Abstract
DNA vaccines have been shown to elicit tumor-protective cytotoxic T lymphocyte (CTL) immunity in preclinical models, but have shown limited efficacy in cancer patients. Plasmids used for DNA vaccines can stimulate several innate immune receptors, triggering the activation of master transcription factors, including interferon regulatory factor 3 (IRF3) and nuclear factor κ B (NF-κB). These transcription factors drive the production of type I interferons (IFNs) and pro-inflammatory cytokines, which promote the induction of CTL responses. Understanding the innate immune signaling pathways triggered by DNA vaccines that control the generation of CTL responses will increase our ability to design more effective vaccines. To gain insight into the contribution of these pathways, we vaccinated mice lacking different signaling components with plasmids encoding tyrosinase-related protein 2 (TRP2) or ovalbumin (OVA) using intradermal electroporation. Antigen-specific CTL responses were detected by intracellular IFN-γ staining and in vivo cytotoxicity. Mice lacking IRF3, IFN-α receptor, IL-1β/IL-18, TLR9 or MyD88 showed similar CTL responses to wild-type mice, arguing that none of these molecules were required for the immunogenicity of DNA vaccines. To elucidate the role of NF-κB activation we co-vaccinated mice with pIκBα-SR, a plasmid encoding a mutant IκBα that blocks NF-κB activity. Mice vaccinated with pIκBα-SR and the TRP2-encoding plasmid (pTRP2) drastically reduced the frequencies of TRP2-specific CTLs and were unable to suppress lung melanoma metastasis in vivo, as compared with mice vaccinated only with pTRP2. Taken together these results indicate that the activation of NF-κB is essential for the immunogenicity of intradermal DNA vaccines.
Introduction
Vaccinations have been an essential part of basic medical care for over a century, providing protection to various diseases and pathogens. Traditionally inactive or attenuated viruses have been used to establish humoral and cellular immunity. Since the advent of molecular biology and the ease of DNA manipulation, it has been proposed to use DNA as a vehicle of immunization.Citation1 Different techniques have been developed to establish immunity against DNA-encoded antigens (Ag). Intramuscular and intradermal (i.d.) injections of naked DNA followed by electroporation (EP) greatly increases the ability of DNA vaccines to elicit cellular immune responses including cytotoxic CD8+ T lymphocytes (CTL).Citation2,Citation3 Cancer vaccines able to elicit strong antigen-specific CTL responses that are effective at eliminating tumors are highly desired for achieving favorable clinical outcomes.Citation1,Citation4,Citation5
Mature activated antigen presenting cells (APC), such as dendritic cells (DC) are essential for establishing effective CTL responses. DNA vaccines can target sentinel APCs, such as Langerhans cells and dermal DCs, that are proficient in processing the DNA-encoded antigen and migrating to lymphoid organs to elicit Ag-specific T and B cell responses.Citation2,Citation3,Citation6 Skin-resident APCs are well suited to recognize pathogen-associated molecular patterns (PAMPs) such as double stranded plasmid DNA used for DNA vaccines. Pattern recognition receptors (PRRs), such as Toll like receptors (TLRs), present mostly in APCs detect PAMPs and trigger signaling pathways that activate master transcription factors, including interferon regulatory factors (IRF) and nuclear factor κ B (NF-κB). The resulting production of type I interferons (IFNs), proinflammatory cytokines and chemokines promotes DC maturation that in turn generate CTL responses linking innate to adaptive immune responses. TLR9 recognizes DNA containing unmethylated CpG motifs from bacterial and viral DNA present within the endosomal compartment.Citation7 Cytosolic DNA sensors include DExD/H box helicases (DHX9/36),Citation8 DNA-dependent activator of IFN regulatory factors (DAI),Citation9 absent in melanoma 2 (AIM2),Citation10 meiotic recombination 11 homolog A (MRE11),Citation11 gamma-interferon-inducible protein 16 (IFI16),Citation12 leucine rich repeat flightless-interacting protein (LRRFIP1),Citation13 probable ATP-dependent RNA helicase DDX41Citation14 and RNA polymerase III,Citation15 which transcribes dsDNA into double stranded RNA leading to RIG-I activation. However, which of these DNA-sensing signaling pathways promotes the maturation of APCs after DNA electroporation leading to the generation of CTL responses is not fully understood, especially for intradermal vaccination. Understanding the innate immune mechanisms involved in this process will increase our capacity to design more potent DNA vaccines.
In the following study, we explored the mechanisms involved in the induction of CTL responses elicited by intradermal DNA electroporation resulting in tumor protection. INF-α and transcription factor IRF3, previously identified as a key signaling pathway for establishing response to DNA vaccines, were not required for the induction of CTL responses by intradermal DNA vaccination. Furthermore, the generation of CTL responses was found to be independent of the classical TLR9 DNA sensing pathway and any other MyD88-dependent proinflammatory pathway. Lastly, we demonstrate that NF-κB acts as a master transcription factor that is essential for inducing antigen-specific CTLs and in providing protection against in vivo tumor challenge.
