interestingly, it has been recently described that the murine heart also utilizes glycerol as energy substrate via the glycerol-3-phosphate shuttle pathway, a mechanism that allows the NADH synthesized in the cytosol by glycolysis to contribute to the oxidative phosphorylation pathway in the mitochondria to generate ATP for the contraction of myofilaments ().Citation19 The mitochondrial creatine kinase catalyzes the reaction transforming ATP and creatine into phosphocreatine and ADP.Citation66 Phosphocreatine rapidly diffuses from the mitochondria to the myofibrils, where myofibrillar creatine kinase catalyzes the restauration of ATP from phosphocreatine. ATP is used by myofibrillar ATPase and other ATP-consuming reactions, such as sarcolemmal and sarcoplasmic reticulum pumps to carry out the contractile work.
AQP7 is apparently the sole glycerol facilitator in the murine heart, and it is localized in cardiac capillaries, fibroblasts and cardiomyocytes.Citation19,Citation67 Plasma glycerol is introduced into cardiomyocytes by AQP7 and converted to glycerol-3-phosphate by the enzymatic activity of GK (). The subsequent entrance into the glycerol-3-phosphate shuttle will lead to increased ATP production and the contraction of myofibrils. In this sense, it has been shown that transgenic mice lacking Aqp7 gene show reduced cardiac glycerol content, lower expression of enzymes related to the glycerol-3-phosphate shuttle, such as GK and GPD2, as well as lower intracellular ATP levels, suggesting a possible disturbance of cardiac glycerol metabolism and energy production.Citation19 Surprisingly, under basal conditions, Aqp7-knockout mice develop normal cardiac phenotypes. However, Aqp7-deficient mice develop cardiac hypertrophy and defective left ventricular contraction following an isoproterenol challenge and subsequently, exhibit a higher mortality than wild-type counterparts, which suggests an impaired myocardial adaptation to pressure overload.Citation19,Citation68 In humans, the adipose tissue constitutes the major expression site of AQP7,Citation69 but Sjöholm and colleagues showed that the heart displays the second tissue with highest expression of AQP7, both by microarray and real-time PCR analyses.Citation70 Therefore, further studies are needed to better assess the pathophysiological relevance of cardiac AQP7 in humans.
Human Genetic Studies
The functional importance of glycerol channels in mammalian physiology and pathophysiology has been extensively studied by analyzing the phenotype of transgenic knockout mice lacking different aquaglyceroporins.Citation10,Citation11 Until now, information regarding the role of aquaglyceroporins in humans has been scarce. The rare cases of homozygous mutations in the coding region of the AQP3 and AQP7 genes identified so far do not resemble the same evident phenotypic characteristics observed in mice, since they were neither diabetic nor obese.Citation29,Citation30 In the Japanese population, the frequency of missense mutations (R12C, V59L, G264V) and silence mutations (A103A, G250G) of AQP7 was not associated with obesity or T2D, despite the absence of glycerol transport in a subject carrying the G264V mutation.Citation29 Nevertheless, Ceperuelo-Mallafré and colleagues reported one case homozygous for the G264V mutation with overweight and T2D.Citation71
The detection of several single nucleotide polymorphisms (SNPs) within the genes encoding aquaglyceroporins also represents an important approach to investigate their cardiometabolic effects. One single variation (G105C) in the AQP3 gene was found in 11 patients (32%) with Menière's disease, an episodic cochleovestibular dysfunction of unknown etiology.Citation72 Prudente and colleagues identified one SNP (A953G) in the promoter region of the AQP7 gene, resulting in an impaired ability to bind the CCAAT/enhancer binding protein β (C/EBPβ) transcription factor.Citation41 Furthermore, it was shown that the AQP7 gene expression was lower in adipose tissue of obese subjects. In addition, the A953G variant in the AQP7 promoter was associated with T2D in 977 Caucasians.Citation41 Recently, an intronic A/T (rs2414539) SNP in the AQP9 gene has been associated with femoral neck bone mineral density in postmenopausal women and may represent one of the susceptibility genes for phenotypes related to bone mass.Citation73
Finally, altered gene and protein expression levels of aquaglyceroporins have been reported in several human diseases. To date, the impaired coordination of adipose and hepatic aquaglyceroporins have been extensively studied in human obesity and obesity-associated T2D.Citation20,Citation21,Citation40–Citation43 A strong increase in AQP9 transcripts was observed in synovial tissues from patients with osteoarthritis and rheumatoid arthritis, suggesting its relation to the pathogenesis of hydrarthrosis and inflammatory synovitis.Citation74 Interestingly, AQP3 mRNA levels are highly expressed in several human cancer cell types, including skin, esophageal, lingual, lung, colorectal and gastric carcinomas.Citation75–Citation79 In this regard, AQP3 appears to play a critical role in basal and epidermal growth factor (EGF)-induced cancer cell migration and proliferation.Citation77,Citation80
Conclusion
In summary, given the versatile functions of aquaglyceroporins, additional and unexpected roles of these glycerol channels are sure to emerge in the coming years. Likewise, further investigations in novel mutations, SNPs or differential expression levels of aquaglyceroporins are needed to broaden our understanding of the implications of these glycerol pores in the onset of human diseases.
