Abstract
Pannexins (Panxs) are a multifaceted family of ion and metabolite channels that play key roles in a number of physiological and pathophysiological settings. These single membrane large-pore channels exhibit a variety of tissue, cell type, and subcellular distributions. The lifecycles of Panxs are complex, yet must be understood to accurately target these proteins for future therapeutic use. Here we review the basics of Panx function and localization, and then analyze the recent advances in knowledge regarding Panx trafficking. We examine several intrinsic features of Panxs including specific post-translational modifications, the divergent C-termini, and oligomerization, all of which contribute to Panx anterograde transport pathways. Further, we examine the potential influence of extrinsic factors, such as protein-protein interactions, on Panx trafficking. Finally, we highlight what is currently known with respect to Panx internalization and retrograde transport, and present new data illustrating Panx1 internalization following an activating stimulus.
Introduction
Pannexins (Panxs) are emerging as key proteins in a number of clinical settings. As complex multimeric proteins that have the potential to act as powerful signaling effectors, Panxs represent exciting putative drug targets. In order to develop appropriate and effective strategies to target Panxs for future therapeutic use, it is important for us to understand the complexities of their lifecycles. Work by several groups has begun to reveal the multifaceted picture of structural elements intrinsic to Panxs that regulate their precise trafficking. Here we cover the essential basics of Panx structure-function and then expand on recent advances in Panx trafficking, including Panx post-translational modification, the role of the C-terminus, the potential impact of oligomerization and emerging insights into internalization. Further, we highlight the putative importance of each factor in anterograde and/or retrograde trafficking pathways. We also examine new data that has the potential to enhance our understanding of how extrinsic factors, such as crosstalk with other proteins and signaling molecules, impact on Panx trafficking and therefore function.
Panx basics
Panxs were originally described as putative gap-junction proteins by virtue of their homology to innexins, the gap junction-forming proteins in invertebrates.Citation1 To date, three Panx family members have been identified: Pannexin 1 (Panx1), Pannexin 2 (Panx2), and Pannexin 3 (Panx3). Each Panx subunit contains four-transmembrane domains, two extracellular loops, and three intracellular domains, including an N-terminus, intracellular loop, and highly divergent C-terminus. Panx subunits assemble into multimers to form channels.Citation2 The current consensus in the field is that Panxs form large-pore, single membrane channels (rather than intercellular channels), which act as a conduit for ions and small molecules such as ATP (as recently reviewed in ref. Citation3).
Most of what is known about the function of Panxs has been garnered from studies on plasma membrane Panx1. Here Panx1 is known to function as an ATP release channel in a variety of cell types, mediating autocrine and paracrine communication. Under physiological conditions, Panx1 channels are reportedly activated by mechanical stretch,Citation3 strong and prolonged depolarizations,Citation4, Citation5 elevated extracellular potassium,Citation6 and elevated intracellular calcium.Citation7 Panx1 channels are also activated by pathological stimuli, such as oxygen and glucose deprivation,Citation8, Citation9 seizure-related NMDAR receptor activation,Citation6, Citation10 and inflammation.Citation11
Pannexin Distribution at the Tissue and Cellular Level
Not only are Panxs present in multiple subcellular compartments within a given cell type, their subcellular distributions vary across diverse cell and tissue types. What is known about Panx expression in different tissues and organs throughout the body? Panx1 mRNA and protein are ubiquitously expressed in a wide variety of tissues (eye, thyroid, prostate, liver, and kidneys) and is highly expressed in central nervous system.Citation12 Based on northern blot analysis, Panx2 mRNA is also particularly enriched in the central nervous system, relative to other tissue.Citation13 Panx3 mRNA and protein are expressed primarily in skin, chondrocytes, and osteoblasts.Citation14-Citation16 While there is some information on the relative mRNA and protein levels of different Panxs over the course of embryonic development and postnatal life, the extent of co-distribution of different Panxs at these critical stages remains lacking for many tissues.
Panx Distribution of the Subcellular Level
In addition to Panxs at the cell surface, which have dominated functional studies, Panxs are also detected in intracellular compartments of diverse cell types, including neural cellsCitation17, Citation18 keratinocytes,Citation16, Citation19 prostate adenocarcinoma cells,Citation20 chondrocytes,Citation14 and differentiated osteoblasts.Citation15 In contrast with Panx1 and Panx3, which are readily found at the plasma membrane, the contrarian Panx2 is actually primarily localized to intracellular compartments, with limited plasma membrane expression having been noted only in overexpression systemsCitation21 and some neuronal cell types.Citation18 Little is known regarding the function of intracellular Panxs. Evidence suggests that a sub-population of Panxs present at the endoplasmic reticulum (ER) function as Ca2+-leak channels (reviewed in ref. Citation25). These ER resident Panxs have been implicated in various aspects of tumor cell proliferation as well as neuronal and osteoblast differentiation;Citation15, Citation18 however, more work is clearly required to fully comprehend the functional importance of intracellular Panxs.
Anterograde Trafficking of Panx1
Much of the recent work on Panx trafficking focuses on anterograde transport; the process by which proteins transit from the endoplasmic reticulum (ER), through the Golgi apparatus, and ultimately to the plasma membrane (). Several intrinsic structural elements are known to regulate this complex pathway including asparagine residues that are post-translationally modified elements within the C-termini, and oligomerization. Because most of what is understood about Panx function comes from cell surface populations, it is critical to understand the precise signaling mechanisms that regulate anterograde movement to this location.
