Abstract
Gap junctions and connexins are critical for coordinating cellular functions in complex epithelia. In recent years there has been increased interest in understanding the regulation and function of gap junctions in both the testis and epididymis. Studies in transgenic mice in which connexin 43 (Cx43) is mutated or is knocked down only in Sertoli cells have demonstrated the essential role of Cx43 in spermatogenesis and differentiation of Sertoli cells. In the epididymis developmental studies have shown a role for numerous connexins in the differentiation of epithelial cells and communication between the basal cells and both principal and clear cells. In both tissues several factors, such thyroid hormones and androgens, are important in regulating expression and function of connexins. Pannexins, which form cellular channels but are structurally similar to gap junction proteins, have been identified in both testis and epididymis and, in the epididymis, are regulated by androgens. The objective of this review is to summarize the advances that have been made on the role and regulation of connexins and pannexins in the testis and epididymis and their implication in spermatogenesis and sperm maturation.
Introduction
Junctional complexes between cells are comprised of adherens, tight and gap junctions. Adherens junctions are composed of cadherins and nectins, and serve for cell adhesion, as the name implies, and for cell recognition, and intracellular signaling. Tight junctions, composed principally of occludin and claudins, restrict paracellular movement of ions by forming an impermeable barrier. Gap junctions are essential structural components that mediate direct communication between neighboring cells. Intercellular communication using gap junctions is critical for several processes including cell growth and differentiation.Citation1,Citation2 Histologically, gap junctions appear as punctate structures between adjacent cells () and are formed by homomeric or heteromeric hemichannels, referred to as connexons.Citation3 Each connexon is comprised of an oligomer of six connexins (Cx) (). These channels allow the free passage of ions and small molecules of less than 1kDa between adjacent cells. In mammals, there are 21 different Cxs in humans; 20 different Cxs have been identified in other mammals and these have been broadly subdivided into five subfamilies.Citation4,Citation5 Connexons can be composed of either the same Cxs (homotypic) or different Cxs (heterotypic; ).Citation5 Not all Cxs can interact with each other to form heteromeric connexons. For example, Cx26 can form heteromeric channels with Cx30 and Cx32, but not with Cx40.Citation6 One of the functions of Cxs within a connexon is to provide selectivity to the gap junction. This has been shown in experiments in which Cx43 was replaced by Cx26 in transgenic mice resulting in, among other things, the inhibition of spermatogenesis, abnormal mammary gland development and altered cardiac functions.Citation7 Therefore, the spatio-temporal expression of specific Cxs is critical for the development and coordination of cellular events within epithelia. In addition to the makeup of the connexon, connexons from one cell can also bind to connexons comprised of different Cxs, resulting in a gap junction that has different permeability properties from two connexons composed of the same Cxs.Citation5,Citation8
Figure 1. Schematic diagram representing the appearance and structural composition of gap junctions. A. Immunofluorescent photomicrograph of Cx32 in the rat epididymis. The gap junctions appear as punctate (green) structures between adjacent epithelial principal cells. Each punctate structure represents hundreds of connexons that form the gap junctional plaque. The white dotted line outlines a principal cell while the yellow dotted line outlines a basal cell. B. Each connexon, or hemichannel, is comprised of six Cxs. Connexons can be comprised of the same Cxs (homotypic) or different Cxs (heterotypic). The connexins which make up the connexon determine its permeability. C. Connexins are transmembrane proteins that have two extracellular loops. Each extracellular loop has three cysteines (C) which allow connexons from different cell types to dock with another connexon to form and intercellular pore.
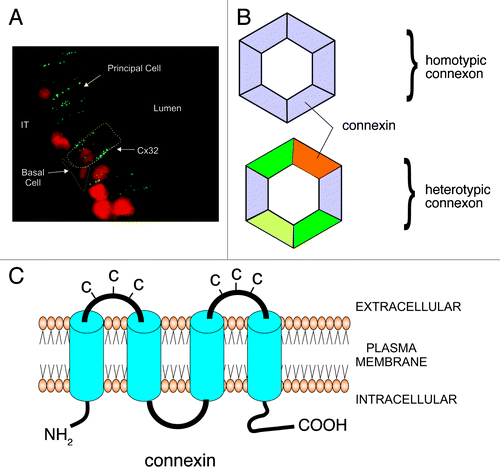
Connexons are assembled in the Golgi and transported to the plasma membrane. Depending on the tissue, some connexons will open, forming a hemichannel that can release small molecules into the extracellular space. Connexons will dock with connexons from adjacent cells forming an intercellular pore. These pores will aggregate to form a gap junction plaque. Connexons have a relatively short half-life of less than one hour and will be internalized into one of the two cells forming an annular junction. The annular junction, or connexosome, will then be degraded by lysosomes and proteosomes ().Citation1-Citation3 With the exception of Cx26, all Cxs are phosphorylated.Citation3 Alterations in the phosphorylation state of Cxs will influence the selectivity of the connexon. In general, both hyper- and hypo-phosphorylated Cxs will result in closing the connexon and decreasing intercellular communication.Citation3,Citation8 Intracellular pH is also a regulator of connexon function, since decreased intracellular pH is associated with loss in intercellular communication.Citation9 The physiological significance of this, however, may be limited, and whether or not intracellular pH plays a role in the gap junctions of reproductive tissues is unclear.Citation9
Figure 2. Schematic representation of the formation and degradation of gap junctions. Following their synthesis, Cxs enter the Golgi where the connexons are assembled. The connexon is then transported and assembled into the plasma membrane. Open connexons will form gap junctional hemichanels that release small molecules, such as ATP, Ca2+, into the extracellular space. Connexons dock with connexons from the adjacent cells to form an intercellular pore that will allow small molecules of less than 1 kDa to be exchanged between cells. The pores aggregate to form the gap junctional plaque. The connexons are then internalized into one of the cells to form an annular junction that will be degraded by lysosomal degradation.