Results
Type 1 interferons are not required for intradermal DNA vaccine-induced CTL responses
DNA-sensing PRRs, including TLR9 and cytosolic DNA sensors, can trigger the production of type I IFNs, which are an important bridge between innate and adaptive immunity by promoting APC maturation and induction of T cell responses.Citation17,Citation18 Moreover, type I IFNs have been found to be essential for the immunogenicity of DNA vaccines delivered intramuscularly in a TLR9-independent fashion.Citation19,Citation20 To determine whether type I IFNs are also essential in establishing CTL immunity after intradermal DNA electroporation, wild type (WT) and IFNαR−/− mice on the 129sv background were immunized with the plasmid encoding the model antigen ovalbumin (OVA). In contrast to previous studies, CTL responses measured after the second immunization in peripheral blood from IFNαR−/− mice were not reduced compared with WT mice (). To test whether the effector function of elicited CTLs was impaired in IFNαR deficient mice, an in vivo cytotoxicity assay was performed. Vaccinated mice were adoptively transferred with CFSEhigh and CFSElow labeled splenocytes pulsed with OVA and control peptides, respectively. Flow cytometry analysis of lymph nodes from recipient mice showed that killing of CFSEhigh labeled OVA peptide pulsed lymphocytes was not significantly different between WT and IFNαR−/− mice (p = 0.19), confirming the establishment of functional OVA-specific CTL immunity ().
Figure 1. Type 1 interferons are not required for generating CTL responses. Wild-type (WT) and IFNαR−/− mice on 129sv background were vaccinated twice with the OVA-encoding plasmid pOVA. The percentages of IFN-γ producing CD8+ T lymphocytes were detected after in vitro stimulation with OVA and control peptides (A). In vivo cytotoxicity was measured by quantifying OVA pulsed CFSEHigh labeled target cells and compared with control pulsed CFSELow labeled cells in WT and IFNαR−/− mice vaccinated with pOVA. The mean percentage ± SEM of specific killing for each case is indicated (B). Tyrosinase-related protein 2 (TRP2) encoding DNA was used to vaccinate WT and IFNαR−/− 129sv mice. IFN-γ producing CD8 T lymphocytes were measured after in vitro stimulation with TRP2 and control peptides (C). WT and IRF3−/− mice on C57BL/6 background were vaccinated with TRP2-encoding plasmid and the percentages of IFN-γ-producing CD8 T cells were measured by flow cytometry after in vitro stimulation with TRP2 and control peptides (D). Bars indicate the mean ± SEM.
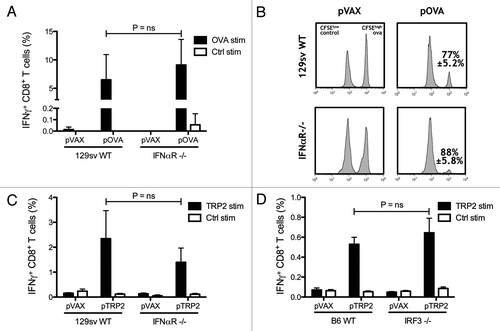
Unlike OVA, tumor antigens are poorly immunogenic and induction of CTL responses against such antigens is controlled by diverse mechanisms of tumor-associated self-tolerance. To identify if the strong immunogenicity of OVA was the reason for the vital CTL responses observed regardless of the deficiency in type I IFN signaling, mice were vaccinated with a vaccine encoding the melanoma antigen tyrosinase-related protein 2 (TRP2). No significant differences in TRP2-specific CTL frequencies could be found between IFNαR−/− and WT mice (). To further confirm these results, the role of IRF3, a key transcription factor driving type I IFN expression resulting after ligation of cytosolic DNA sensors, such as DAI and IFI16, was examined. IRF3−/− mice were able to generate CTL responses as strong as WT mice after vaccination with the TRP2-encoding plasmid (). These results demonstrate that type I IFN/IRF3 signaling axis is not required for the ability of intradermal DNA vaccines to elicit antigen-specific CTL responses.
TLR9 and MyD88 do not mediate the generation of CTL responses after intradermal DNA vaccination
Plasmid DNA produced in the gram-negative bacteria E. coli contains unmethylated CpG motifs that could activate TLR9 and downstream signaling pathways through the adaptor protein myeloid differentiation primary response gene 88 (MyD88), which functions as a central adaptor protein for other TLRs (except TLR3) and the DNA sensor DHX9.Citation21 This led us to speculate that TLR9-mediated innate immune activation could possibly contribute to the specific adaptive CTL responses elicited by intradermal DNA vaccination. To determine if this was indeed the case, TLR9−/−, MyD88−/− and WT mice on C57BL/6 background were vaccinated, as described above, with the OVA-encoding plasmid. Vaccination generated levels of OVA-specific IFN-γ producing CTLs that were similar between the three groups (). To confirm the functionality of the CTLs generated, in vivo cytotoxicity was tested, demonstrating that cytotoxic potential of OVA-specific CTLs was intact in TLR9 and MyD88 KO mice as compared with wild-type mice. In both cases, there was no significant difference in the OVA-specific killing between KO mice and wild-type mice (p = 0.75 and p = 0.98 respectively) (). Similar results were obtained when the less immunogenic TRP2 antigen was tested in these TLR9, MyD88 KO models. TRP2-specific CTL frequencies were not different between wild-type and KO mice (), indicating that TLR9 and downstream MyD88-dependent signaling are not required for the generation of CTL responses elicited by i.d. EP DNA vaccines. A different proinflammatory pathway that can contribute to the immunogenicity of DNA vaccines is the inflammasome. AIM2-mediated DNA sensing can trigger inflammasome activation, leading to the activation of caspase-1 and the catalytic cleavage of pro-forms of the pro-inflammatory cytokines IL-1β and IL-18 into their active secreted form. Given the observed high expression of the inflammasome protein AIM2 in skin,Citation22 we studied whether IL-1β−/− IL-18−/− mice were able to mount similar CTL responses as WT mice. We found that intradermal electroporation induced similar specific CTL responses between the KO and WT mice measured as IFN-γ producingCTLs () and in vivo cytotoxicity ().