Figure 1. Mechanisms regulating the anterograde and retrograde trafficking of Panxs. (A) Anterograde trafficking of Panx1 begins in the endoplasmic reticulum (ER), where it receives a post-translational modification (PTM) in the form of a high-mannose glycan to asparagine 254 (N254) in its second extracellular loop. This forms the high mannose glycosylation species of Panx1 (Gly1). The Gly1 Panx1 species is transported to the Golgi through anterograde COPII vesicle-mediated transport. Here the high mannose N-linked glycan is modified to a complex glycosylation (Gly2). Gly2 glycosylation is necessary for subsequent plasma membrane localization of Panx1. Once at the cell surface, an interaction between the Panx1 C-terminus and filamentous actin (F-actin) is critical for stability at the plasma membrane. The intrinsic and extrinsic cues for retrograde trafficking remain poorly understood (denoted by ‘?’), however there is experimental evidence for Panx1 degradation within endolysosomal compartments. (B) The anterograde trafficking of Panx2 begins in the endoplasmic reticulum. Here, Panx2 receives a high-mannose glycan at an unknown amino acid locus. Panx2 is not glycosylated further and distributes primarily to intracellular compartments, such as the endolysosome, with evidence of cell type-specific plasma membrane localization. The mechanisms controlling anterograde and retrograde Panx2 trafficking remain unknown. (C) Anterograde trafficking of Panx3 also begins in the ER, where it is glycosylated at asparagine 71 (N71) in its first extracellular loop, forming a high mannose glycosylation species (Gly1). Like Panx1, Panx3 is trafficked to the Golgi in COPII transport vesicles, where it is modified to a mature, complex glycosylation species (Gly2). Gly2 glycosylation is necessary for trafficking of Panx3 to the plasma membrane. The internalization and retrograde transport mechanisms remain unknown, however based on endolysosomal localizations of the other Panx family members, Panx3 may also traffic in retrograde to these compartments.
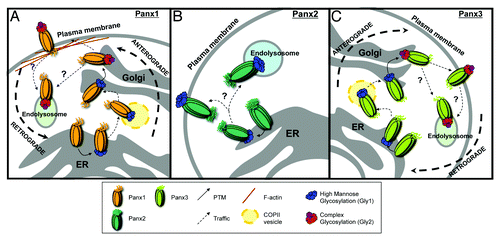
Impact of N-linked glycosylation on Panx trafficking
A combination of bioinformatics tools and site-directed mutagenesis have been used to reveal the critical role that glycosylation plays in Panx localization. Glycosylation of Panx1 and Panx3 at specific asparagine residues during transit between the ER and Golgi occurs during their anterograde trafficking and is required for subsequent cell surface expression.Citation22-Citation24 It was initially thought that only Panx1 and Panx3 were glycosylated;Citation22 however, Panx2 is also glycosylated, albeit to a less complex degree.Citation21 These differences in glycosylation among Panx family members likely account, in part, for the diverse subcellular distributions noted across different Panxs.
Panx1 and Panx3 glycosylation-deficient mutants are retained in intracellular compartments.Citation22-Citation24 Interestingly, Panx1 is glycosylated in the second extracellular loop (asparagine 254; N254), while Panx3 is glycosylated in the first extracellular loop (N71).Citation22 The maturation of Panx1/Panx3 glycosylation proceeds through three species detectable by their differences in molecular weight observed on western blots: Gly0, an ER resident pre-glycosylated form, Gly1, a high-mannose glycosylated form, and Gly2, the mature complex glycosylation species that is able to reach the plasma membrane.Citation22,Citation23 As might be anticipated, disrupting Golgi formation or inhibiting ER to Golgi trafficking prevents formation of the Panx1 and Panx3 Gly2 species.Citation25 Unlike Panx1 and Panx3, Panx2 exists exclusively as a pre-glycosylated form, Gly0, and a high-mannose glycosylation species, Gly1.Citation21 As of yet, there is no evidence for a fully mature, complex glycosylation species of Panx2 that is equivalent to the Panx1/Panx3 Gly2 species. Perhaps unsurprisingly, the majority of Panx2 is located in intracellular membranes.Citation18,Citation26 The factors regulating its trafficking to the plasma membrane in neurons have not yet been fully elucidated, but evidence suggests that another post-translational modification, palmitoylation, could be involved.Citation18
In summary, the diverse glycosylation profile of Panxs appears to have important implications for subcellular distribution (), especially through anterograde trafficking pathways. Therefore any physiological or pathophysiological process affecting glycosylation of Panxs will likely impact Panx distribution, and thus function. What regulates this process? New evidence suggests that Panx glycosylation is regulated in a tissue and cell type dependent manner,Citation22 which could account, in part, for the diverse subcellular distributions of a given Panx family member observed across different tissues and cell types. Although glycosylation enzymes exhibit diverse cell and tissue expression patterns,Citation27,Citation28 a correlation with the cell and tissue differences in Panx localization has not yet been identified. In pathophysiological scenarios, such as stroke, alterations to post-translational modification machinery could also impact on glycosylation.Citation28 Therefore, while the importance of glycosylation in Panx anterograde transport is becoming clear, more work is required to understand the mechanisms controlling this post-translational modification and resulting alterations to Panx localizations.