Abbreviations
Akt | = | protein kinase B |
AQP | = | aquaporin |
CD36 | = | fatty acid translocase |
DHAP | = | dihydroxyacetone phosphate |
FABP | = | fatty acid binding protein |
FATP | = | fatty acid transporter protein |
FFA | = | free fatty acids |
GK | = | glycerol kinase |
GLUT | = | glucose transporter |
GPD | = | glycerol-3-phosphate dehydrogenase |
IRE | = | insulin response elements |
LPL | = | lipoprotein lipase |
mTOR | = | mammalian target of rapamycin |
NAFLD | = | non-alcoholic fatty liver disease |
NASH | = | non-alcoholic steatohepatitis |
PC | = | pyruvate carboxylase |
PEPCK | = | phosphoenolpyruvate carboxykinase |
PI3K | = | phosphatidylinositol-3-kinase |
SNP | = | single nucleotide polymorphism |
T2D | = | type 2 diabetes |
TAG | = | triacylglycerols |
TZD | = | thiazolidinediones |
Figures and Tables
Figure 1 Proposed role of aquaglyceroporins in lipolysis and lipogenesis. (A) Immunohistochemical detection of AQP3, AQP7 and AQP9 in omental adipose tissue obtained from obese patients (magnification x400). (B) In response to lipogenic conditions, adipocytes synthesize triacylglycerols from FFA and glycerol-3-phosphate. LPL and fatty acid transporters (FABP, FATP and CD36) facilitate the FFA transport across the membrane. Glycerol-3-phosphate proceeds from: (1) glucose, (2) glycerol from HSL-dependent lipolysis which is phosphorylated by GK or (3) AQP9-mediated-glycerol uptake. Lipolytic stimuli are characteristically embodied by stimulation of adrenergic receptors by catecholamines, leading to the translocation of HSL to the lipid droplets and its activation as well as to a parallel translocation of AQP3 and AQP7 to the plasma membrane to facilitate the glycerol release. Interestingly, leptin and catecholamines downregulate AQP7 expression, suggesting a negative feedback regulation in lipolytic states to restrict glycerol release from adipocytes. CM, chylomicron; CD, fatty acid translocase; FFA, free fatty acids; FABP, fatty acid binding protein; FATP, fatty acid transporter protein; GK, glycerol kinase; GLUT4, glucose transporter 4; HSL, hormone sensitive lipase; LPL, lipoprotein lipase; TAG, triacylglycerols; VLDL, very low density lipoprotein.