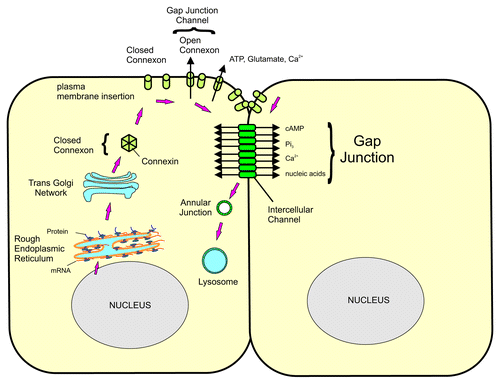
The Testis
In the testis, intercellular gap junctional communication is essential for the exchange of cellular messengers necessary for spermatogenesis.Citation10-Citation13 The first study reporting the presence of Cxs in the testis was done by Risley and Tan,Citation14 who identified the presence of Cx43 in both Leydig cells and Sertoli cells. They also reported the presence of Cx26 and Cx32 in the apical region of the seminiferous tubule. Dye-coupling experiments indicated that cellular communication between adjacent Sertoli cells was non-selective, while communication between Sertoli cells and developing germ cells favored the passage of positively-charged biotin tracers.Citation14 This exchange was asymmetric, since that dye could be exchanged from Sertoli cells to spermatogonia but not from spermatogonia to Sertoli cells. The expression of Cx43 in the seminiferous tubule is stage-dependent, suggesting differences in gap junctional communication between Sertoli cells and germ cells of different maturational stage.Citation15 The importance of gap junctional communication, especially Cx43, in the testis was shown with mice lacking Cx43.Citation16 While these animals died at birth due to cardiac insufficiency, there was an absence of developing germ cells in the testis of developing embryos.Citation16 To further understand the consequence of the absence of Cx43 in the testis, testes from Cx43-null mice were grafted under the kidney capsule of wildtype mice.Citation17 The germ cells in the seminiferous tubules of transplanted testes of wildtype mice underwent meiotic division, but those of Cx43-null mice failed to develop, resulting in Sertoli cell-only testes.Citation17 Similar results were observed in adult mice having a selective deletion of Cx43 in Sertoli cells.Citation18,Citation19 These mice had significantly smaller testes, which contained only Sertoli cells. Furthermore, both Sertoli cells and spermatogonia were actively proliferating in the adult, in contrast to normal mice, in which Sertoli cells differentiated and stopped proliferating at two weeks of age, when the blood-testis barrier was formed.Citation18,Citation19
Connexins regulate the selective permeability of the connexon, and consequently the gap junction. Plum et al.Citation20 replaced the coding region of Cx43 with that of either Cx32 or Cx40. While the knock-in mice had some serious issues and early mortality, spermatogenesis was compromised in both transgenic animal lines. While the testes had primary spermatogonia, the seminiferous tubules were devoid of more advanced germ cells. This implies that Cx43 cannot be replaced by either Cx32 or Cx40 for spermatogenesis to proceed. An interest point however, is that in these transgenic mice, germ cell populations were decreased in embryos. Since this occurs before the formation of the blood-testis barrier (postnatal day 14 in the mouse and rat), and the differentiation of Sertoli cells, it is clear that Cx43 also plays a role in embryonic germ cell recruitment. Francis and LoCitation21 reported an increase in primordial germ cell apoptosis in Cx43 knockout mice. This was associated with a decrease in the expression of β1-integrin which is necessary for primordial germ cell migration. These may explain the reduced number of germ cells observed in the testis of Cx43 knockout mice as well as Cx43 knockout mice with either Cx32 or Cx40 knock-in.Citation16,Citation20
A recent study examined the effects of altered Cx43 function using a mouse model of oculodentodigital dysplasia (ODDD), in which there is a mutation in one of the extracellular loops of Cx43.Citation22 In humans, ODDD is caused by mutations in Cx43 that are inherited in an autosomal fashion.Citation23 This dysmorphogenesis syndrome is characterized by oligosyndactyly of fingers and toes, abnormal cranial features, and physiological problems associated with cardiac and neurological functions, hearing, and skin.Citation23 In the testis and epididymis of the mouse ODDD model,Citation22 the G60S mutation resulted in a decreased level of phosphorylated Cx43 as well as decreased intercellular communication.Citation24 While the males were fertile, there was an increase in levels of germ cell apoptosis, abnormal spermatogenesis, and the presence of many degenerating seminiferous tubules.Citation24 Epididymal sperm counts were also reduced, as were total percent motility and several sperm velocity parameters, suggesting that the mice were subfertile. These results confirmed previous findings on the importance of Cx43 in spermatogenesis, but also indicated that the presence of Cx43 was not sufficient to maintain spermatogenesis, and that functional communication between Sertoli cells and germ cells was necessary.
Thyroid hormones (TH) play a critical role in the differentiation of Sertoli cells.Citation25 Studies have indicated that treatment of rats with the anti-thyroid drug n-propylthiouracil (PTU) during pubertal development resulted in increased testicular size and daily sperm production as a result of an increased number of Sertoli cells in the testis.Citation25-Citation27 St-Pierre et al.Citation28 showed that neonatal PTU treatment was associated with a decrease in Cx43 levels and with cytoplasmic localization of Cx43 in the testis. Gilleron et al.Citation29 using cultured Sertoli cells, then showed that TH regulated the localization of Cx43 to the plasma membrane, and that triiodothyronine (T3) could decrease Sertoli cell proliferation. These two studies support the notion that TH regulate Sertoli cell differentiation via Cx43. Interestingly, in Sertoli specific Cx43-null mice, the TH receptor α (THRα) remains elevated throughout adulthood.Citation18 In normal mice, THRα is high prior to the differentiation of Sertoli cells and decreases thereafter.Citation30,Citation31 TH therefore regulate Cx43 levels in Sertoli cells; once Cx43 levels increase, there is an inhibition of TH receptor levels and therefore decreased TH action on Sertoli cells. This close association between TH and Cx43 suggests that Cx43 is the main target of TH on the testis. Once Cx43 levels increase and Sertoli cells differentiate, the actions of TH are no longer necessary and the system is shut down, or at the very least, significantly decreased.
The regulation of Cx43 by TH in Sertoli cells is highly conserved in evolution and has been shown in fish.Citation32,Citation33 Both the mammalian and fish Cx43 promoters contain TH response elements, and the regulation of Cx43 appears to occur via direct transactivation of the GJa1 gene.Citation33,Citation34 In studies using immature fish exposed to municipal wastewater effluent containing estrogens and estrogenic-like substances, deMontgolfier et al.Citation35 showed that trout testicular Cx43 and Cx31 increases precede changes in testicular maturation. This observation suggests that changes in Cxs may be a driving force in regulating germ cell development during spermatogenesis.
In humans, correlations have been reported between decreased Cx43 levels and infertility in azoospermic men.Citation36 Studies have also reported decreased Cx43 in in situ carcinoma, in patients with Klinefelter’s syndrome, as well as in infertile patients that lack a portion of the Y-chromosome and have arrested spermatogenesis.Citation37,Citation38 What remains to be elucidated, however, is whether or not changes in Cx43 precede effects on spermatogenesis in mammals, as is suggested in fish studies, or if these occur as a result of changes in spermatogenesis. If the former is the case, then Cx43 could be a potential therapeutic target for certain cases of azoospermia due to testicular failure. However, given the ubiquitous distribution of Cx43 throughout the different organs of the body, selectively targeting the testis represents a formidable challenge.
The exact role of Cx43 in spermatogenesis has received considerable attention, although its role(s) remain to be elucidated. Cx43 connexons form gap junction plaques between adjacent Sertoli cells as well as between Sertoli and germ cells.Citation6 A possible mechanism by which Sertoli cells may regulate intercellular communication could be regulating the turnover, or internalization of gap junction plaques. It has been shown that c-Src is involved in the internalization of gap junction plaques in Sertoli cells.Citation39 The interaction between c-Src and Cx43-containing gap junction plaques appear to be regulated by dynamin, which can regulate the association of c-Src with Cx43 and appears regulated by the large GTPase dynamin2.Citation40 Whether or not this process is activated and deactivated during spermatogenesis and whether or not it represents a critical role in the regulation of spermatogenesis still needs to be demonstrated.
In the adult murine testis, Cx43 co-localizes with the tight junction protein occludin in the area of the blood-testis barrier.Citation41,Citation42 More recently, it has been shown that Cx43 is important for the formation of tight junctions between Sertoli cells, responsible for creating the blood-testis barrier.Citation13,Citation43-Citation45 Li et al.Citation44 have reported that Cx43, along with plakophilin2, can alter the transepithelial resistance of Sertoli cells in vitro, suggesting that these two proteins interact in the regulation of tight junctions. However, in mice lacking Sertoli cell-specific Cx43, a blood-testis barrier was formed.Citation43 Furthermore, levels of occludin, N-cadherin and β-catenin were all increased, while ZO-1 levels were decreased. In the same study, similar results were obtained using Sertoli cells in which Cx43 levels were knocked down using antisense siRNA. While these results suggest that having a functional blood-testis barrier is not the only requirement for spermatogenesis to proceed, the question arises as to whether or not the barrier is, in fact, fully patent and functional. Alterations in adherens junctions indicate that the dynamic nature of the blood-testis barrier is altered in Cx43 Sertoli-null mice. The tight junctions of the blood-testis barrier are unique in that developing germ cells must be transported across the tight junctions from the basal to the adluminal compartment. Thus, the dynamic nature of testicular tight junctions requires precise interactions between various types of cellular junctions to coordinate these processes. However, the question remains as to how Cx43 gap junctions accomplish this. Perhaps the asymmetric intercellular communication between Sertoli cells and germ cells reported by Risley et al.Citation14 may activate signals from the germ cells back to the Sertoli cells to regulate both the adherens and tight junctions of the blood-testis barrier.