Role of the Panx C-termini in trafficking
Intracellular C-terminal domains of many plasma membrane channels and receptors are known to play key roles in protein trafficking; as such, divergence in sequence and/or post-translational modification of these domains often results in dramatic alterations to protein localization.Citation29-Citation33 While the Panx C-termini are emerging as critical structural features in trafficking, further research is required to fully understand their complex roles. The C-termini are the most dissimilar among Panxs in terms of sequence homology and length.Citation34 They therefore enable divergence in post-translational modification, protein-protein interactions, and oligomerization, which can allow for complex fine-tuning of Panx localization.
Again among the Panxs, Panx1 has been the primary focus of study in terms of the role of the C-terminus in trafficking. Recent work has demonstrated that this region is vital for plasma membrane localization. A Panx1 C-terminal mutant truncated at threonine 307 accumulates in intracellular compartments.Citation35 Specifically, truncated Panx1 partially localizes to the ER, and is unable to become the fully mature Gly2 species. Furthermore, this mutant is aberrantly targeted to the proteasome for degradation, likely due to impaired protein folding and oligomerization.Citation35 This differs from wildtype Panx1, which first traffics through anterograde pathways to the cell surface and subsequently in retrograde to endolysosomal compartments for degradation ().Citation35 Another study by Sandilos and colleagues examined the effects of different C-terminal truncation mutants on channel function, however the role of these mutants on Panx1 trafficking was not addressed.Citation36 Interestingly, endogenous C-terminal truncations of Panx1 exist, as a portion of the C-terminus is actually cleaved following activation of caspases 3/7.Citation37 While functional studies have examined the role of this endogenous truncation in channel function,Citation36-Citation38 its putative impact on Panx1 trafficking has yet to be determined.
Moreover, our own group recently developed a Panx2-Panx1 chimeric protein consisting of the Panx1 C-terminus fused to the channel portion of Panx2 to further analyze the role of Panx C-termini in their trafficking.Citation17 In neuroblastoma cells, the chimeric Panx protein exhibits diffuse intracellular localization compared with the predominantly plasma membrane expression of Panx1, and the endolysosomal localization of Panx2 (). Thus, the Panx1 C-terminus disrupts normal Panx2 localization, but is insufficient to re-direct the Panx2 pore to the plasma membrane. While these studies confirm the importance of C-termini for channel trafficking, they imply that C-terminal elements act in concert with additional targeting signals, such as complex glycosylation, to effectively traffic Panxs to their proper localizations.
Emerging evidence has also linked Panx C-termini to associations with the cytoskeleton,Citation25,Citation39 a vital subcellular network for protein trafficking and stabilization.Citation40 In fact, the C-terminus of Panx1 has been shown to directly interact with actin, the major component of the microfilament cytoskeleton,Citation41 in both overexpression and endogenous systems.Citation25,Citation39 Moreover, Bhalla-Gehi et al. identified a dependence on actin microfilaments for proper cell surface trafficking and stabilization of Panx1 (). Microfilament disruption caused intracellular accumulation and reduction in the mobility of both plasma membrane and vesicular populations of Panx1.Citation25
Overall, Panx C-termini are emerging as vital components for proper subcellular targeting. They not only contain necessary intrinsic signaling sequences, but also regulate extrinsic trafficking factors, including important protein-protein interactions. Moreover, caspase cleavage of Panx C-termini may contribute an additional level of control to Panx localization. Thus the C-termini are likely critical for both anterograde and retrograde movements of Panx channels.
Role of oligomerization and Panx intermixing on localization
The potential for Panx hetero-oligomerization or physical interaction between homomeric channels could also influence Panx localization. Current conceptualization of Panx oligomerization is that they exist as homomers, but some evidence suggests Panx1 heteromerization could occur when expressed in the same cell type. For example, in overexpression systems, Panx1 co-precipitates with Panx2 and Panx3,Citation21,Citation42 which could be a result of either heteromerization or physical interaction between homomeric channels.Citation2 Moreover, co-expression of different family members alters the trafficking patterns of typical Panx channels. For instance, when co-expressed with Panx1 in mammalian cells, both Panx2 and Panx3 show increased plasma membrane localization.Citation21 Interestingly, there is a reduction in Panx1 current amplitude in the presence of Panx2 in Xenopus oocytes,Citation43 suggesting trafficking crosstalk between Panxs could be cell type dependent (i.e., dependent on the expression of signaling systems unique to specific cell types). Co-expression of Panx1 in mammalian cells with a glycosylation-deficient Panx3 mutant, or vice versa, rescues plasma membrane expression of the mutant protein.Citation21 This evidence suggests a role especially for Panx1 itself in modulating the anterograde transit patterns of other Panxs.
Does Panx subunit intermixing occur endogenously?