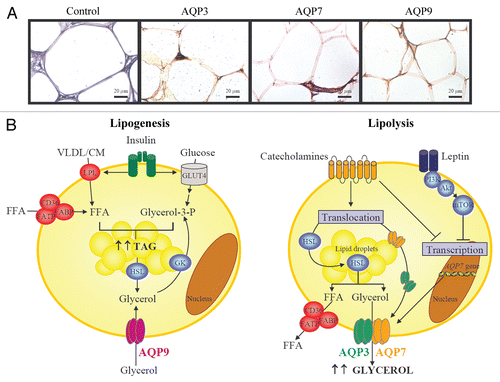
Figure 2 Participation of AQP9 in hepatic gluconeogenesis and steatosis. During fasting, adipocytes induce glycerol release through AQP3 and AQP7 while hepatocytes favor glycerol uptake through AQP9. Glycerol is phosphorylated by GK to produce glycerol-3-phosphate, a precursor of gluconeogenesis and a direct source of glycerol-3-phosphate for de novo synthesis of triacylglycerols. Coordinated regulation of adipose and hepatic aquaglyceroporins is necessary to maintain a correct balance between fat accumulation, hepatic gluconeogenesis and steatosis. FFA, free fatty acids; GK, glycerol kinase; GLUT, glucose transporter; PC, pyruvate carboxylase; PEP, phosphoenolpyruvate; PEPCK, phosphoenolpyruvate carboxykinase; TAG, triacylglycerols.
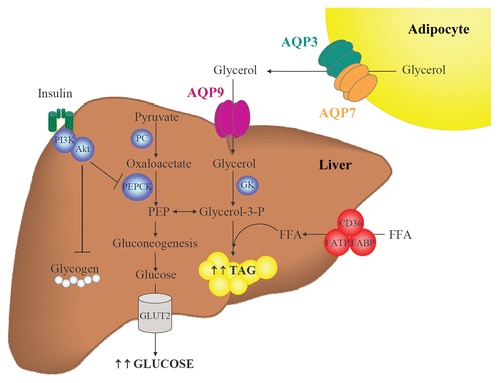
Figure 3 Pancreatic AQP7, glycerol phosphate shuttle and insulin secretion. AQP7 mediates glycerol uptake in pancreatic β cells. Glycerol is phosphorylated to glycerol-3-phosphate by GK and, subsequently, glycerol-3-phosphate is converted to DHAP by the mitochondrial GPD. This reaction reduces equivalents into the mitochondrion for use in oxidative phosphorylation and is called the glycerol-3-phosphate shuttle. The increase in intracellular ATP:ADP ratio induces the closure of ATP-sensitive K+ channels, the cell membrane depolarization and the opening of voltage-sensitive Ca2+ channels. The raise in intracellular Ca2+ enhances the exocytosis of insulin-containing granules. GK, glycerol kinase; GLUT, glucose transporter; GPD, glycerol-3-phosphate dehydrogenase.
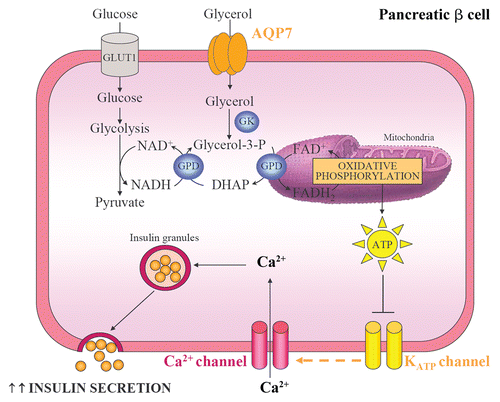
Figure 4 Cardiac AQP7, the glycerol phosphate shuttle and ATP production. AQP7 facilitates glycerol uptake by cardiomyocytes. Glycerol is phosphorylated to glycerol-3-phosphate by GK that enters in the glycerol-3-phosphate shuttle to trigger ATP production in the mitochondrial oxidative phosphorylation. The transfer of ATP to the cytosol is achieved by the creatine kinase energy shuttle and is used for the contraction of myofilaments. DHAP, dihydroxyacetone phosphate; GK, glycerol kinase; GPD, glycerol-3-phosphate dehydrogenase.