Several studies have reported that cadherins and other components of adherens junctions are necessary for gap junction formation. Increased E-cadherin-dependent cell–cell contacts are associated with increased gap junctions.Citation46 Cx43 co-localizes with N-cadherin (cadherin 2) in NIH3T3 cells, and these immunoprecipitate together, suggesting that they form a multi-protein complex.Citation47 Furthermore, anti-N-cadherin antibodies prevent gap junctional communication in these cells,Citation48 suggesting a crucial interaction between these two types of cellular junctions. Similar regulation may occur in the testis which also expresses N-cadherin and Cx43.Citation49-Citation52
Interaction of ZO proteins with various Cxs, including Cx43, is well-characterized.Citation53-Citation55 ZO proteins appear to be important in the localization of gap junctions and/or in recruiting signaling molecules that regulate intercellular communication. Several studies have demonstrated that Cxs interact with other tight junctional proteins, such as occludin and claudins, in a variety of tissues such as liver and endothelial cellsCitation56-Citation58 as well as in the testis, where occludin is colocalized with Cx43 in Sertoli cells in the area of the blood-testis barrier.Citation41 Studies have also shown that Cxs can modulate tight junction functions. Increased expression of Cx32 in hepatocytes increased the number of tight junctional strands and expression of occludin, CLDN1 and -2 and MAGI-1, while decreasing paracellular permeability.Citation56,Citation57,Citation59 Similar observations have been reported for Cx26 overexpression in Caco-2 cells, which increases CLDN4 levels.Citation60 Whether or not the permeability of the blood-testis barrier is changed in mice where Cx43 is knocked down in Sertoli cells remains to be established, but given the extensive literature on the role of Cldns in paracellular transport of specific ions, and the close interaction between adherens junctions and tight junctions, it would not be surprising that the permeability of the barrier may be altered in these mice, resulting in, or contributing to, the inhibition of spermatogenesis observed in these testes.Citation18,Citation19,Citation43
Leydig cells also have gap junctions and express Cx43.Citation14,Citation61,Citation62 The physiological functions of Leydig cells and their response to LH appears to be unaltered by lack of Cx43.Citation17,Citation63 Whether or not there are other Cxs in Leydig cells remains to be established and whether or not these may compensate for Cx43 in knockout animals is unknown.
Clearly, all of these studies point to the critical nature of gap junctional communication and connexins in spermatogenesis. The challenge now is how to use this information in the development of therapeutic strategies for treatment of male infertility or in controlling male fertility, particularly since Cx43 is ubiquitously expressed throughout the body.
The Epididymis
Early studies have revealed that sperm were not simply retained in the epididymis to allow for “aging,” but rather that the functional maturation of sperm resulted from their exposure to the luminal environment.Citation64,Citation65 Thus, the ability of the epididymis to provide the appropriate milieu for maturation of spermatozoa is considered one of its most important functions.Citation66-Citation70 This milieu is created by several processes, notably the absorptive and secretory activities of the epithelial cells which line the duct, many of which are androgen-dependent. The primary function of the epididymis is to provide a defined environment necessary for sperm maturation. This environment involves proteins secreted directly by epididymal epithelial cells. These proteins interact with maturing spermatozoa, and contribute to acidification of the lumen, as well as to detoxification of free radicals and ion/organic molecule composition.Citation69 The composition of the lumen changes along the length of the epididymis, presumably to favor the final stages of sperm maturation and to maintain the sperm in a quiescent state until they are ejaculated.Citation69 Coordinating cellular and physiological functions along the epididymis is therefore critical to these functions. Morphologically and physiologically, the epididymis can be subdivided into at least four distinct regions: the initial segment, the caput, the corpus, and the cauda.Citation69 The epididymal tubule is lined with a pseudostratified epithelium that contains five major cell types: principal, basal, clear, halo, and narrow cells. The most abundant cell type found in the epididymal epithelium is the principal cell.Citation69 Epithelial principal cells are the major protein-secreting cells of the epididymis. Tight junctions between epididymal epithelial cells are responsible for the formation of the blood-epididymis barrierCitation66,Citation67,Citation71 (). The barrier is composed of numerous tight junction proteins including occludin and multiple claudins.Citation72-Citation74 Claudins have been shown to be critical for regulating paracellular transport between adjacent cells and the variations in the expression of claudins along the epididymis suggest important differences in tight junction physiology along the epididymis.Citation67,Citation75 Segment-specific differences in epididymal physiology are suggestive of a complex coordination of function. Thus, cellular communication is critical to epididymal function, and, as in other tissues, is regulated by gap junctions.
Gap Junctions in the Epididymis
Epididymal gap junctions were initially identified in epithelial principal cells of the rat using freeze fracture electron microscopy.Citation76 They reported that macular gap junctions were present in the apical region of the epithelial cells and these were associated with the tight junctions of the blood-epididymal barrier. They also noted that gap junctions were present in the basal compartment of the epithelial cells. Pelletier,Citation77 using freeze fracture electron microscopy in the mink, a seasonal breeder, showed that gap junctions were present basal to the tight junctions but also at the basal region of principal cells. He also reported that gap junctions were present between principal and clear cells.
Cx43 was the first connexin to be identified in the epididymisCitation78 and was shown to be localized between principal and basal cells. Cx43 was also present between clear and basal cells and between myoid cells, particularly in the distal regions of the epididymis. These data were interesting, since Cx43 was not observed between adjacent principal cells or between principal and clear cells, implying that the coordinated functions of the epididymal epithelium involved direct interactions with basal cells. Since specific Cxs confer the selective properties of the connexons, these data also suggested that the molecules exchanged between adjacent principal cells, or between principal and clear cells, differed from those exchanged between principal and basal cells, since Cx43 was not present between adjacent principal cells or between principal and clear cells.
It is interesting to note that in efferent ducts, which lack basal cells, Cx43 was localized apically between ciliated epithelial cells.Citation78 However, in the initial segment of the epididymis, where basal cells were present, Cx43 was localized between principal and basal cells. These observations suggest that the presence of basal cells regulate, or at the very least influence, intercellular communication in the epididymis. Studies have reported that basal cells may regulate certain physiological aspects of principal and clear cells via prostaglandins. Thus the role of Cx43 in epididymal physiology may be more complex than previously thought. When discussing epididymal physiology, there is tendency to examine it from the lumen toward the base of the epithelium; perhaps we should consider the alternative perspective: that is, regulation from the blood toward the lumen, i.e., from the base upwards. Certainly if this is the case for regulation of epididymal function, then it makes sense that basal cells would be a primary target of blood-borne regulators.
The localization of Cx43 between principal and basal cells has now been observed in several species, including the water buffalo, the stallionCitation79,Citation80 and human (unpublished). This would suggest that basal-principal cell intercellular communication appears to be conserved between species, supporting its importance for epididymal function.
In addition to Cx43, epididymal cells of the rat also express transcripts for Cx26, 30.3, 31.1, and 32.Citation81 In the proximal region of the epididymis, Cx26 is expressed primarily in young animals, when the epithelium is undifferentiated, and is localized to the apical region between epithelial cells.Citation81 In contrast, Cx32 is expressed in the epididymis of adult rat between adjacent principal cells, as well as between principal and basal cells.Citation81 This implies that Cx32-mediated gap junctions are involved in direct communication between adjacent principal cells. The localization of Cx26 and Cx32 along the lateral plasma membrane supports previous observations of the presence of gap junctions between these cells.Citation77,Citation82
Postnatal developmental events in the epididymis have been well characterized. In the rat, cellular differentiation occurs between days 16 and 44Citation83,Citation84 and basal cells are present in the epididymis by day 28.Citation84 As epididymal epithelial cells differentiate into columnar and then into principal cells, there is a correlation between levels of Cx26 mRNA in the proximal region of the epididymis and the degree of differentiation.Citation81,Citation83,Citation84 As Cx26 levels decrease, there are concurrent increases in both Cx32 and Cx31.1 mRNA levels. This switch in expression from Cx26 to either Cx32 or Cx31.1 has been reported for keratinocytes during differentiation.Citation85 Epididymal Cx43 mRNA levels during rat postnatal development display a similar pattern as Cx26, but the levels do not decrease as dramatically as those of Cx26. This may be linked to the appearance of basal cells by day 28, since Cx43 mediates the coupling of basal and both principal and clear cells, and may explain why the pattern of Cx43 differs from that of Cx26 during development.