The potential formation of Panx1/Panx2 heteromeric channels is particularly intriguing and potentially functionally significant. For example, the reduction in Panx1 current amplitude in the presence of Panx2 that was observed by Bruzzone et al. (referred to in the preceding section) could be explained by heteromerization-dependent changes in channel function. However, work from the Sosinsky lab suggests that Panx1 and Panx2 proteins likely have differing oligomeric symmetry and subunit sizes,Citation2 and that Panx1/Panx2 heteromers are unstable over time.Citation2 Further, no evidence for their endogenous formation exists as of yet. Moreover, it is not yet known whether cell populations in the nervous system co-express Panx1 and Panx2 protein, which would be required for the potential physical and functional intermixing in the nervous system. Therefore, there is potential for endogenous intermixing of Panx1/Panx2, but there are still major gaps in our knowledge of pannexins that need to be overcome.
The relevance of understanding the relationship and potential for crosstalk between Panx1 and Panx2 was recently reinforced by a stroke study from Bargiotas and colleagues. Their work revealed a relationship between Panx1 and Panx2 in stroke-induced dye uptake and infarct volume, as well as functional recovery following stroke. Only Panx1/Panx2 double knockout mice presented increased functional outcomeCitation9,Citation44 and reduced infarct sizeCitation9 after stroke, when compared with wild-type animals; whereas, single knockouts were statistically indistinguishable from wild type. These results suggest that Panx1 and Panx2 can functionally compensate for one another (and alone have sub-threshold deleterious effects), or, that the individual contributions to the impairment of cell survival are additive via a physical or functional interaction in pathophysiological scenarios such as stroke. Clearly, this intriguing relationship will be the focus of future study.
To date, there is no evidence that Panx2 and Panx3 interact. Further, when ectopically co-expressed they are distributed to different subcellular compartments.Citation21 Moreover, such an interaction may not be physiologically relevant, as these Panxs have not yet been identified to endogenously co-exist within the same cell type.Citation5,Citation45-Citation47 Thus, while Panx intermixing represents a putative regulatory mechanism for anterograde Panx trafficking, further information regarding endogenous co-expression profiles of Panx family members is required to fully appreciate its significance.
Retrograde Trafficking of Panxs
Although several studies have addressed the regulation of anterograde trafficking of Panx channels, limited information is available regarding Panx internalization and retrograde transport. Following internalization into early endosomes, plasma membrane proteins may traffic to earlier secretory compartments (ER, Golgi) through retrograde transport pathways, recycle back to the cell surface, or be degraded in endolysosomal compartments. Current work, again focused on Panx1, demonstrates an endolysosomal targeting and degradation of a plasma membrane Panx1 population ().Citation35 However the method of channel internalization is unknown.
Panx1 partially co-distributes with several proteins involved in each of the three canonical modes of internalization (clathrin-, caveolin-, and dynamin-mediated endocytosis).Citation35 However, Panx1 fails to co-precipitate with any of these proteins. Moreover, pathway-specific inhibition of endocytosis had no effect on Panx1 internalization.Citation35 While these data are unable to demonstrate the mechanism responsible for internalization of Panx1, it is important to consider that these studies were performed in a cell type devoid of endogenous Panx1 expression (BICR-M1Rk cells), and therefore might be missing necessary accessory proteins for proper Panx internalization. Consequently, such pathways should be further evaluated in an endogenous model to re-assess internalization mechanisms.
To further expand on potential retrograde trafficking mechanisms of Panx family members, we have used a combination of published signal sequencesCitation48-Citation50 and bioinformatics tools (Eukaryotic Linear Motifs; http://elm.eu.org/) to identify canonical targeting sequences in their intracellular domains (). Interestingly, we have uncovered signal sequences for clathrin-mediated endocytosis and endolysosomal localization in each Panx. Moreover, using live cell confocal microscopy of Panx1-EGFP expressing Neuro-2A neuroblastoma cells (which endogenously express Panx1)Citation17 stimulated with increased extracellular potassium, we observe EGFP-positive vesicles undergoing retrograde transit from the plasma membrane to subcellular regions (). While a clathrin-mediated mechanism of internalization implied from these data has not yet been experimentally determined, it is evident that Panxs indeed undergo retrograde trafficking, the function of which is not fully understood.