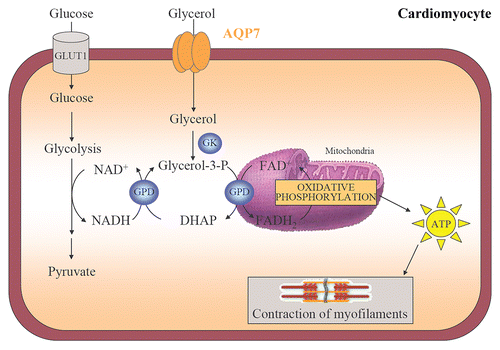
Acknowledgements
This work was funded by the Instituto de Salud Carlos III (FIS PS09/02330 and PI10/01677). CIBER de Fisiopatología de la Obesidad y Nutrición (CIBERobn) is an initiative of the Instituto de Salud Carlos III, Spain.
References
- Frühbeck G, Gómez-Ambrosi J. Rationale for the existence of additional adipostatic hormones. FASEB J 2001; 15:1996 - 2006
- Rodríguez A, Catalán V, Gómez-Ambrosi J, Frühbeck G. Visceral and subcutaneous adiposity: are both potential therapeutic targets for tackling the metabolic syndrome?. Curr Pharm Des 2007; 13:2169 - 2175
- Kuk JL, Katzmarzyk PT, Nichaman MZ, Church TS, Blair SN, Ross R. Visceral fat is an independent predictor of all-cause mortality in men. Obesity 2006; 14:336 - 341
- Yusuf S, Hawken S, Ounpuu S, Bautista L, Franzosi MG, Commerford P, et al. Obesity and the risk of myocardial infarction in 27,000 participants from 52 countries: a case-control study. Lancet 2005; 366:1640 - 1649
- Eckel RH, Grundy SM, Zimmet PZ. The metabolic syndrome. Lancet 2005; 365:1415 - 1428
- Fujioka S, Matsuzawa Y, Tokunaga K, Kawamoto T, Kobatake T, Keno Y, et al. Improvement of glucose and lipid metabolism associated with selective reduction of intra-abdominal visceral fat in premenopausal women with visceral fat obesity. Int J Obes 1991; 15:853 - 859
- Gómez-Ambrosi J, Pastor C, Salvador J, Silva C, Rotellar F, Gil MJ, et al. Influence of waist circumference on the metabolic risk associated with impaired fasting glucose: effect of weight loss after gastric bypass. Obes Surg 2007; 17:585 - 591
- Maeda N, Funahashi T, Shimomura I. Metabolic impact of adipose and hepatic glycerol channels aquaporin 7 and aquaporin 9. Nat Clin Pract Endocrinol Metab 2008; 4:627 - 634
- Hibuse T, Maeda N, Nagasawa A, Funahashi T. Aquaporins and glycerol metabolism. Biochim Biophys Acta 2006; 1758:1004 - 1011
- King LS, Kozono D, Agre P. From structure to disease: the evolving tale of aquaporin biology. Nat Rev Mol Cell Biol 2004; 5:687 - 698
- Agre P, Kozono D. Aquaporin water channels: molecular mechanisms for human diseases. FEBS Lett 2003; 555:72 - 78
- Jung JS, Preston GM, Smith BL, Guggino WB, Agre P. Molecular structure of the water channel through aquaporin CHIP. The hourglass model. J Biol Chem 1994; 269:14648 - 14654
- Jung JS, Bhat RV, Preston GM, Guggino WB, Baraban JM, Agre P. Molecular characterization of an aquaporin cDNA from brain: candidate osmoreceptor and regulator of water balance. Proc Natl Acad Sci USA 1994; 91:13052 - 13056
- Agre P, King LS, Yasui M, Guggino WB, Ottersen OP, Fujiyoshi Y, et al. Aquaporin water channels—from atomic structure to clinical medicine. J Physiol 2002; 542:3 - 16
- Reshef L, Olswang Y, Cassuto H, Blum B, Croniger CM, Kalhan SC, et al. Glyceroneogenesis and the triglyceride/fatty acid cycle. J Biol Chem 2003; 278:30413 - 30416
- Frühbeck G. Obesity: aquaporin enters the picture. Nature 2005; 438:436 - 437
- Frühbeck G, Catalán V, Gómez-Ambrosi J, Rodríguez A. Aquaporin-7 and glycerol permeability as novel obesity drug-target pathways. Trends Pharmacol Sci 2006; 27:345 - 347