In contrast to the proximal region of the epididymis, the postnatal pattern of Cx31.1 levels in the cauda is low at day 7 and increases rapidly to peak at approximately day 28.Citation81 Cx32 mRNA levels, on the other hand, are not detectable at day 7 and peak by day 63. However, Cx43 mRNA levels in the cauda remain unchanged and Cx26 levels are below detection level. These data support the notion that epididymal Cxs are regulated not only in segment-specific manner, but also independently of one another.
Regulation of epididymal connexins
There is still limited information of the regulation of gap junctions or of Cxs in the epididymis. One of the reasons for this has been the absence of reliable cellular tools that can be used to understand regulatory mechanisms in the epididymis. The development of epididymal cells lines from canine, murine, rat and from both fertile and infertile human epididymisCitation86-Citation92 should provide more rapid advances in the regulation of epididymal cell and molecular biology including the regulation of Cxs.
Studies in the rat have shown that the levels, phosphorylation status and cellular targeting of Cx43 in the initial segment of the epididymis are androgen-dependent.Citation78 As indicated previously, Cx43 in intact adult rats is located between principal and basal cells. However, in orchidectomized rats, Cx43 is also localized between adjacent principal cells at their apical margins. This change in cellular targeting is lost in orchidectomized rats when testosterone levels are maintained at physiological levels. These results indicate that androgens are key regulators of Cx43 in the epididymis and that cellular targeting of Cx43 is also regulated. These observations further suggest that the regulation of intercellular communication in the initial segment is different from other regions. Other studies have reported that claudin-1 (CLDN1), a tight junction protein, is also regulated differently in the initial segment of the epididymis.Citation72 Since both Cx43 and CLDN1 bind to ZO-1, there may be certain commonalities in the regulation of these two proteins in the initial segment, as opposed to other regions of the epididymis. Certainly in the rat, the appearance of the apical junctional complex appears to be much more extensive in the initial segment than in other regions of the epididymis.Citation71
In the boar, Lydka et al.Citation93 reported that the administration of the anti-androgen flutamide during gestation resulted in decreased Cx43 levels in the cauda epididymidis during gestation. This decrease, which persisted to adulthood, was associated with a decrease in androgen receptor levels, which were more pronounced in the cauda, and an increase in apoptosis. The results support the fact that androgens represent an important regulator of Cx43 in the epididymis. Using orchidectomized rats in which the epididymis is regressed and stimulating the epididymis with dihydrotestosterone (DHT), Hamzeh and RobaireCitation94 showed that Cx31 is androgen-dependent. Their analyses also predicted that epididymal Cx43 is regulated by EGF (epidermal growth factor) and IGF via endothelin1 and IGFBP3 (IGF binding protein 3).
Studies in the testis have reported that TH are essential regulators of Cx43 and of the subsequent differentiation of Sertoli and Leydig cells.Citation25,Citation95-Citation98 In the epididymis, PTU-induced neonatal hypothyroidism resulted in a significant decrease in Cx43 levels in the proximal region of the epididymis.Citation28 Once PTU treatment was stopped, the levels of Cx43 returned to control levels.Citation28 Cx43 levels were not regulated by TH in the cauda epididymidis. As with orchidectomy and testosterone replacement, CLDN1 levels in the proximal epididymis were also decreased by hypothyroidism.Citation28 Thus, the relationship between gap and tight junctions appears to be finely regulated in epididymal function.
Functional regulation of epididymal connexins
Data from recent studies using a transgenic mouse model for human ODDD, in which there is a mutation in one of the extracellular loops of Cx43, indicated that overall levels of both Cx43 and phosphorylated Cx43 were significantly decreased in the epididymis.Citation24 Surprisingly, no consistent phenotype was evident in the epididymis of these animals. Although unexpected, other studies have shown decreased Cx43 levels in human patients with ODDD, but tissues such as skin generally appear to have a normal morphology.Citation99 Functionally, however, skin fibroblasts display reduced proliferation and migration capabilities, and also express less α smooth muscle actin, suggesting that they are generally ineffective in differentiating.Citation99
While the morphology of the epididymal epithelium in ODDD transgenic mice was not altered in a consistent manner, however sperm motility was significantly decreased in these animals.Citation24 Since sperm motility is acquired during epididymal transit, we speculated that there may be physiological alterations in epididymal functions that result from the Cx43 mutation and these may be responsible for, or at least contribute to, observed differences in sperm motility and velocity parameters.
Connexin Hemichannels
While the distribution of Cxs in the formation of gap junctions, in spermatogenesis, and in epididymal function is well-established, it has been generally held that connexons reached the plasma membrane in a closed state until ‘docking’ with opposing connexons. There is increasing information, however, that connexons may reach the plasma membrane in an ‘open’ configuration, thereby forming a large open pore, or channel.Citation100,Citation101 These channels, or hemichannels, appear to be involved in the release of ions and metabolic products such as ATP from the cell.Citation100,Citation102,Citation103 In osteocytes, stimulating the opening of Cx43-hemichannels results in the release of prostaglandin E2.Citation104 Different studies have suggested that connexons of several Cxs may have the ability to form hemichannels (Cx23, 26, 30, 302.2, 31.9, 32, 36, 37, 41.8, 43, 45, 45.6,46, 50, 55.5 and 56).Citation100,Citation105,Citation106 The strongest evidence of hemichannel formation has been from a study showing that Cx46 and 50 can form hemichannels in transfected Xenopus oocytes.Citation107 There is very little information to date as to whether or not Cxs can form hemichannels in the male reproductive tract.Citation12 However, since the testis, epididymis and prostate all express many of the Cxs known to form, or suspected of forming, hemichannels, the possibility remains that Cx-based hemichannels exist in the male reproductive tract.
Several studies have reported the presence of functional Cx hemichannels in the kidney and their role in the regulation of ATP release, as well as in the regulation of ion secretion and the control of blood volume and pressure.Citation108,Citation109 Given the many similarities and common embryonic origin of the epididymis and the kidney,Citation68,Citation69 it is possible, and even likely, that Cx-based hemichannels exist in the epididymis. One can postulate that the role of such hemichannels may be implicated in the regulation of the composition of the epididymal lumen and that it may act in concert with the blood-epididymis barrier in regulating the epididymal luminal milieu that in turn allows for sperm maturation. A second potential function of these hemichannels may be in contributing to cell-cell paracrine signaling in the epididymis. Given the requirements to coordinate cellular functions in the epididymis in terms of modulating segment-specific physiological functions, the involvement of several factors or pathways are likely.
As indicated above, Cx43 is perhaps the best studied Cx in the male reproductive tract. Using HeLa cells as an experimental model, it has been shown that Cx43 transfection can alter membrane polarization, suggesting the occurrence of Cx43 hemichannels.Citation110 Likewise, in osteocytes in vitro, Cx43 hemichannels can be stimulated to open, and these open hemichannels release PGE2.Citation104 Since prostaglandins in the epididymis are synthesized in basal cells and act on principal cells,Citation111 it is intriguing that Cx43 localizes between basal and principal cells. Since prostaglandins exert their effects via plasma membrane receptors, it is possible that Cx43 hemichannels in basal cells release PGE2 to act on principal cells. However, while Cx43 hemichannels can occur under in vitro conditions, are these functional in vivo? Most of the Cx hemichannels have been observed to be open under ischemic conditionsCitation112-Citation114 and strong depolarization (+40 mV).Citation110,Citation115 Furthermore, Cx hemichannels are closed when intracellular Ca2+ levels are elevated, or in the presence of physiological levels of extracellular Ca2+, and under conditions in which the plasma membrane is depolarized, suggesting that Cx hemichannels may not be functional under normal physiological conditions.Citation116-Citation118 Thus, the exact physiological role of Cx-based hemichannels remains contentious.Citation106,Citation119 If these are functional physiologically, they likely occur in either a tissue- or cell-specific manner and under specific physiological conditions. Addressing this issue remains a challenge for understanding the functions of Cxs in the reproduction.