Figure 2. Panxs contain putative endocytotic recognition sequences and endolysosomal targeting sequences. Each Panx subunit contains four transmembrane domains, two extracellular loops, and three intracellular domains, including an N-terminus (NH2), intracellular loop, and a highly divergent C-terminus (COOH). The Panx2 C-terminus is the most highly divergent domain in terms of sequence homology and length, at over twice the length (361 amino acids – residues 316–677) of the Panx1 (128 amino acids - residues 298–426) and Panx3 C-termini (103aa – residues 289–392). Amino acid position of the predicted fourth transmembrane domain and the terminal amino acid of each C-terminus are indicated next to their corresponding domains. A combination of known signaling sequences and bioinformatics tools predict that each Panx protein contains both endolysosomal targeting sequences (BLUE; “*TRG_LysEnd_APsAcLL” - http://elm.eu.org/) and endocytotic recognition sequences (GREEN; “TRG_ENDOCYTOTIC_2” - http://elm.eu.org/) that putatively interact with GGA adaptor and AP protein complexes – key players in clathrin-mediated endocytosis.Citation49-Citation51
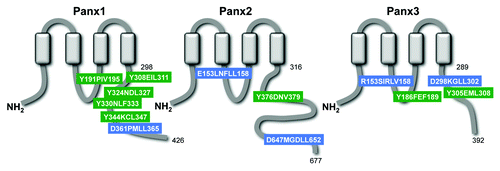
Figure 3. Panx1 activation stimulates channel internalization. (A) Selected time-lapse images from confocal live cell imaging of Panx1-EGFP expressing Neuro-2A neuroblastoma cells, demonstrates retrograde movement of EGFP-positive vesicles (arrows track same structure - images captured every minute) following extracellular elevated K+ (10mM) stimulus. Scale bar = 10μm. Hoechst 33342 was used as a nuclear stain. (B) western blotting analysis of cell surface biotinylation followed by NeutrAvidin bead pulldown illustrates Panx1-EGFP expression at the plasma membrane in Neuro-2A neuroblastoma cells. Lower levels of Panx1-EGFP were observed in the biotinylated fraction following calcimycin A23187 ionophore treatment (10µM, 4 h; right panel) compared with control (left panel). The membranes were stripped and re-probed for LaminB (nuclear) to control for any non-specific biotinylation of intracellular proteins (lower panels). These western blots are representative of three independent biological replicates. (C) Quantification of the relative levels of cell surface Panx1-EGFP under control and A23187 treatments. Calcimycin significantly reduced cell surface Panx1 levels (P = 0.0077 by unpaired Student’s t test). Western blot quantifications were performed using ImageJ. (D) We propose that upon stimulation (increased extracellular K+ or intracellular Ca2+) activated Panx1 channels are internalized and trafficked in retrograde, likely to endolysosomes.
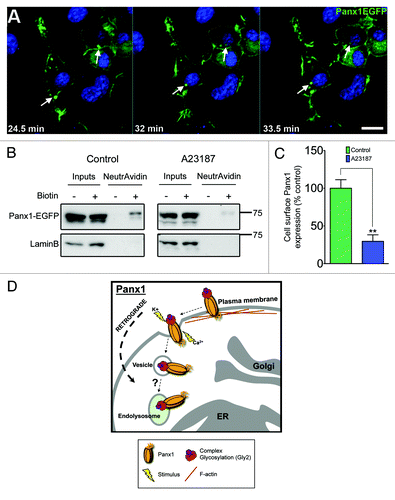
What factors might lead to the internalization of Panxs?
Classic channel and receptor literature demonstrates that prolonged levels of channel/receptor activation often lead to deactivation and/or internalization of these proteins in order to downregulate the associated transmembrane signals.Citation51,Citation52 Therefore Panx activation, under both physiological and pathophysiological conditions, could likely result in channel internalization (). Indeed, in our live cell experiments, we observe retrograde transport of Panx-EGFP positive vesicles with increasing levels of extracellular potassium, a known activating stimulus for Panx1.Citation7 It should be noted that this treatment induces cell depolarization (another known activator or Panx1),Citation27,Citation42 and the surface expression of other proteins were not analyzed to rule out generalized protein internalization. Furthermore, upon treatment with calcimycin A23187, a calcium ionophore which raises intracellular Ca2+ levels,Citation11 we notice a decrease in the cell surface proportion of exogenous Panx1EGFP (). Overall, since alterations to Panx localization are expected to variably impact their physiological and pathophysiological roles, understanding the signals mediating Panx internalization and retrograde transport will provide important information for channel function.
Concluding Remarks
The Panx family of single membrane channels exhibits highly variable tissue, cell type and subcellular distributions, controlled through a complex interplay of intrinsic and extrinsic molecular signaling mechanisms that we are just beginning to understand. Dynamic interaction between Panx post-translational modifications, intrinsic signaling sequences, protein-protein interactions, and subunit intermixing appear to tightly regulate the anterograde and retrograde trafficking patterns, and subsequent subcellular localizations of the different Panx family members. As we are just beginning to uncover the variety of physiological and pathophysiological roles of Panxs, the emerging understanding of Panx subcellular distributions across different cell and tissue types will provide important insight into the cellular functions of Panxs.
Disclosure of Potential Conflicts of Interest
No potential conflicts of interest were disclosed.
Acknowledgments
Research in the Swayne lab is supported by an NSERC Discovery grant, a Heart and Stroke Foundation Partnership for Stroke Recovery grant, and a University of Victoria Division of Medical Sciences start up grant to Swayne LA. Boyce AKJ is supported by an NSERC Canadian Graduate Scholarship—Masters and a University of Victoria Graduate Award. Prager RT is supported by a Jamie Cassels Undergraduate Research Award. Wicki-Stordeur LE is supported by a Vanier Canada Graduate Scholarship (NSERC), a Howard E. Petch research scholarship, and an Edythe Hembroff-Schleicher graduate scholarship. We thank the Victoria Foundation Willard and Elva Dawson Fund for previous stipend support for Boyce AKJ and Wicki-Stordeur LE. We are grateful to the Canadian Foundation for Innovation and the British Columbia Knowledge Development Fund for granting funds for our confocal microscope.