Figure 2. Toll-like receptor 9 (TLR9) and myeloid differentiation primary response gene 88 (MyD88) are not essential for CTL induction. Wild-type (WT), TLR9−/− and MyD88−/− mice on C57BL/6 background were vaccinated twice. Levels of IFN-γ producing CD8 T cells induced by DNA vaccination against OVA and TRP2 were measured two weeks after the second DNA vaccination by flow cytometry. Bars indicate the mean ± SEM (A and C). In vivo cytotoxicity was measured by quantifying OVA pulsed CFSEHigh labeled target cells and compared with control pulsed CFSELow labeled cells in WT, TLR9−/− and MyD88−/− mice vaccinated with the OVA encoding plasmid pOVA. The mean percentage ± SEM of specific killing for each case is indicated (B). WT and IL-1β−/− IL-18−/− mice on C57BL/6 background were vaccinated with OVA or TRP2 encoded plasmid as described previously. The percentages of IFN-γ-producing CD8 T cells were measured by flow cytometry after in vitro stimulation with TRP2 and control peptides (D). Functionality of OVA-specific CTLs was measured by in vivo cytotoxicity. Lymph nodes were harvested one day after mice were injected i.v. with OVA peptide pulsed CFSEHigh labeled splenocytes and control peptide pulsed CFSELow splenocytes. Bars indicate the mean percentage ± SEM of OVA-specific killing (E).
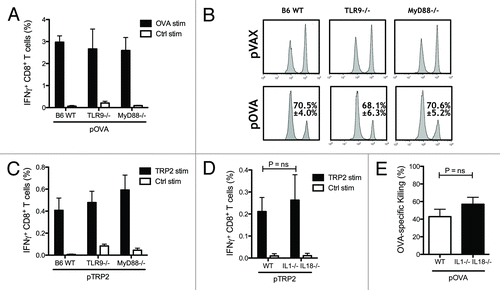
Suppression of NF-κB during intradermal DNA vaccination decreases CTL responses and impedes tumor rejection
Most DNA-sensing PRR (DAI, IFI16, AIM2, DHX9) signaling pathways converge in the activation and nuclear translocation of NF-κB to drive the expression of proinflammatory transcriptional targets. To investigate the role of this pathway, we used a plasmid encoding the IκBα-super repressor (IκBα-SR), which functions similarly to endogenous IκBα in its ability to bind and block NF-κB, but carries mutations that prevent its phosphorylation and subsequent degradation and, as consequence, stably impedes the translocation of NF-κB to the nucleus.Citation23 Thus, this plasmid allows blocking of NF-κB activation specifically at the vaccination site in the context of DNA vaccines.Citation24 To test the activation of NF-κB during DNA vaccination, a NF-κB luciferase reporter plasmid was i.d EP into the hindquarters of mice as done with DNA vaccines. In vivo luciferase activity was detected six hours after intradermal electroporation, which is indicative of early NF-κB activation. As expected, when the functionality of IκBα-SR was evaluated, co-electroporation with the IκBα-SR encoding plasmid showed decreased in vivo bioluminescence (). To test the ability of NF-κB activation during intradermal DNA vaccination to mediate specific CTL antitumor responses, mice were vaccinated with the TRP2–encoding plasmid and pIκBα-SR. This led to a stunning decrease in IFN-γ producing CD8+ T lymphocytes as compared with mice vaccinated with pTRP2 alone ().
Figure 3. NF-κB activation during intradermal DNA vaccination mediates the generation of CTLs against the tumor-associated antigen TRP2. C57BL/6 mice were electroporated with NFκB luciferase reporter plasmid and IκBα-SR encoding plasmid. One day after DNA electroporation, in vivo luminescence was measured after intraperitoneal (i.p.) injection of luciferin. Representative images are shown (A). C57BL/6 mice were vaccinated twice against TRP2. One group was co-electroporated with IκBα-SR encoding plasmid and the control group with the empty vector. Percentage of TRP2-specific IFN-γ-producing CD8 T cells were measured two weeks after the second DNA vaccination by flow cytometry after in vitro stimulation with TRP2 and control peptides. Bars indicate the mean ± SEM (B). C57BL/6 mice vaccinated with pVAX, pTRP2 or co-electroporated with pTRP2 and pIκBα-SR were challenged by i.v. injection of 105 B16F10 melanoma cells. Two weeks after injection lungs were excised and melanoma foci were enumerated. The mean percentage ± SEM of melanoma foci is shown for each group (C).