Pannexin Channels
In the past few years, a new family of three proteins, the pannexins (Panxs), that are structurally similar to innexins, the invertebrate gap junction proteins, has been identified. While Panxs share sequence homology with innexins, they lack sequence homology with the Cxs and, unlike Cxs, are heavily glycosylated.Citation120,Citation121 However, Panxs share the structural topology common to all members of the gap junction protein superfamily.Citation122-Citation124 As a result of their homology to innexins,Citation125,Citation126 Panxs were initially thought to form gap junctions. Studies suggested that Xenopus oocytes transfected with Panx1, but not Panx2, could form gap junctions when pairs of oocytes were incubated over a long period of time.Citation127 However, this was not observed in any other cell type and it is generally accepted that Panxs form hemichannels exclusively.Citation128
Panxs are integral transmembrane proteins with 4 transmembrane domains; each of the two extracellular loops contain 2 cysteine residues.Citation100,Citation128,Citation129 The cytoplasmic NH3-terminal is highly conserved between different Panxs, while the COOH -terminal region varies quite a bit between Panxs.Citation122 Transient expression of Panx proteins has demonstrated that they accumulate in the RER and the Golgi apparatus, suggesting that Panx channels, or pannexons, are assembled and transported to the plasma membrane through the same secretory pathway as the majority of Cxs.Citation123 However, unlike Cxs, which are phosphoproteins, Panxs are glycosylated as they transit the RER and Golgi apparatus. This modification is required for the transport of pannexons to the plasma membrane and appears to preclude close interactions between pannexons.Citation120,Citation121,Citation130 Panx1 has been the most studied Panx and, in addition to its ability to assemble into homomeric hemichannels, it has also been demonstrated to form heteromeric hemichannels along with Panx2.Citation127
The ability of Panxs to assemble into hemichannels and the gating properties of these channels have been studied in Xenopus oocytesCitation131 as well as in mammalian cell lines.Citation132,Citation133 It has been demonstrated that the physiological properties of vertebrate Panx channels are distinct from those of Cxs and ensure that a unique, rather than a redundant, cellular role can be assigned to this protein family. Upon activation, pannexons open into large non-selective pores permeable to ions, small molecules and metabolites up to 1kDa over a wide range of depolarization levels, and have been shown to be insensitive to physiological levels of extracellular Ca2+. In addition, Panx hemichannels differ from typical Cx channels by exhibiting much slower cellular dynamics, larger currents with increasing depolarization, faster kinetics of pore opening, larger unitary conductance, very weak voltage gating and multiple substrates in single-channel recordings.Citation123,Citation131,Citation134 The presence of Panx2 with Panx1 in heteromeric hemichannels modified properties of the channels, since currents with smaller amplitudes were recorded and peak currents were significantly delayed compared with currents measured in Panx1 mRNA injected cells.Citation127
There now exists considerable evidence supporting a role for Panxs in the formation of hemichannels, as opposed to gap junctions, in non-junctional regions of the plasma membrane.Citation127,Citation129 In addition, both the localization and functional properties of Panx1 hemichannels suggest that they are perfectly suited for Ca2+ propagation waves.Citation127,Citation132 Ca2+ wave propagation is a mechanism of cellular communication which consists of two distinct pathways: the first involves the diffusion of Ca2+-mobilizing second messengers, such as Ca2+ itself or Ins(1,4,5)P3, from the cytosol of one cell to that of another via gap junctional intercellular communication; and the second, which is extracellular, involves the diffusion of molecules released from one cell into the extracellular space, which act upon the cell surface membrane receptors of neighboring cells.Citation106 In many cell types, this extracellular paracrine/autocrine pathway is mediated by the release of ATP through vesicles and/or ion channels activating ATP-sensitive purinergic receptors (P2Rs), which can be further distinguished into metabotropic (P2Y) and ionotropic (P2X) receptors.Citation106 Although there is evidence suggesting that Cxs may also be involved in extracellular ATP release, a majority of Cx hemichannels has been shown to close following elevation of intra- and extracellular Ca2+ levels, or during membrane depolarization, therefore limiting their ability to participate in the extracellular Ca2+ wave propagation.Citation116,Citation117 Panx1 hemichannels open in association with an increase in intercellular Ca2+ and have been demonstrated to support a rapid ATP release, capable of generating the ATP-specific current across the membrane.Citation123 Therefore, the properties of Panx1 hemichannels support the notion that Panxs are physiologically more suitable than Cxs for ATP transport across the plasma membrane under a wider range of conditions, a critical process for controlling intracellular Ca2+ homeostasis and supporting efficient ATP release in non-contact Ca2+ wave propagation.Citation123
Panx1 channels have been demonstrated to physically interact and work in synergy with ionotropic and metabotropic purinergic receptors to support ATP-induced ATP release, the central event of regenerative Ca2+ wave propagation in many cell types.Citation123 The role of Panx1 in relation to this mechanism has been demonstrated in the production of nitric oxide by endothelial cells, causing relaxation of smooth muscle and hence vasodilationCitation123; in erythrocytes. where it is involved in the release of ATP in response to ischemia and mechanical stress trigger Ca2+ waves in the endotheliumCitation123; in gustatory stimulation in mammalian taste budsCitation133; in astrocytesCitation106; and during the early stage of the inflammatory response, where it associates with the purinergic receptors signaling cascade involved in the activation of caspase-1 and the processing of interleukin 1β (IL-1β) from macrophages.Citation134 In accordance with these results, Panx1 was found to be part of the P2X7-receptor complex that mediates dye uptake and the release of ATP from erythrocytes and taste bud cells.Citation106,Citation118,Citation133 It has also been proposed that Panx1 hemichannels observed in the rough endoplasmic reticulum (RER) could serve as functional pannexons for RER Ca2+ release channels, thus providing a conduit for the enigmatic Ca2+ leak across the RER membrane.Citation135 Panx1 may also play a role as a tumor suppressor and in neuronal necrosis following CNS ischemia.Citation123
In the male, transcripts and proteins for Panx1 and 3 in the testis and epididymis of the rat have been reported.Citation136 Three main transcripts of 532, 500 and 311bp were identified in the testis and epididymis. Sequence analysis of these transcripts indicated that the 311bp transcript has a 211 bp deletion corresponding to the 3rd exon of Panx1. This region corresponds to the second transmembrane domain and a portion of the cytoplasmic loop (). The Panx1 encoding-gene contains 5 exons and several introns, allowing for alternative splicing events.Citation127 It has been demonstrated that Panx1 has an alternatively spliced exon 5 which leads to a 4 amino acid insert within the intracellular C-terminus.Citation126 In addition, Ma and colleaguesCitation137 identified a novel difference in the intracellular C-terminus of human Panx1 consisting of the deletion of valine-377, encoded within exon 4. This suggests that Panx1 is regulated at the mRNA level, and a splice variant in which all of exon 3 is removed from the transcript occurs in the testis, in efferent ducts and in all regions of the epididymis. Exon 3 codes for the second transmembrane domain; thus, the predicted protein would essentially lack one of its four transmembrane spanning regions. At the moment, the function of this transcript is still unknown.
Figure 4. Schematic diagram representing the appearance and structural composition of pannexon channels. A. Immunofluorescent photomicrograph of Panx1 (left) and Panx3 (right) in the rat epididymis. Panx1 is localized between principal and basal cells while Panx3 is present at the apical region of the epithelium. B. Pannexons are composed of 6 to 8 pannexins. C. Pannexins are transmembrane proteins that have two extracellular loops of different lengths and a long carboxy terminal in the intra cellular space.