References
- Sosinsky GE, Boassa D, Dermietzel R, Duffy HS, Laird DW, MacVicar B, Naus CC, Penuela S, Scemes E, Spray DC, et al. Pannexin channels are not gap junction hemichannels. Channels (Austin) 2011; 5:193 - 7; http://dx.doi.org/10.4161/chan.5.3.15765; PMID: 21532340
- Ambrosi C, Gassmann O, Pranskevich JN, Boassa D, Smock A, Wang J, Dahl G, Steinem C, Sosinsky GE. Pannexin1 and Pannexin2 channels show quaternary similarities to connexons and different oligomerization numbers from each other. J Biol Chem 2010; 285:24420 - 31; http://dx.doi.org/10.1074/jbc.M110.115444; PMID: 20516070
- Bao L, Locovei S, Dahl G. Pannexin membrane channels are mechanosensitive conduits for ATP. FEBS Lett 2004; 572:65 - 8; http://dx.doi.org/10.1016/j.febslet.2004.07.009; PMID: 15304325
- Ma W, Hui H, Pelegrin P, Surprenant A. Pharmacological characterization of pannexin-1 currents expressed in mammalian cells. J Pharmacol Exp Ther 2009; 328:409 - 18; http://dx.doi.org/10.1124/jpet.108.146365; PMID: 19023039
- Bruzzone R, Hormuzdi SG, Barbe MT, Herb A, Monyer H. Pannexins, a family of gap junction proteins expressed in brain. Proc Natl Acad Sci U S A 2003; 100:13644 - 9; http://dx.doi.org/10.1073/pnas.2233464100; PMID: 14597722
- Santiago MF, Veliskova J, Patel NK, Lutz SE, Caille D, Charollais A, Meda P, Scemes E. Targeting pannexin1 improves seizure outcome. PLoS One 2011; 6:e25178; http://dx.doi.org/10.1371/journal.pone.0025178; PMID: 21949881
- Locovei S, Wang J, Dahl G. Activation of pannexin 1 channels by ATP through P2Y receptors and by cytoplasmic calcium. FEBS Lett 2006; 580:239 - 44; http://dx.doi.org/10.1016/j.febslet.2005.12.004; PMID: 16364313
- Thompson RJ, Zhou N, MacVicar BA. Ischemia opens neuronal gap junction hemichannels. Science 2006; 312:924 - 7; http://dx.doi.org/10.1126/science.1126241; PMID: 16690868
- Bargiotas P, Krenz A, Hormuzdi SG, Ridder DA, Herb A, Barakat W, Penuela S, von Engelhardt J, Monyer H, Schwaninger M. Pannexins in ischemia-induced neurodegeneration. Proc Natl Acad Sci U S A 2011; 108:20772 - 7; http://dx.doi.org/10.1073/pnas.1018262108; PMID: 22147915
- Thompson RJ, Jackson MF, Olah ME, Rungta RL, Hines DJ, Beazely MA, MacDonald JF, MacVicar BA. Activation of pannexin-1 hemichannels augments aberrant bursting in the hippocampus. Science 2008; 322:1555 - 9; http://dx.doi.org/10.1126/science.1165209; PMID: 19056988
- Silverman WR, de Rivero Vaccari JP, Locovei S, Qiu F, Carlsson SK, Scemes E, Keane RW, Dahl G. The pannexin 1 channel activates the inflammasome in neurons and astrocytes. J Biol Chem 2009; 284:18143 - 51; http://dx.doi.org/10.1074/jbc.M109.004804; PMID: 19416975
- MacVicar BA, Thompson RJ. Non-junction functions of pannexin-1 channels. Trends Neurosci 2010; 33:93 - 102; http://dx.doi.org/10.1016/j.tins.2009.11.007; PMID: 20022389
- Baranova A, Ivanov D, Petrash N, Pestova A, Skoblov M, Kelmanson I, Shagin D, Nazarenko S, Geraymovych E, Litvin O, et al. The mammalian pannexin family is homologous to the invertebrate innexin gap junction proteins. Genomics 2004; 83:706 - 16; http://dx.doi.org/10.1016/j.ygeno.2003.09.025; PMID: 15028292
- Iwamoto T, Nakamura T, Doyle A, Ishikawa M, de Vega S, Fukumoto S, Yamada Y. Pannexin 3 regulates intracellular ATP/cAMP levels and promotes chondrocyte differentiation. J Biol Chem 2010; 285:18948 - 58; http://dx.doi.org/10.1074/jbc.M110.127027; PMID: 20404334
- Ishikawa M, Iwamoto T, Nakamura T, Doyle A, Fukumoto S, Yamada Y. Pannexin 3 functions as an ER Ca(2+) channel, hemichannel, and gap junction to promote osteoblast differentiation. J Cell Biol 2011; 193:1257 - 74; http://dx.doi.org/10.1083/jcb.201101050; PMID: 21690309
- Cowan KN, Langlois S, Penuela S, Cowan BJ, Laird DW. Pannexin1 and Pannexin3 exhibit distinct localization patterns in human skin appendages and are regulated during keratinocyte differentiation and carcinogenesis. Cell Commun Adhes 2012; 19:45 - 53; http://dx.doi.org/10.3109/15419061.2012.712575; PMID: 22947051