- Rodríguez A, Catalán V, Gómez-Ambrosi J, Frühbeck G. Role of aquaporin-7 in the pathophysiological control of fat accumulation in mice. FEBS Lett 2006; 580:4771 - 4776
- Hibuse T, Maeda N, Nakatsuji H, Tochino Y, Fujita K, Kihara S, et al. The heart requires glycerol as an energy substrate through aquaporin 7, a glycerol facilitator. Cardiovasc Res 2009; 83:34 - 41
- Miranda M, Escote X, Ceperuelo-Mallafre V, Alcaide MJ, Simon I, Vilarrasa N, et al. Paired subcutaneous and visceral adipose tissue aquaporin-7 expression in human obesity and type 2 diabetes: differences and similarities between depots. J Clin Endocrinol Metab 2010; 95:3470 - 3479
- Rodríguez A, Catalán V, Gómez-Ambrosi J, García-Navarro S, Rotellar F, Valentí V, et al. Insulin- and leptin-mediated control of aquaglyceroporins in human adipocytes and hepatocytes is mediated via the PI3K/Akt/mTOR signaling cascade. J Clin Endocrinol Metab 2011; http://dx.doi.org/10.1210/jc.2010-1408
- Maeda N, Funahashi T, Hibuse T, Nagasawa A, Kishida K, Kuriyama H, et al. Adaptation to fasting by glycerol transport through aquaporin 7 in adipose tissue. Proc Natl Acad Sci USA 2004; 101:17801 - 17806
- Zhao FQ, Keating AF. Functional properties and genomics of glucose transporters. Curr Genomics 2007; 8:113 - 128
- Kishida K, Shimomura I, Kondo H, Kuriyama H, Makino Y, Nishizawa H, et al. Genomic structure and insulin-mediated repression of the aquaporin adipose (AQPap), adipose-specific glycerol channel. J Biol Chem 2001; 276:36251 - 36260
- Gonzales AM, Orlando RA. Role of adipocyte-derived lipoprotein lipase in adipocyte hypertrophy. Nutr Metab 2007; 4:22
- Guan HP, Li Y, Jensen MV, Newgard CB, Steppan CM, Lazar MA. A futile metabolic cycle activated in adipocytes by antidiabetic agents. Nat Med 2002; 8:1122 - 1128
- Gradilone SA, Tietz PS, Splinter PL, Marinelli RA, LaRusso NF. Expression and subcellular localization of aquaporin water channels in the polarized hepatocyte cell line, WIF-B. BMC Physiol 2005; 5:13
- Kuriyama H, Shimomura I, Kishida K, Kondo H, Furuyama N, Nishizawa H, et al. Coordinated regulation of fat-specific and liver-specific glycerol channels, aquaporin adipose and aquaporin 9. Diabetes 2002; 51:2915 - 2921
- Kondo H, Shimomura I, Kishida K, Kuriyama H, Makino Y, Nishizawa H, et al. Human aquaporin adipose (AQPap) gene. Genomic structure, promoter analysis and functional mutation. Eur J Biochem 2002; 269:1814 - 1826
- Roudier N, Ripoche P, Gane P, Le Pennec PY, Daniels G, Cartron JP, et al. AQP3 deficiency in humans and the molecular basis of a novel blood group system, GIL. J Biol Chem 2002; 277:45854 - 45859
- Nejsum LN, Kwon TH, Marples D, Flyvbjerg A, Knepper MA, Frokiaer J, et al. Compensatory increase in AQP2, p-AQP2 and AQP3 expression in rats with diabetes mellitus. Am J Physiol Renal Physiol 2001; 280:715 - 726
- Arner P. Differences in lipolysis between human subcutaneous and omental adipose tissues. Ann Med 1995; 27:435 - 438
- Kishida K, Kuriyama H, Funahashi T, Shimomura I, Kihara S, Ouchi N, et al. Aquaporin adipose, a putative glycerol channel in adipocytes. J Biol Chem 2000; 275:20896 - 20902