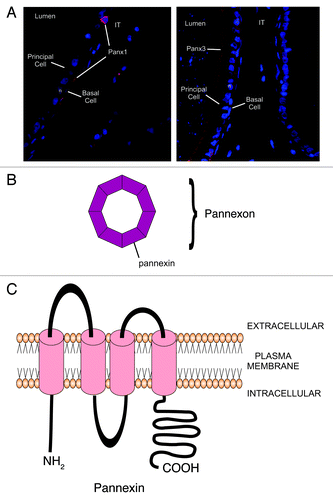
Not surprisingly, multiple Panx1 and Panx3 immunoreactive bands have been observed by western blot analyses, owing to the extensive glycosylation of these proteins.Citation120,Citation121,Citation136 Differences in glycosylation have been reported in the different regions of the epididymis, suggesting that the regulation and/or cellular processing of Panx1 and Panx3 occurs in a cell type- and region-specific manner with distinct subcellular localizations. In the testis, Panx1 is observed within the seminiferous epithelium, where it appears to be expressed by the Sertoli cells at the level of the blood-testis barrier and by peritubular myoid cells, while Panx3 localizes to the interstitial space in areas of Leydig cells. In the efferent ducts, both Panxs are expressed in the epithelium, notably at the apical region of ciliated cells, while lateral plasma membranes and the basal regions of non-ciliated and ciliated cells are reactive for both Panxs. In addition, in the case of Panx1 only, the peritubular myoid cells are reactive. In the epididymis, although both Panxs are expressed by principal cells, Panx1 localization is restricted to the basal region of the epithelium, while Panx3 is found at the apical region of the epithelium. Panx1 is also expressed by basal cells and myoid cells, while Panx3 localizes to halo cells. Cx-based GJs are known to cluster at the plasma membrane into dense arrays at locations of cell-cell contact. This is typically visualized as a punctate staining and is usually accompanied by a lack of cell surface staining in non-junctional areas.Citation3 This has been observed in many tissues, including the male reproductive tract.Citation78,Citation81,Citation138,Citation139 In contrast, the distribution profile of Panx1 and Panx3 reveals a relatively even cell surface distribution, with limited clustering at junctional areas. In addition, the two Panxs localize at non-junctional regions of the plasma membrane, with Panx1 at the basal region of epididymal principal cells, adjacent to the basement membrane and at times overlying basal cells, while Panx3 localizes to the apical region of principal cells, adjacent to the epididymal lumen. These findings are consistent with the distribution profile of Panx1 in non-GJ-forming erythrocytes and HeLa cells,Citation117,Citation133 and support the notion that Panxs are involved in the formation of functional single membrane channels in the male reproductive tract. As previously reported in some human tissues for Panx1,Citation121,Citation140 we also observed a punctate immunostaining for Panx3 in the testis. These data suggest that the organization of Panxs into junction-like complexes may occur in some tissues, including the male reproductive tract.
Studies in the epididymis have indicated that orchidectomy results in an increase in the number of Panx1 and Panx3 immunoreactive bands, as well as of total protein levels throughout the epididymis, while testosterone maintenance with orchidectomy reduces the number of bands in the caput and corpus. This suggests that testosterone and other testicular factors are involved in the post-translational modification of these Panxs. These effects were attenuated in the cauda, suggesting that the regulation of Panxs in this segment is distinct from other epididymal regions. While the use of orchidectomized animals does have inherent limitations as an experimental model, the regulation of Panxs appears complex and involves multiple testicular factors. Several other proteins have also been reported to be androgen-repressed in the epididymis.Citation141-Citation143
The function of Panxs in the testis and epididymis has yet to be clearly established. However, it has been reported that epididymal cells can release ATP into the lumenCitation144 where there appears to be considerable crosstalk involving ATP between the epididymal cells and the maturating sperm. Since Panx3 is localized to the apical regions of the epididymis, it may be implicated in the release of ATP into the epididymal lumen. Panx1, on the other hand, localizes at the basal region of the epididymal epithelium, where it is expressed by principal and basal cells. Studies have shown that basal cells can reach the lumen of the epididymisCitation145,Citation146 and it has been suggested that they may be involved in scanning and sensing the luminal milieu and modulating the functions of adjacent cells.Citation147 Furthermore, there is evidence that basal cells release paracrine factors, such as prostaglandin E2, into the extracellular space, and activate corresponding receptors on principal cells.Citation148 This results in an increase in intracellular cAMP and causes the activation of plasma membrane channels, thereby modulating epithelial principal cell secretion.Citation149 Thus, the basal presence of Panx1 in the epididymis may regulate principal cell function and may also regulate the secretion of ATP into the basal extracellular space for functions as yet undefined ( and ).
Figure 5. Schematic diagram showing the immunolocalization of Panx1 in the seminiferous tubule. Panx1 was localized to the basal compartment of seminiferous epithelium, and suggestive of a reaction of the basal plasma membrane of Sertoli cells and in the myoid cells surrounding the seminiferous tubule. Panx3 was not present in the seminiferous trubule but was present in Leydig cells (not shown). Modified from a diagram of Clermont, The Sertoli cell, 1993, pp. xxi–xxv. Cache River Press.
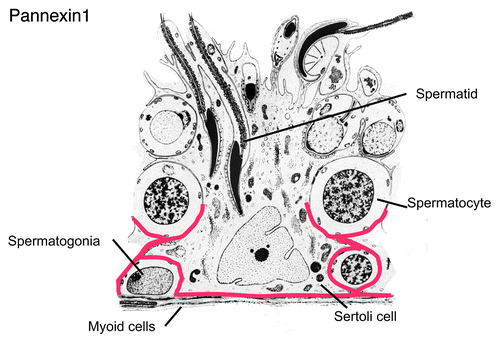
Figure 6. Schematic diagram showing the immunolocalization of Panx1 and Panx3 along the epididymis. Panx1 was localized to the base of the epithelium of the caput, corpus and cauda regions, where it stained the basal plasma membrane of principal cells as well as basal cells. The peritubular myoid cells were reactive especially in distal epididymal regions. Panx3 was localized along the apical plasma membrane of principal cells and along the entire length of the epididymis.
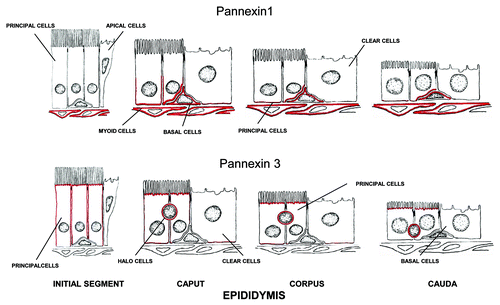
Conclusions and Perspectives
The role of connexins and intercellular gap junctions, particularly in the testis, have been shown to be critical for spermatogenesis and Sertoli cell differentiation and function. Asymmetric communication between Sertoli and developing germ cells via gap junctions likely represents a key modulator of germ cell maturation. The fact that changes in Cxs precede stages of development in some animal models supports the notion that gap junctions between Sertoli cells and germ cells may provide essential cues for stage-dependent maturation of germ cells. Furthermore, the influence of gap junctions on other cellular junctions, particularly the tight junctions which form the blood-testis barrier, indicates that gap junction intercellular communication may regulate multiple key aspects of testicular function. As such, the Cxs, which form the gap junctions, represent important but as yet unexplored therapeutic targets for modulating male reproduction. In the epididymis, data from our studies and those of others have demonstrated that different cell types of the epididymis communicate with one another. Based on the expression of different Cxs between different cell types, it is clear that this communication is selective between particular cell types, and this communication may be implicated in the differentiation of the epithelium as suggested by switches in Cx expression during postnatal cell differentiation in the epididymis. It is evident that critical interactions between apical epithelial cells, such as principal, clear, and basal cells occur, but the mechanisms and physiological significance of these interactions remain to be elucidated.
The presence of Cx hemichannels in the male reproductive remains unknown. However, initial studies show that Panxs are expressed in a compartmentalized fashion in both testis and epididymis. Studies regarding the intercellular communication and cell signaling via these structures may provide a better understanding of how gap junctions and these large cellular channels may work together in coordinating physiological functions in these tissues.
Acknowledgments
Mary Gregory and Julie Dufresne (INRS) are thanked for their helpful suggestions. Patrick Turmel, Dr. Évemie Dubé and Dr. Kenneth Finson are thanked for their research contributions; Drs. Louis Hermo (McGill), Charlie Smith (McGill) and Dale Laird (Western Ontario) are also thanked for their collaboration in many of the studies presented. Research funding from the Natural Sciences and Engineering Research Council of Canada is gratefully acknowledged.