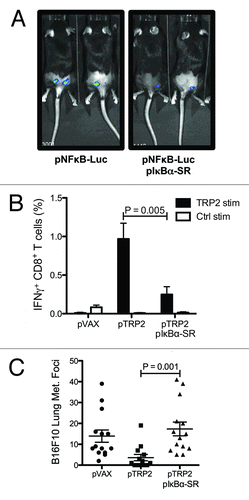
Finally to confirm that the reduced TRP2-specific CTL responses would have an impact on the ability of intradermal DNA vaccination to confer tumor protection, the B16F10 mouse melanoma lung metastasis model was used. Mice were vaccinated with either control plasmid, pTRP2 or co-vaccinated with pTRP2 and IκBα-SR encoding plasmid as described above; 14 d after the second immunization were challenged with 105 B16F10 cells injected i.v. Three weeks after the challenge, lungs were excised and the melanoma metastatic foci were enumerated (). Accordingly, significantly decreased melanoma metastasis was observed in mice vaccinated with TRP2 encoding plasmid, whereas mice co-electroporated with both pTRP2 and pIκBα-SR were unable to suppress melanoma formation. Note that electroporation with the IκBα-SR encoding plasmid did not affect lung metastasis when administrated by itself (data not shown). In conclusion, these results indicate that the activation of NF-κB during intradermal DNA vaccination is essential for the induction of CTL-mediated tumor protective immunity.
Discussion
The promise that DNA vaccines would lead to a revolution in vaccine technology has not yet materialized. Many clinical trials have been falling short of their mark with little explanation as to possible reasons, while in contrast, mouse models have shown therapeutic and prophylactic efficacy. In this work we attempt to shed some light on the mechanisms involved in establishing adaptive T cell responses elicited by DNA vaccination. We show here that naked plasmid DNA vaccines delivered via an intradermal route and assisted with electroporation produce antigen-specific CTL responses through a mechanism that relies on the activation of NF-κB. In contrast, the IRF3-type 1 IFN and TLR9-MyD88 innate immune pathways, generally considered to contribute to the sensing of DNA and the initiation of adaptive immune responses, were not required for establishing antigen-specific CTLs.
Electroporated DNA vaccines lead to high expression levels of the plasmid-encoded antigen as well as the introduction of large amounts of plasmid DNA inside the cells that represent a strong signal for several DNA-sensing innate immune receptors. The sensing of DNA by PRRs has become a complex research field, with a multitude of proteins binding to DNA and activating distinct signaling cascades, as recently reviewed in detail by Desmet et al.Citation21 The net result of these pathways is the production of type I IFNs and proinflammatory cytokines, including IL-1β and IL-18 that promote DC maturation and initiate robust cellular immune responses. In response to DNA, DAI, IFI16, DHX36, DDX41, RNA-PolIII, and LRRFIP1 trigger the production of type I IFNs primarily via TBK1-dependent activation of IRF3, whereas TLR9 and DHX9 can signal through MyD88 to activate IRF7. Type I IFNs lead to the maturation of DCs, inducing an increased expression of MHC molecules and costimulatory molecules, such as CD80 and CD86.Citation18 Importantly, type I IFNs also provide the “third signal” needed for CD8+ T cells to undergo clonal proliferation and differentiation into CTLs.Citation17,Citation25,Citation26 The importance of the type I IFN signaling, in particular the TBK-1/IRF3-dependent pathway, for innate immune recognition of DNA vaccines has been established by studies showing that adaptive immune responses elicited by DNA vaccination are almost completely abrogated in mice lacking the components of this cascade.Citation19,Citation20,Citation27 Ishii et al. found that IFNαR−/− and Tbk1−/− mice were not able to generate LacZ specific CD8+, as measured by MHC tetramers, or stimulate antigen-specific CD4+ and CD8+ T-cell proliferation.Citation20 Similarly, Shirota et al. found that IFNαR−/− and IRF3−/− mice displayed severely impaired antigen-specific CD4+ and CD8+ T cell responses, including the production of Th1, Th2, and Th17 cytokines.Citation19 In contrast to the aforementioned studies, in this study we present evidence demonstrating that, at least with DNA vaccines delivered by intradermal electroporation, IRF3- or MyD88-dependent type I IFN signaling is not required for the induction of fully functional antigen-specific T cell responses ( and ) These opposing results can be explained by the different administration routes used in each particular case. Studies proposing a preponderant role for type I IFN signaling have been performed after intramuscular immunization (with or without electroporation), whereas our studies were conducted using intradermal electroporation. It seems reasonable to expect that delivering DNA intramuscularly would target different sets of both DNA-sensing and antigen-presenting cells, than when injecting intradermally. Similar divergences have been found when immunizing with alphavirus replicon-based DNA vectors, which activate dsRNA-sensing innate immune receptors.Citation28,Citation29 Induction of antigen-specific T cell responses elicited by vaccination with replicon-based DNA vectors delivered intramuscularly has shown to be dependent on type I IFNsCitation27 while in a different study immunizing with the same kind of vector but delivered intradermally, type I IFN signaling was absolutely dispensable, and rather had a suppressive effect.Citation30 The contrasting results obtained by different groups seem to be related to the vaccination route, further encouraging the need of more comprehensive studies dissecting the signaling pathways activated in each particular tissue.Citation30 Indeed, a different situation can be observed within the tumor microenvironment, where activation of type I IFN-producing PRRs has been shown to promote potent anti-tumor effects by acting on antigen-presenting cells and subsequently on tumor-specific T cells.Citation31,Citation32 Treatment of B16F10 melanoma tumors with poly(I:C) and CpG oligos, triggering TLR3 and 9 respectively, protected mice from tumor challenge together with melanoma specific T-cell transfer. Poly(I:C) and CpG were shown to be responsible for upregulation of CD40 and CD86 on pDCs enriched from tumor draining lymph nodes.Citation31 In the context of vaccination, it has been found that intratumoral injection of TLR3/9 ligands was responsible for reprograming the infiltrating immune cell population; significantly increasing the CD8 to Treg cell ratio and anti-tumor effects of the vaccine strategy.Citation32