- Yasui H, Kubota M, Iguchi K, Usui S, Kiho T, Hirano K. Membrane trafficking of aquaporin 3 induced by epinephrine. Biochem Biophys Res Commun 2008; 373:613 - 617
- Walker CG, Holness MJ, Gibbons GF, Sugden MC. Fasting-induced increases in aquaporin 7 and adipose triglyceride lipase mRNA expression in adipose tissue are attenuated by peroxisome proliferator-activated receptor alpha deficiency. Int J Obes 2007; 31:1165 - 1171
- Fasshauer M, Klein J, Lossner U, Klier M, Kralisch S, Paschke R. Suppression of aquaporin adipose gene expression by isoproterenol, TNFa and dexamethasone. Horm Metab Res 2003; 35:222 - 227
- Kong CS, Kim JA, Bak SS, Byun HG, Kim SK. Antiobesity effect of carboxymethyl chitin by AMPK and aquaporin-7 pathways in 3T3-L1 adipocytes. J Nutr Biochem 2011; 22:276 - 281
- Hibuse T, Maeda N, Funahashi T, Yamamoto K, Nagasawa A, Mizunoya W, et al. Aquaporin 7 deficiency is associated with development of obesity through activation of adipose glycerol kinase. Proc Natl Acad Sci USA 2005; 102:10993 - 10998
- Hara-Chikuma M, Sohara E, Rai T, Ikawa M, Okabe M, Sasaki S, et al. Progressive adipocyte hypertrophy in aquaporin-7-deficient mice: adipocyte glycerol permeability as a novel regulator of fat accumulation. J Biol Chem 2005; 280:15493 - 15496
- Marrades MP, Milagro FI, Martínez JA, Moreno-Aliaga MJ. Differential expression of aquaporin 7 in adipose tissue of lean and obese high fat consumers. Biochem Biophys Res Commun 2006; 339:785 - 789
- Prudente S, Flex E, Morini E, Turchi F, Capponi D, De Cosmo S, et al. A functional variant of the adipocyte glycerol channel aquaporin 7 gene is associated with obesity and related metabolic abnormalities. Diabetes 2007; 56:1468 - 1474
- Catalán V, Gómez-Ambrosi J, Pastor C, Rotellar F, Silva C, Rodríguez A, et al. Influence of morbid obesity and insulin resistance on gene expression levels of AQP7 in visceral adipose tissue and AQP9 in liver. Obes Surg 2008; 18:695 - 701
- Miranda M, Ceperuelo-Mallafré V, Lecube A, Hernández C, Chacón MR, Fort JM, et al. Gene expression of paired abdominal adipose AQP7 and liver AQP9 in patients with morbid obesity: relationship with glucose abnormalities. Metabolism 2009; 58:1762 - 1768
- Kishida K, Shimomura I, Nishizawa H, Maeda N, Kuriyama H, Kondo H, et al. Enhancement of the aquaporin adipose gene expression by a peroxisome proliferator-activated receptor γ. J Biol Chem 2001; 276:48572 - 48579
- Lee DH, Park DB, Lee YK, An CS, Oh YS, Kang JS, et al. The effects of thiazolidinedione treatment on the regulations of aquaglyceroporins and glycerol kinase in OLETF rats. Metabolism 2005; 54:1282 - 1289
- Carbrey JM, Gorelick-Feldman DA, Kozono D, Praetorius J, Nielsen S, Agre P. Aquaglyceroporin AQP9: solute permeation and metabolic control of expression in liver. Proc Natl Acad Sci USA 2003; 100:2945 - 2950
- Elkjaer M, Vajda Z, Nejsum LN, Kwon T, Jensen UB, Amiry-Moghaddam M, et al. Immunolocalization of AQP9 in liver, epididymis, testis, spleen and brain. Biochem Biophys Res Commun 2000; 276:1118 - 1128