- Wicki-Stordeur LE, Boyce AK, Swayne LA. Analysis of a pannexin 2-pannexin 1 chimeric protein supports divergent roles for pannexin C-termini in cellular localization. Cell Commun Adhes 2013; 20:73 - 9; http://dx.doi.org/10.3109/15419061.2013.791681; PMID: 23659289
- Swayne LA, Sorbara CD, Bennett SA. Pannexin 2 is expressed by postnatal hippocampal neural progenitors and modulates neuronal commitment. J Biol Chem 2010; 285:24977 - 86; http://dx.doi.org/10.1074/jbc.M110.130054; PMID: 20529862
- Penuela S, Celetti SJ, Bhalla R, Shao Q, Laird DW. Diverse subcellular distribution profiles of pannexin 1 and pannexin 3. Cell Commun Adhes 2008; 15:133 - 42; http://dx.doi.org/10.1080/15419060802014115; PMID: 18649185
- Vanden Abeele F, Bidaux G, Gordienko D, Beck B, Panchin YV, Baranova AV, Ivanov DV, Skryma R, Prevarskaya N. Functional implications of calcium permeability of the channel formed by pannexin 1. J Cell Biol 2006; 174:535 - 46; http://dx.doi.org/10.1083/jcb.200601115; PMID: 16908669
- Penuela S, Bhalla R, Nag K, Laird DW. Glycosylation regulates pannexin intermixing and cellular localization. Mol Biol Cell 2009; 20:4313 - 23; http://dx.doi.org/10.1091/mbc.E09-01-0067; PMID: 19692571
- Penuela S, Bhalla R, Gong XQ, Cowan KN, Celetti SJ, Cowan BJ, Bai D, Shao Q, Laird DW. Pannexin 1 and pannexin 3 are glycoproteins that exhibit many distinct characteristics from the connexin family of gap junction proteins. J Cell Sci 2007; 120:3772 - 83; http://dx.doi.org/10.1242/jcs.009514; PMID: 17925379
- Boassa D, Qiu F, Dahl G, Sosinsky G. Trafficking dynamics of glycosylated pannexin 1 proteins. Cell Commun Adhes 2008; 15:119 - 32; http://dx.doi.org/10.1080/15419060802013885; PMID: 18649184
- Bunse S, Locovei S, Schmidt M, Qiu F, Zoidl G, Dahl G, Dermietzel R. The potassium channel subunit Kvbeta3 interacts with pannexin 1 and attenuates its sensitivity to changes in redox potentials. FEBS J 2009; 276:6258 - 70; http://dx.doi.org/10.1111/j.1742-4658.2009.07334.x; PMID: 19780818
- Bhalla-Gehi R, Penuela S, Churko JM, Shao Q, Laird DW. Pannexin1 and pannexin3 delivery, cell surface dynamics, and cytoskeletal interactions. J Biol Chem 2010; 285:9147 - 60; http://dx.doi.org/10.1074/jbc.M109.082008; PMID: 20086016
- Lai CP, Bechberger JF, Naus CC. Pannexin2 as a novel growth regulator in C6 glioma cells. Oncogene 2009; 28:4402 - 8; http://dx.doi.org/10.1038/onc.2009.283; PMID: 19749789
- Yang J, Bhaumik M, Liu Y, Stanley P. Regulation of N-linked glycosylation. Neuronal cell-specific expression of a 5′ extended transcript from the gene encoding N-acetylglucosaminyltransferase I. Glycobiology 1994; 4:703 - 12; http://dx.doi.org/10.1093/glycob/4.5.703; PMID: 7881185
- Dennis JW, Granovsky M, Warren CE. Protein glycosylation in development and disease. Bioessays 1999; 21:412 - 21; http://dx.doi.org/10.1002/(SICI)1521-1878(199905)21:5<412::AID-BIES8>3.0.CO;2-5; PMID: 10376012
- Haitin Y, Attali B. The C-terminus of Kv7 channels: a multifunctional module. J Physiol 2008; 586:1803 - 10; http://dx.doi.org/10.1113/jphysiol.2007.149187; PMID: 18218681
- Snyder PM. Minireview: regulation of epithelial Na+ channel trafficking. Endocrinology 2005; 146:5079 - 85; http://dx.doi.org/10.1210/en.2005-0894; PMID: 16150899
- Leithe E, Rivedal E. Ubiquitination of gap junction proteins. J Membr Biol 2007; 217:43 - 51; http://dx.doi.org/10.1007/s00232-007-9050-z; PMID: 17657522
- Feng W, Zhang M. Organization and dynamics of PDZ-domain-related supramodules in the postsynaptic density. Nat Rev Neurosci 2009; 10:87 - 99; http://dx.doi.org/10.1038/nrn2540; PMID: 19153575
- Coussen F. Molecular determinants of kainate receptor trafficking. Neuroscience 2009; 158:25 - 35; http://dx.doi.org/10.1016/j.neuroscience.2007.12.052; PMID: 18358623
- Yen MR, Saier MH Jr.. Gap junctional proteins of animals: the innexin/pannexin superfamily. Prog Biophys Mol Biol 2007; 94:5 - 14; http://dx.doi.org/10.1016/j.pbiomolbio.2007.03.006; PMID: 17507077
- Gehi R, Shao Q, Laird DW. Pathways regulating the trafficking and turnover of pannexin1 protein and the role of the C-terminal domain. J Biol Chem 2011; 286:27639 - 53; http://dx.doi.org/10.1074/jbc.M111.260711; PMID: 21659516