In addition to type I IFNs, proinflammatory cytokines produced after DNA stimulation play a direct role in promoting DC maturation and T cell induction. Activation of TLR9-MyD88 pathway is a major source of both type I IFNs and proinflammatory cytokines. However, the participation of TLR9 in the immunogenicity of DNA vaccines is controversial with different studies showing TLR9-dependent and -independent mechanisms. In this study, we found that TLR9 and the adaptor protein MyD88 did not play an important role in establishing the pDNA induced adaptive CTL responses, which were similar between the KO and WT mice. In vivo cytotoxicity showed a trend to less effective killing in the KO mice, though this was not statistically significant (). Our findings are in agreement with previous investigations showing that TLR9 and MyD88 KO mice are able to mount antigen-specific CTL responses after i.m. DNA vaccination.Citation33,Citation34 Considering all these studies, it can be concluded that TLR9 contribute but is dispensable for T cell responses elicited by DNA vaccines delivered, and targeting TLR9 represents an opportunity for improvement of adaptive immune responses. Indeed, strategies introducing more CpG motifs into the antigen-encoding plasmid DNA vector have been shown to further boost antibody titers in i.m. DNA vaccines.Citation35-Citation37 A key proinflammatory pathway downstream of DNA recognition is the production of IL-1β and IL-18.Citation36 The pro-forms of the IL-1 family members are cleaved into their functional state by caspase-1 through interaction with AIM2-containing inflammasome. It has also been described by Roos et al. that IL-1β mRNA expression is increased 1000 fold after DNA electroporation in the skin.Citation39 Although AIM2 can be expressed in skin-resident cells, IL-1β−/− IL-18−/− mice were able to generate CTL responses as efficiently as wild-type mice further discarding that the inflammasome pathway is required for DNA vaccine-induced innate immune activation.
The maturation of DCs and the subsequent activation of adaptive immunity are regulated largely by NK-κB signaling pathways. The classical NF-κB signaling cascade triggered in response to dsDNA by DAI, IFI16, AIM2, DHX9, DDX41 DNA sensors,Citation21 relies on formation and activation of the NEMO/IκB kinase complex. This complex phosphorylates IκBα, facilitating the ubiquitination and proteasomal degradation of this protein. Once IκBα is degraded, it is no longer able to sequester NF-κB in the cytosol, the p50-RelA dimer then translocates to the nucleus.Citation40 Our studies show the key role of transcription factor NF-κB in establishing effective anti-tumor CTL responses by intradermal electroporation of plasmid DNA vaccines. By co-electroporating a mutant IκBα construct into the dermis of the skin along with antigen-encoding plasmids, the mechanism for triggering adaptive immunity is abrogated, preventing the tumor protection otherwise offered by DNA vaccines (). Electroporation of the IκBα-SR construct did not have an effect on tumor metastasis when administrated independently of the TRP2 encoding plasmid (data not shown). Among other targets, activation of NF-κB leads to the production of inflammatory cytokines, such as: IL-6, IL-1β, IL-12, and TNF-α. Among these molecules and similarly to type I IFNs, IL-12 is also able to provide the “third signal” to CD8+ T cells for promoting clonal proliferation and differentiation into CTLs. Therefore, NF-κB can provide all the necessary signals for generating effective T cell immunity. Despite the ability of NF-κB to support malignant cell survival, proliferation, and metastatic potential when expressed in tumor cells;Citation41 activation of NF-κB during antigen presentation has been extensively demonstrated to promote anti-tumor immune responses using TLR agonistsCitation27,Citation28 and DNA vaccine adjuvants.Citation24 In conclusion, in our study we demonstrate the essential nature of NF-κB in establishing CTL immunity after intradermal DNA vaccination. However, identifying which of the multiple DNA-sensing receptors that is involved in sensing intradermally electroporated DNA will be the focus of future studies. A more complete understanding of the DNA-sensing signaling networks initiated after DNA vaccination will allow us to design more effective vaccines.
Materials and Methods
Animals and cells
C57BL/6, TLR9−/−, MyD88−/−, IRF3 −/−, 129sv, and IFN-αR−/− mice were kept in accordance with the local Animal Ethics Committee guidelines. Mice were bred and maintained at the Microbiology and Tumor Biology center at the Karolinska Institute. B16F10 were cultured in complete RPMI medium, supplemented with 100 IU/ml penicillin and 100 µg/ml streptomycin, one percent non-essential amino acids, one percent sodium pyruvate and 10 percent heat-inactivated fetal bovine serum (Gibco 21875, 15140, 11140, 11360, and 10270 respectively) in a humidified incubator at 37 °C with 5% CO2.