- Ishibashi K, Kuwahara M, Gu Y, Tanaka Y, Marumo F, Sasaki S. Cloning and functional expression of a new aquaporin (AQP9) abundantly expressed in the peripheral leukocytes permeable to water and urea, but not to glycerol. Biochem Biophys Res Commun 1998; 244:268 - 274
- Ishibashi K, Sasaki S, Saito F, Ikeuchi T, Marumo F. Structure and chromosomal localization of a human water channel (AQP3) gene. Genomics 1995; 27:352 - 354
- Ma T, Song Y, Yang B, Gillespie A, Carlson EJ, Epstein CJ, et al. Nephrogenic diabetes insipidus in mice lacking aquaporin-3 water channels. Proc Natl Acad Sci USA 2000; 97:4386 - 4391
- Rojek AM, Skowronski MT, Fuchtbauer EM, Fuchtbauer AC, Fenton RA, Agre P, et al. Defective glycerol metabolism in aquaporin 9 (AQP9) knockout mice. Proc Natl Acad Sci USA 2007; 104:3609 - 3614
- Higuchi S, Kubota M, Iguchi K, Usui S, Kiho T, Hirano K. Transcriptional regulation of aquaporin 3 by insulin. J Cell Biochem 2007; 102:1051 - 1058
- Postic C, Girard J. The role of the lipogenic pathway in the development of hepatic steatosis. Diabetes Metab 2008; 34:643 - 648
- Widhalm K, Ghods E. Nonalcoholic fatty liver disease: a challenge for pediatricians. Int J Obes 2010; 34:1451 - 1467
- Potter JJ, Koteish A, Hamilton J, Liu X, Liu K, Agre P, et al. Effects of Acetaldehyde on Hepatocyte Glycerol Uptake and Cell Size: Implication of Aquaporin 9. Alcohol Clin Exp Res 2011; http://dx.doi.org/10.1111/j.530-0277.2010.01424.x
- Muoio DM, Newgard CB. Mechanisms of disease: molecular and metabolic mechanisms of insulin resistance and β-cell failure in type 2 diabetes. Nat Rev Mol Cell Biol 2008; 9:193 - 205
- Skelly RH, Wicksteed B, Antinozzi PA, Rhodes CJ. Glycerol-stimulated proinsulin biosynthesis in isolated pancreatic rat islets via adenoviral-induced expression of glycerol kinase is mediated via mitochondrial metabolism. Diabetes 2001; 50:1791 - 1798
- Matsumura K, Chang BH, Fujimiya M, Chen W, Kulkarni RN, Eguchi Y, et al. Aquaporin 7 is a β-cell protein and regulator of intraislet glycerol content and glycerol kinase activity, β-cell mass and insulin production and secretion. Mol Cell Biol 2007; 27:6026 - 6037
- Delporte C, Virreira M, Crutzen R, Louchami K, Sener A, Malaisse WJ, et al. Functional role of aquaglyceroporin 7 expression in the pancreatic β-cell line BRIN-BD11. J Cell Physiol 2009; 221:424 - 429
- Best L, Brown PD, Yates AP, Perret J, Virreira M, Beauwens R, et al. Contrasting effects of glycerol and urea transport on rat pancreatic β-cell function. Cell Physiol Biochem 2009; 23:255 - 264
- Shen W, Wei Y, Dauk M, Tan Y, Taylor DC, Selvaraj G, et al. Involvement of a glycerol-3-phosphate dehydrogenase in modulating the NADH/NAD+ ratio provides evidence of a mitochondrial glycerol-3-phosphate shuttle in Arabidopsis. Plant Cell 2006; 18:422 - 441
- Virreira M, Perret J, Delporte C. Pancreatic β-cells: Role of glycerol and aquaglyceroporin 7. Int J Biochem Cell Biol 2011; 43:10 - 13
- Montell E, Lerin C, Newgard CB, Gomez-Foix AM. Effects of modulation of glycerol kinase expression on lipid and carbohydrate metabolism in human muscle cells. J Biol Chem 2002; 277:2682 - 2686
- Wakayama Y, Jimi T, Inoue M, Kojima H, Shibuya S, Murahashi M, et al. Expression of aquaporin 3 and its localization in normal skeletal myofibres. Histochem J 2002; 34:331 - 337