References
- Kumar NM, Gilula NB. The gap junction communication channel. Cell 1996; 84:381 - 8; http://dx.doi.org/10.1016/S0092-8674(00)81282-9; PMID: 8608591
- Laird DW. The gap junction proteome and its relationship to disease. Trends Cell Biol 2010; 20:92 - 101; http://dx.doi.org/10.1016/j.tcb.2009.11.001; PMID: 19944606
- Goodenough DA, Goliger JA, Paul DL. Connexins, connexons, and intercellular communication. Annu Rev Biochem 1996; 65:475 - 502; http://dx.doi.org/10.1146/annurev.bi.65.070196.002355; PMID: 8811187
- Söhl G, Willecke K. An update on connexin genes and their nomenclature in mouse and man. Cell Commun Adhes 2003; 10:173 - 80; PMID: 14681012
- Meşe G, Richard G, White TW. Gap junctions: basic structure and function. J Invest Dermatol 2007; 127:2516 - 24; http://dx.doi.org/10.1038/sj.jid.5700770; PMID: 17934503
- Segretain D, Falk MM. Regulation of connexin biosynthesis, assembly, gap junction formation, and removal. Biochim Biophys Acta 2004; 1662:3-21.
- Winterhager E, Pielensticker N, Freyer J, Ghanem A, Schrickel JW, Kim JS, et al. Replacement of connexin43 by connexin26 in transgenic mice leads to dysfunctional reproductive organs and slowed ventricular conduction in the heart. BMC Dev Biol 2007; 7:26; http://dx.doi.org/10.1186/1471-213X-7-26; PMID: 17408477
- Goodenough DA, Paul DL. Gap junctions. Cold Spring Harb Perspect Biol 2009; 1:a002576; http://dx.doi.org/10.1101/cshperspect.a002576; PMID: 20066080
- Sosinsky GE, Nicholson BJ. Structural organization of gap junction channels. Biochim Biophys Acta 2005; 1711:99-125.
- Pelletier RM. The blood-testis barrier: the junctional permeability, the proteins and the lipids. Prog Histochem Cytochem 2011; 46:49 - 127; http://dx.doi.org/10.1016/j.proghi.2011.05.001; PMID: 21705043
- Hermo L, Pelletier RM, Cyr DG, Smith CE. Surfing the wave, cycle, life history, and genes/proteins expressed by testicular germ cells. Part 5: intercellular junctions and contacts between germs cells and Sertoli cells and their regulatory interactions, testicular cholesterol, and genes/proteins associated with more than one germ cell generation. Microsc Res Tech 2010; 73:409 - 94; PMID: 19941291
- Weider K, Bergmann M, Brehm R. Connexin 43: its regulatory role in testicular junction dynamics and spermatogenesis. Histol Histopathol 2011; 26:1343 - 52; PMID: 21870337
- Li MW, Mruk DD, Lee WM, Cheng CY. Connexin 43 is critical to maintain the homeostasis of the blood-testis barrier via its effects on tight junction reassembly. Proc Natl Acad Sci U S A 2010; 107:17998 - 8003; http://dx.doi.org/10.1073/pnas.1007047107; PMID: 20921394
- Risley MS, Tan IP, Roy C, Sáez JC. Cell-, age- and stage-dependent distribution of connexin43 gap junctions in testes. J Cell Sci 1992; 103:81 - 96; PMID: 1331136
- Tan IP, Roy C, Sáez JC, Sáez CG, Paul DL, Risley MS. Regulated assembly of connexin33 and connexin43 into rat Sertoli cell gap junctions. Biol Reprod 1996; 54:1300 - 10; http://dx.doi.org/10.1095/biolreprod54.6.1300; PMID: 8724358
- Juneja SC, Barr KJ, Enders GC, Kidder GM. Defects in the germ line and gonads of mice lacking connexin43. Biol Reprod 1999; 60:1263 - 70; http://dx.doi.org/10.1095/biolreprod60.5.1263; PMID: 10208994
- Roscoe WA, Barr KJ, Mhawi AA, Pomerantz DK, Kidder GM. Failure of spermatogenesis in mice lacking connexin43. Biol Reprod 2001; 65:829 - 38; http://dx.doi.org/10.1095/biolreprod65.3.829; PMID: 11514348
- Sridharan S, Simon L, Meling DD, Cyr DG, Gutstein DE, Fishman GI, et al. Proliferation of adult sertoli cells following conditional knockout of the Gap junctional protein GJA1 (connexin 43) in mice. Biol Reprod 2007; 76:804 - 12; http://dx.doi.org/10.1095/biolreprod.106.059212; PMID: 17229929
- Brehm R, Zeiler M, Rüttinger C, Herde K, Kibschull M, Winterhager E, et al. A sertoli cell-specific knockout of connexin43 prevents initiation of spermatogenesis. Am J Pathol 2007; 171:19 - 31; http://dx.doi.org/10.2353/ajpath.2007.061171; PMID: 17591950
- Plum A, Hallas G, Magin T, Dombrowski F, Hagendorff A, Schumacher B, et al. Unique and shared functions of different connexins in mice. Curr Biol 2000; 10:1083 - 91; http://dx.doi.org/10.1016/S0960-9822(00)00690-4; PMID: 10996788
- Francis RJ, Lo CW. Primordial germ cell deficiency in the connexin 43 knockout mouse arises from apoptosis associated with abnormal p53 activation. Development 2006; 133:3451 - 60; http://dx.doi.org/10.1242/dev.02506; PMID: 16887824
- Flenniken AM, Osborne LR, Anderson N, Ciliberti N, Fleming C, Gittens JE, et al. A Gja1 missense mutation in a mouse model of oculodentodigital dysplasia. Development 2005; 132:4375 - 86; http://dx.doi.org/10.1242/dev.02011; PMID: 16155213
- Paznekas WA, Karczeski B, Vermeer S, Lowry RB, Delatycki M, Laurence F, et al. GJA1 mutations, variants, and connexin 43 dysfunction as it relates to the oculodentodigital dysplasia phenotype. Hum Mutat 2009; 30:724 - 33; http://dx.doi.org/10.1002/humu.20958; PMID: 19338053
- Gregory M, Kahiri CN, Barr KJ, Smith CE, Hermo L, Cyr DG, et al. Male reproductive system defects and subfertility in a mutant mouse model of oculodentodigital dysplasia. Int J Androl 2011; 34:e630 - 41; http://dx.doi.org/10.1111/j.1365-2605.2011.01224.x; PMID: 22004529
- Holsberger DR, Cooke PS. Understanding the role of thyroid hormone in Sertoli cell development: a mechanistic hypothesis. Cell Tissue Res 2005; 322:133 - 40; http://dx.doi.org/10.1007/s00441-005-1082-z; PMID: 15856309
- Cooke PS, Meisami E. Early hypothyroidism in rats causes increased adult testis and reproductive organ size but does not change testosterone levels. Endocrinology 1991; 129:237 - 43; http://dx.doi.org/10.1210/endo-129-1-237; PMID: 2055186
- Cooke PS. Thyroid hormones and testis development: a model system for increasing testis growth and sperm production. Ann N Y Acad Sci 1991; 637:122 - 32; http://dx.doi.org/10.1111/j.1749-6632.1991.tb27305.x; PMID: 1785766
- St-Pierre N, Dufresne J, Rooney AA, Cyr DG. Neonatal hypothyroidism alters the localization of gap junctional protein connexin 43 in the testis and messenger RNA levels in the epididymis of the rat. Biol Reprod 2003; 68:1232 - 40; http://dx.doi.org/10.1095/biolreprod.102.010504; PMID: 12606457
- Gilleron J, Nebout M, Scarabelli L, Senegas-Balas F, Palmero S, Segretain D, et al. A potential novel mechanism involving connexin 43 gap junction for control of sertoli cell proliferation by thyroid hormones. J Cell Physiol 2006; 209:153 - 61; http://dx.doi.org/10.1002/jcp.20716; PMID: 16823880