DNA vaccination
Vaccination was done in mice as previously described.Citation16 Briefly, mice were anesthetized with isoflurane at gas concentration of 3.5%. Vaccination was delivered by injecting 20 µg of each plasmid DNA dissolved in 40 µl of phosphate-buffered saline intradermally. The intradermal injection was done in two different sites (20 µl each) on the hindquarters of the mouse. Electroporation of the injection site was done using the Derma Vax DNA Vaccine Skin Delivery System (Cellectis) that delivered electric pulses (two 1125 V/cm, 0.05 ms pulses followed by eight 275 V/cm, 10 ms pulses) via a parallel needle array electrode placed on the injection site. Immunization of the mice was done twice with a two-week interval. The plasmids used for immunization were as follows: pTRP2 encoding human TRP2 (kindly provided by Dr. T. Wölfel, Johannes Gutenberg University), pOVA encoding membrane-bound ovalbumin (kindly provided by Dr. A. Lew, Walter and Eliza Hall Institute of Medical Research), pIκBα-SR encoding the IκBα supper-repressor (kindly provided by Dr R. Toftgård, Karolinska Institutet), and pNF-κB-Fluc encoding firefly luciferase under the control of a NF-κB promoter. Plasmids were purified using EndoFree Plasmid Giga Kit (Qiagen 12391).
Intracellular cytokine staining
Peripheral blood was taken 13 d after the second DNA vaccination from the tail vein. Lymphocytes were cultured with 1 µg/ml of antigen-derived or control MHC class I-restricted peptide during eight hours. GolgiPlug was added during the last six hours. After surface staining, cells were stained intracellularly using the Cytofix/Cytoperm and fixation/permeabilization kit (BD Biosciences 554722 and 554723) following manufacturer’s instructions. Monoclonal anti-mouse CD-8α FITC conjugated, IFN-γ PE conjugated TNF-α APC antibodies were used (Biolegend clones 5H10–1, XMG1.2 and MP6-XT22 respectively). Nonspecific binding was blocked by mouse Fc receptor blocking (Biolegend clone 93). Acquisition and analysis of cells was performed with FACSCalibur and FlowJo version 9.2, respectively.
In vivo cytotoxicity
Splenocytes harvested from naïve mice were pulsed with OVA or control peptide after being labeled with 2 and 0.2 µmol/l of CFSE respectively. Then, 107 pulsed splenocytes from each population were mixed and injected intravenously into vaccinated mice. The next day, inguinal lymph nodes from recipient mice were analyzed by flow cytometry. DNA vaccine mediated killing was determined as follows: 100 – (% of CFSEhigh OVA peptide-pulsed cells/% of CFSElow control peptide-pulsed cells).
In vivo luminescence
Six hours after C57BL/6 mice were vaccinated with plasmid encoding firefly luciferase, under control of an NF-κB promoter, luminescence was measured. This was done by anesthetizing the mice with isoflurane prior to intraperitoneally injecting 100 µl of 30 mg/ml D-luciferin (Promega E1603) dissolved in phosphate buffered saline solution. Mice were scanned with the Xenogen IVIS 100 imaging system where bioluminescence was measured.
Lung metastasis model
DNA vaccinated C57BL/6 mice were challenged by intravenous injection of 105 B16F10 cells 14 d after the second DNA vaccination. Three weeks after intravenous challenge, mice were sacrificed and lungs were washed in phosphate buffered saline solution before bleaching in Feketes solution. Analysis of metastasis was performed by enumeration of the visible pigmented melanoma foci in the lung surface.
Acknowledgments
Research described here have been supported by grants to AL and RK from FONDECYT 11110525, CONICYT 791100038, CONICYT Program PFB-16, CORFO-Innova 12IDL2-13348, The Swedish Medical Research Council (K2011-66X-15387-07-3 VR), The Swedish Cancer Society (12 0598 Cancerfonden), The Cancer Society of Stockholm (121103 Cancerföreningen, Radiumhemmets Forskningsfonder), ALF-Project grant from Stockholm City Council (20110070 ALF Medicin 2012).
Disclosure of Potential Conflicts of Interest
No potential conflicts of interest were disclosed.
References
- Tang DC, DeVit M, Johnston SA. Genetic immunization is a simple method for eliciting an immune response. Nature 1992; 356:152 - 4; http://dx.doi.org/10.1038/356152a0; PMID: 1545867
- Luxembourg A, Evans CF, Hannaman D. Electroporation-based DNA immunisation: translation to the clinic. Expert Opin Biol Ther 2007; 7:1647 - 64; http://dx.doi.org/10.1517/14712598.7.11.1647; PMID: 17961089
- van Drunen Littel-van den Hurk S, Hannaman D. Electroporation for DNA immunization: clinical application. Expert Rev Vaccines 2010; 9:503 - 17; http://dx.doi.org/10.1586/erv.10.42; PMID: 20450325