- Wakayama Y, Inoue M, Kojima H, Jimi T, Shibuya S, Hara H, et al. Expression and localization of aquaporin 7 in normal skeletal myofiber. Cell Tissue Res 2004; 316:123 - 129
- Neubauer S. The failing heart—an engine out of fuel. N Engl J Med 2007; 356:1140 - 1151
- Skowronski MT, Lebeck J, Rojek A, Praetorius J, Fuchtbauer EM, Frokiaer J, et al. AQP7 is localized in capillaries of adipose tissue, cardiac and striated muscle: implications in glycerol metabolism. Am J Physiol Renal Physiol 2007; 292:956 - 965
- Gladka M, El Azzouzi H, De Windt LJ, da Costa Martins PA. Aquaporin 7: the glycerol aquaeductus in the heart. Cardiovasc Res 2009; 83:3 - 4
- Ishibashi K, Yamauchi K, Kageyama Y, Saito-Ohara F, Ikeuchi T, Marumo F, et al. Molecular characterization of human Aquaporin-7 gene and its chromosomal mapping. Biochim Biophys Acta 1998; 1399:62 - 66
- Sjöholm K, Palming J, Olofsson LE, Gummesson A, Svensson PA, Lystig TC, et al. A microarray search for genes predominantly expressed in human omental adipocytes: adipose tissue as a major production site of serum amyloid A. J Clin Endocrinol Metab 2005; 90:2233 - 2239
- Ceperuelo-Mallafré V, Miranda M, Chacón MR, Vilarrasa N, Megia A, Gutiérrez C, et al. Adipose tissue expression of the glycerol channel aquaporin-7 gene is altered in severe obesity but not in type 2 diabetes. J Clin Endocrinol Metab 2007; 92:3640 - 3645
- Candreia C, Schmuziger N, Gurtler N. Molecular analysis of aquaporin genes 1 to 4 in patients with Meniere's disease. Cell Physiol Biochem 2010; 26:787 - 792
- Chanprasertyothin S, Saetung S, Rajatanavin R, Ongphiphadhanakul B. Genetic variant in the aquaporin 9 gene is associated with bone mineral density in postmenopausal women. Endocrine 2010; 38:83 - 86
- Nagahara M, Waguri-Nagaya Y, Yamagami T, Aoyama M, Tada T, Inoue K, et al. TNFalpha-induced aquaporin 9 in synoviocytes from patients with OA and RA. Rheumatology 2010; 49:898 - 906
- Verkman AS, Hara-Chikuma M, Papadopoulos MC. Aquaporins—new players in cancer biology. J Mol Med 2008; 86:523 - 529
- Liu YL, Matsuzaki T, Nakazawa T, Murata S, Nakamura N, Kondo T, et al. Expression of aquaporin 3 (AQP3) in normal and neoplastic lung tissues. Hum Pathol 2007; 38:171 - 178
- Huang Y, Zhu Z, Sun M, Wang J, Guo R, Shen L, et al. Critical role of aquaporin-3 in the human epidermal growth factor-induced migration and proliferation in the human gastric adenocarcinoma cells. Cancer Biol Ther 2010; 9:1000 - 1007
- Melis M, Hernandez J, Siegel EM, McLoughlin JM, Ly QP, Nair RM, et al. Gene expression profiling of colorectal mucinous adenocarcinomas. Dis Colon Rectum 2010; 53:936 - 943
- Kusayama M, Wada K, Nagata M, Ishimoto S, Takahashi H, Yoneda M, et al. Critical role of aquaporin 3 on growth of human esophageal and oral squamous cell carcinoma. Cancer Sci 2011; http://dx.doi.org/10.1111/j.349-7006.2011.01927.x
- Ji C, Cao C, Lu S, Kivlin R, Amaral A, Kouttab N, et al. Curcumin attenuates EGF-induced AQP3 upregulation and cell migration in human ovarian cancer cells. Cancer Chemother Pharmacol 2008; 62:857 - 865