- Sandilos JK, Chiu YH, Chekeni FB, Armstrong AJ, Walk SF, Ravichandran KS, Bayliss DA. Pannexin 1, an ATP release channel, is activated by caspase cleavage of its pore-associated C-terminal autoinhibitory region. J Biol Chem 2012; 287:11303 - 11; http://dx.doi.org/10.1074/jbc.M111.323378; PMID: 22311983
- Chekeni FB, Elliott MR, Sandilos JK, Walk SF, Kinchen JM, Lazarowski ER, Armstrong AJ, Penuela S, Laird DW, Salvesen GS, et al. Pannexin 1 channels mediate ‘find-me’ signal release and membrane permeability during apoptosis. Nature 2010; 467:863 - 7; http://dx.doi.org/10.1038/nature09413; PMID: 20944749
- Weilinger NL, Tang PL, Thompson RJ. Anoxia-induced NMDA receptor activation opens pannexin channels via Src family kinases. J Neurosci 2012; 32:12579 - 88; http://dx.doi.org/10.1523/JNEUROSCI.1267-12.2012; PMID: 22956847
- Wicki-Stordeur LE, Swayne LA. Panx1 regulates neural stem and progenitor cell behaviours associated with cytoskeletal dynamics and interacts with multiple cytoskeletal elements. Cell Commun Signal 2013; 11:62; http://dx.doi.org/10.1186/1478-811X-11-62; PMID: 23964896
- Noda Y, Sasaki S. The role of actin remodeling in the trafficking of intracellular vesicles, transporters, and channels: focusing on aquaporin-2. Pflugers Arch 2008; 456:737 - 45; http://dx.doi.org/10.1007/s00424-007-0404-2; PMID: 18066585
- Fletcher DA, Mullins RD. Cell mechanics and the cytoskeleton. Nature 2010; 463:485 - 92; http://dx.doi.org/10.1038/nature08908; PMID: 20110992
- Bruzzone R, Barbe MT, Jakob NJ, Monyer H. Pharmacological properties of homomeric and heteromeric pannexin hemichannels expressed in Xenopus oocytes. J Neurochem 2005; 92:1033 - 43; http://dx.doi.org/10.1111/j.1471-4159.2004.02947.x; PMID: 15715654
- Silverman W, Locovei S, Dahl G. Probenecid, a gout remedy, inhibits pannexin 1 channels. Am J Physiol Cell Physiol 2008; 295:C761 - 7; http://dx.doi.org/10.1152/ajpcell.00227.2008; PMID: 18596212
- Bargiotas P, Krenz A, Monyer H, Schwaninger M. Functional outcome of pannexin-deficient mice after cerebral ischemia. Channels (Austin) 2012; 6:453 - 6; http://dx.doi.org/10.4161/chan.22315; PMID: 23111424
- Dvoriantchikova G, Ivanov D, Panchin Y, Shestopalov VI. Expression of pannexin family of proteins in the retina. FEBS Lett 2006; 580:2178 - 82; http://dx.doi.org/10.1016/j.febslet.2006.03.026; PMID: 16616526
- Barbe MT, Monyer H, Bruzzone R. Cell-cell communication beyond connexins: the pannexin channels. Physiology (Bethesda) 2006; 21:103 - 14; http://dx.doi.org/10.1152/physiol.00048.2005; PMID: 16565476
- Litvin O, Tiunova A, Connell-Alberts Y, Panchin Y, Baranova A. What is hidden in the pannexin treasure trove: the sneak peek and the guesswork. J Cell Mol Med 2006; 10:613 - 34; http://dx.doi.org/10.1111/j.1582-4934.2006.tb00424.x; PMID: 16989724
- Kelly BT, Owen DJ. Endocytic sorting of transmembrane protein cargo. Curr Opin Cell Biol 2011; 23:404 - 12; http://dx.doi.org/10.1016/j.ceb.2011.03.004; PMID: 21450449
- Bonifacino JS, Traub LM. Signals for sorting of transmembrane proteins to endosomes and lysosomes. Annu Rev Biochem 2003; 72:395 - 447; http://dx.doi.org/10.1146/annurev.biochem.72.121801.161800; PMID: 12651740
- Behnke J, Eskelinen EL, Saftig P, Schröder B. Two dileucine motifs mediate late endosomal/lysosomal targeting of transmembrane protein 192 (TMEM192) and a C-terminal cysteine residue is responsible for disulfide bond formation in TMEM192 homodimers. Biochem J 2011; 434:219 - 31; http://dx.doi.org/10.1042/BJ20101396; PMID: 21143193
- Maier PJ, Marin I, Grampp T, Sommer A, Benke D. Sustained glutamate receptor activation down-regulates GABAB receptors by shifting the balance from recycling to lysosomal degradation. J Biol Chem 2010; 285:35606 - 14; http://dx.doi.org/10.1074/jbc.M110.142406; PMID: 20826795
- Scott DB, Michailidis I, Mu Y, Logothetis D, Ehlers MD. Endocytosis and degradative sorting of NMDA receptors by conserved membrane-proximal signals. J Neurosci 2004; 24:7096 - 109; http://dx.doi.org/10.1523/JNEUROSCI.0780-04.2004; PMID: 15306643