- Mlecnik B, Bindea G, Pagès F, Galon J. Tumor immunosurveillance in human cancers. Cancer Metastasis Rev 2011; 30:5 - 12; http://dx.doi.org/10.1007/s10555-011-9270-7; PMID: 21249426
- Muul LM, Spiess PJ, Director EP, Rosenberg SA. Identification of specific cytolytic immune responses against autologous tumor in humans bearing malignant melanoma. J Immunol 1987; 138:989 - 95; PMID: 3100623
- Igyártó BZ, Kaplan DH. Antigen presentation by Langerhans cells. Curr Opin Immunol 2013; 25:115 - 9; http://dx.doi.org/10.1016/j.coi.2012.11.007; PMID: 23246038
- Hemmi H, Takeuchi O, Kawai T, Kaisho T, Sato S, Sanjo H, et al. A Toll-like receptor recognizes bacterial DNA. Nature 2000; 408:740 - 5; http://dx.doi.org/10.1038/35047123; PMID: 11130078
- Kim T, Pazhoor S, Bao M, Zhang Z, Hanabuchi S, Facchinetti V, et al. Aspartate-glutamate-alanine-histidine box motif (DEAH)/RNA helicase A helicases sense microbial DNA in human plasmacytoid dendritic cells. Proc Natl Acad Sci U S A 2010; 107:15181 - 6; http://dx.doi.org/10.1073/pnas.1006539107; PMID: 20696886
- Takaoka A, Wang Z, Choi MK, Yanai H, Negishi H, Ban T, et al. DAI (DLM-1/ZBP1) is a cytosolic DNA sensor and an activator of innate immune response. Nature 2007; 448:501 - 5; http://dx.doi.org/10.1038/nature06013; PMID: 17618271
- Bürckstümmer T, Baumann C, Blüml S, Dixit E, Dürnberger G, Jahn H, et al. An orthogonal proteomic-genomic screen identifies AIM2 as a cytoplasmic DNA sensor for the inflammasome. Nat Immunol 2009; 10:266 - 72; http://dx.doi.org/10.1038/ni.1702; PMID: 19158679
- Kondo T, Kobayashi J, Saitoh T, Maruyama K, Ishii KJ, Barber GN, et al. DNA damage sensor MRE11 recognizes cytosolic double-stranded DNA and induces type I interferon by regulating STING trafficking. Proc Natl Acad Sci U S A 2013; 110:2969 - 74; http://dx.doi.org/10.1073/pnas.1222694110; PMID: 23388631
- Unterholzner L, Keating SE, Baran M, Horan KA, Jensen SB, Sharma S, et al. IFI16 is an innate immune sensor for intracellular DNA. Nat Immunol 2010; 11:997 - 1004; http://dx.doi.org/10.1038/ni.1932; PMID: 20890285
- Yang P, An H, Liu X, Wen M, Zheng Y, Rui Y, et al. The cytosolic nucleic acid sensor LRRFIP1 mediates the production of type I interferon via a beta-catenin-dependent pathway. Nat Immunol 2010; 11:487 - 94; http://dx.doi.org/10.1038/ni.1876; PMID: 20453844
- Zhang Z, Yuan B, Bao M, Lu N, Kim T, Liu YJ. The helicase DDX41 senses intracellular DNA mediated by the adaptor STING in dendritic cells. Nat Immunol 2011; 12:959 - 65; http://dx.doi.org/10.1038/ni.2091; PMID: 21892174
- Ablasser A, Bauernfeind F, Hartmann G, Latz E, Fitzgerald KA, Hornung V. RIG-I-dependent sensing of poly(dA:dT) through the induction of an RNA polymerase III-transcribed RNA intermediate. Nat Immunol 2009; 10:1065 - 72; http://dx.doi.org/10.1038/ni.1779; PMID: 19609254
- Roos AK, Eriksson F, Walters DC, Pisa P, King AD. Optimization of skin electroporation in mice to increase tolerability of DNA vaccine delivery to patients. Mol Ther 2009; 17:1637 - 42; http://dx.doi.org/10.1038/mt.2009.120; PMID: 19532140
- Kolumam GA, Thomas S, Thompson LJ, Sprent J, Murali-Krishna K. Type I interferons act directly on CD8 T cells to allow clonal expansion and memory formation in response to viral infection. J Exp Med 2005; 202:637 - 50; http://dx.doi.org/10.1084/jem.20050821; PMID: 16129706
- Farkas A, Kemény L. Interferon-α in the generation of monocyte-derived dendritic cells: recent advances and implications for dermatology. Br J Dermatol 2011; 165:247 - 54; http://dx.doi.org/10.1111/j.1365-2133.2011.10301.x; PMID: 21410666
- Shirota H, Petrenko L, Hattori T, Klinman DM. Contribution of IRF-3 mediated IFNbeta production to DNA vaccine dependent cellular immune responses. Vaccine 2009; 27:2144 - 9; http://dx.doi.org/10.1016/j.vaccine.2009.01.134; PMID: 19356618
- Ishii KJ, Kawagoe T, Koyama S, Matsui K, Kumar H, Kawai T, et al. TANK-binding kinase-1 delineates innate and adaptive immune responses to DNA vaccines. Nature 2008; 451:725 - 9; http://dx.doi.org/10.1038/nature06537; PMID: 18256672
- Desmet CJ, Ishii KJ. Nucleic acid sensing at the interface between innate and adaptive immunity in vaccination. Nat Rev Immunol 2012; 12:479 - 91; http://dx.doi.org/10.1038/nri3247; PMID: 22728526
- Kopfnagel V, Wittmann M, Werfel T. Human keratinocytes express AIM2 and respond to dsDNA with IL-1β secretion. Exp Dermatol 2011; 20:1027 - 9; http://dx.doi.org/10.1111/j.1600-0625.2011.01382.x; PMID: 22092578
- van Hogerlinden M, Rozell BL, Ahrlund-Richter L, Toftgård R. Squamous cell carcinomas and increased apoptosis in skin with inhibited Rel/nuclear factor-kappaB signaling. Cancer Res 1999; 59:3299 - 303; PMID: 10416581
- Lladser A, Mougiakakos D, Tufvesson H, Ligtenberg MA, Quest AF, Kiessling R, et al. DAI (DLM-1/ZBP1) as a genetic adjuvant for DNA vaccines that promotes effective antitumor CTL immunity. Mol Ther 2011; 19:594 - 601; http://dx.doi.org/10.1038/mt.2010.268; PMID: 21157438
- Curtsinger JM, Valenzuela JO, Agarwal P, Lins D, Mescher MF, Type I. Type I IFNs provide a third signal to CD8 T cells to stimulate clonal expansion and differentiation. J Immunol 2005; 174:4465 - 9; PMID: 15814665
- Le Bon A, Etchart N, Rossmann C, Ashton M, Hou S, Gewert D, et al. Cross-priming of CD8+ T cells stimulated by virus-induced type I interferon. Nat Immunol 2003; 4:1009 - 15; http://dx.doi.org/10.1038/ni978; PMID: 14502286
- Leitner WW, Bergmann-Leitner ES, Hwang LN, Restifo NP, Type I. Type I Interferons are essential for the efficacy of replicase-based DNA vaccines. Vaccine 2006; 24:5110 - 8; http://dx.doi.org/10.1016/j.vaccine.2006.04.059; PMID: 16725231
- Diebold SS, Schulz O, Alexopoulou L, Leitner WW, Flavell RA, Reis e Sousa C. Role of TLR3 in the immunogenicity of replicon plasmid-based vaccines. Gene Ther 2009; 16:359 - 66; http://dx.doi.org/10.1038/gt.2008.164; PMID: 19052633
- Leitner WW, Hwang LN, deVeer MJ, Zhou A, Silverman RH, Williams BRG, et al. Alphavirus-based DNA vaccine breaks immunological tolerance by activating innate antiviral pathways. Nat Med 2003; 9:33 - 9; http://dx.doi.org/10.1038/nm813; PMID: 12496961
- Näslund TI, Kostic L, Nordström EK, Chen M, Liljeström P. Role of innate signalling pathways in the immunogenicity of alphaviral replicon-based vaccines. Virol J 2011; 8:36; http://dx.doi.org/10.1186/1743-422X-8-36; PMID: 21261958
- Amos SM, Pegram HJ, Westwood JA, John LB, Devaud C, Clarke CJ, et al. Adoptive immunotherapy combined with intratumoral TLR agonist delivery eradicates established melanoma in mice. Cancer Immunol Immunother 2011; 60:671 - 83; http://dx.doi.org/10.1007/s00262-011-0984-8; PMID: 21327636
- Xiao H, Peng Y, Hong Y, Huang L, Guo ZS, Bartlett DL, et al. Local administration of TLR ligands rescues the function of tumor-infiltrating CD8 T cells and enhances the antitumor effect of lentivector immunization. J Immunol 2013; 190:5866 - 73; http://dx.doi.org/10.4049/jimmunol.1203470; PMID: 23610140
- Babiuk S, Mookherjee N, Pontarollo R, Griebel P, van Drunen Littel-van den Hurk S, Hecker R, et al. TLR9-/- and TLR9+/+ mice display similar immune responses to a DNA vaccine. Immunology 2004; 113:114 - 20; http://dx.doi.org/10.1111/j.1365-2567.2004.01938.x; PMID: 15312142
- Spies B, Hochrein H, Vabulas M, Huster K, Busch DH, Schmitz F, et al. Vaccination with plasmid DNA activates dendritic cells via Toll-like receptor 9 (TLR9) but functions in TLR9-deficient mice. J Immunol 2003; 171:5908 - 12; PMID: 14634101
- Klinman DM, Yamshchikov G, Ishigatsubo Y. Contribution of CpG motifs to the immunogenicity of DNA vaccines. J Immunol 1997; 158:3635 - 9; PMID: 9103425
- Coban C, Ishii KJ, Gursel M, Klinman DM, Kumar N. Effect of plasmid backbone modification by different human CpG motifs on the immunogenicity of DNA vaccine vectors. J Leukoc Biol 2005; 78:647 - 55; http://dx.doi.org/10.1189/jlb.1104627; PMID: 15961575
- Ohlschläger P, Spies E, Alvarez G, Quetting M, Groettrup M. The combination of TLR-9 adjuvantation and electroporation-mediated delivery enhances in vivo antitumor responses after vaccination with HPV-16 E7 encoding DNA. Int J Cancer 2011; 128:473 - 81; http://dx.doi.org/10.1002/ijc.25344; PMID: 20309939
- Mills KHG, Dunne A. Immune modulation: IL-1, master mediator or initiator of inflammation. Nat Med 2009; 15:1363 - 4; http://dx.doi.org/10.1038/nm1209-1363; PMID: 19966773
- Roos AK, Eriksson F, Timmons JA, Gerhardt J, Nyman U, Gudmundsdotter L, et al. Skin electroporation: effects on transgene expression, DNA persistence and local tissue environment. PLoS One 2009; 4:e7226; http://dx.doi.org/10.1371/journal.pone.0007226; PMID: 19789652
- Oeckinghaus A, Hayden MS, Ghosh S. Crosstalk in NF-κB signaling pathways. Nat Immunol 2011; 12:695 - 708; http://dx.doi.org/10.1038/ni.2065; PMID: 21772278
- Prasad S, Ravindran J, Aggarwal BB. NF-kappaB and cancer: how intimate is this relationship. Mol Cell Biochem 2010; 336:25 - 37; http://dx.doi.org/10.1007/s11010-009-0267-2; PMID: 19823771