- Sridharan S, Brehm R, Bergmann M, Cooke PS. Role of connexin 43 in Sertoli cells of testis. Ann N Y Acad Sci 2007; 1120:131 - 43; http://dx.doi.org/10.1196/annals.1411.004; PMID: 17905936
- Holsberger DR, Kiesewetter SE, Cooke PS. Regulation of neonatal Sertoli cell development by thyroid hormone receptor alpha1. Biol Reprod 2005; 73:396 - 403; http://dx.doi.org/10.1095/biolreprod.105.041426; PMID: 15858214
- de Montgolfier B, Dufresne J, Letourneau M, Nagler JJ, Fournier A, Audet C, et al. The expression of multiple connexins throughout spermatogenesis in the rainbow trout testis suggests a role for complex intercellular communication. Biol Reprod 2007; 76:2 - 8; http://dx.doi.org/10.1095/biolreprod.106.054288; PMID: 16971556
- de Montgolfier B, Audet C, Cyr DG. Regulation of the connexin 43 promoter in the brook trout testis: role of the thyroid hormones and cAMP. Gen Comp Endocrinol 2011; 170:110 - 8; http://dx.doi.org/10.1016/j.ygcen.2010.09.013; PMID: 20932836
- Stock A, Sies H. Thyroid hormone receptors bind to an element in the connexin43 promoter. Biol Chem 2000; 381:973 - 9; http://dx.doi.org/10.1515/BC.2000.120; PMID: 11076030
- de Montgolfier B, Fournier M, Audet C, Marcogliese DJ, Cyr DG. Influence of municipal effluents on the expression of connexins in the brook trout (Salvelinus fontinalis) testis. Aquat Toxicol 2008; 86:38 - 48; http://dx.doi.org/10.1016/j.aquatox.2007.09.013; PMID: 18006156
- Defamie N, Berthaut I, Mograbi B, Chevallier D, Dadoune JP, Fénichel P, et al. Impaired gap junction connexin43 in Sertoli cells of patients with secretory azoospermia: a marker of undifferentiated Sertoli cells. Lab Invest 2003; 83:449 - 56; PMID: 12649345
- Kotula-Balak M, Hejmej A, Sadowska J, Bilinska B. Connexin 43 expression in human and mouse testes with impaired spermatogenesis. Eur J Histochem 2007; 51:261 - 8; PMID: 18162455
- Brehm R, Rüttinger C, Fischer P, Gashaw I, Winterhager E, Kliesch S, et al. Transition from preinvasive carcinoma in situ to seminoma is accompanied by a reduction of connexin 43 expression in Sertoli cells and germ cells. Neoplasia 2006; 8:499 - 509; http://dx.doi.org/10.1593/neo.05847; PMID: 16820096
- Gilleron J, Fiorini C, Carette D, Avondet C, Falk MM, Segretain D, et al. Molecular reorganization of Cx43, Zo-1 and Src complexes during the endocytosis of gap junction plaques in response to a non-genomic carcinogen. J Cell Sci 2008; 121:4069 - 78; http://dx.doi.org/10.1242/jcs.033373; PMID: 19033388
- Gilleron J, Carette D, Fiorini C, Dompierre J, Macia E, Denizot JP, et al. The large GTPase dynamin2: a new player in connexin 43 gap junction endocytosis, recycling and degradation. Int J Biochem Cell Biol 2011; 43:1208 - 17; http://dx.doi.org/10.1016/j.biocel.2011.04.014; PMID: 21554976
- Cyr DG, Hermo L, Egenberger N, Mertineit C, Trasler JM, Laird DW. Cellular immunolocalization of occludin during embryonic and postnatal development of the mouse testis and epididymis. Endocrinology 1999; 140:3815 - 25; http://dx.doi.org/10.1210/en.140.8.3815; PMID: 10433243
- Batias C, Defamie N, Lablack A, Thepot D, Fenichel P, Segretain D, et al. Modified expression of testicular gap-junction connexin 43 during normal spermatogenic cycle and in altered spermatogenesis. Cell Tissue Res 1999; 298:113 - 21; http://dx.doi.org/10.1007/s004419900076; PMID: 10555545
- Carette D, Weider K, Gilleron J, Giese S, Dompierre J, Bergmann M, et al. Major involvement of connexin 43 in seminiferous epithelial junction dynamics and male fertility. Dev Biol 2010; 346:54 - 67; http://dx.doi.org/10.1016/j.ydbio.2010.07.014; PMID: 20655897
- Li MW, Mruk DD, Lee WM, Cheng CY. Connexin 43 and plakophilin-2 as a protein complex that regulates blood-testis barrier dynamics. Proc Natl Acad Sci U S A 2009; 106:10213 - 8; http://dx.doi.org/10.1073/pnas.0901700106; PMID: 19509333
- Chung SS, Lee WM, Cheng CY. Study on the formation of specialized inter-Sertoli cell junctions in vitro. J Cell Physiol 1999; 181:258 - 72; http://dx.doi.org/10.1002/(SICI)1097-4652(199911)181:2<258::AID-JCP8>3.0.CO;2-Q; PMID: 10497305
- Jongen WM, Fitzgerald DJ, Asamoto M, Piccoli C, Slaga TJ, Gros D, et al. Regulation of connexin 43-mediated gap junctional intercellular communication by Ca2+ in mouse epidermal cells is controlled by E-cadherin. J Cell Biol 1991; 114:545 - 55; http://dx.doi.org/10.1083/jcb.114.3.545; PMID: 1650371
- Wei CJ, Francis R, Xu X, Lo CW. Connexin43 associated with an N-cadherin-containing multiprotein complex is required for gap junction formation in NIH3T3 cells. J Biol Chem 2005; 280:19925 - 36; http://dx.doi.org/10.1074/jbc.M412921200; PMID: 15741167
- Meyer RA, Laird DW, Revel JP, Johnson RG. Inhibition of gap junction and adherens junction assembly by connexin and A-CAM antibodies. J Cell Biol 1992; 119:179 - 89; http://dx.doi.org/10.1083/jcb.119.1.179; PMID: 1326565
- Cyr DG, Blaschuk OW, Robaire B. Identification and developmental regulation of cadherin messenger ribonucleic acids in the rat testis. Endocrinology 1992; 131:139 - 45; http://dx.doi.org/10.1210/en.131.1.139; PMID: 1611992
- Yan HH, Mruk DD, Lee WM, Cheng CY. Cross-talk between tight and anchoring junctions-lesson from the testis. Adv Exp Med Biol 2008; 636:234 - 54; http://dx.doi.org/10.1007/978-0-387-09597-4_13; PMID: 19856171
- Cheng CY, Mruk DD. The Blood-Testis Barrier and Its Implications for Male Contraception. Pharmacol Rev 2011; In press PMID: 22039149
- Goossens S, van Roy F. Cadherin-mediated cell-cell adhesion in the testis. Front Biosci 2005; 10:398 - 419; http://dx.doi.org/10.2741/1537; PMID: 15574378
- Giepmans BN, Moolenaar WH. The gap junction protein connexin43 interacts with the second PDZ domain of the zona occludens-1 protein. Curr Biol 1998; 8:931 - 4; http://dx.doi.org/10.1016/S0960-9822(07)00375-2; PMID: 9707407
- Toyofuku T, Yabuki M, Otsu K, Kuzuya T, Hori M, Tada M. Direct association of the gap junction protein connexin-43 with ZO-1 in cardiac myocytes. J Biol Chem 1998; 273:12725 - 31; http://dx.doi.org/10.1074/jbc.273.21.12725; PMID: 9582296
- Singh D, Solan JL, Taffet SM, Javier R, Lampe PD. Connexin 43 interacts with zona occludens-1 and -2 proteins in a cell cycle stage-specific manner. J Biol Chem 2005; 280:30416 - 21; http://dx.doi.org/10.1074/jbc.M506799200; PMID: 15980428
- Kojima T, Sawada N, Chiba H, Kokai Y, Yamamoto M, Urban M, et al. Induction of tight junctions in human connexin 32 (hCx32)-transfected mouse hepatocytes: connexin 32 interacts with occludin. Biochem Biophys Res Commun 1999; 266:222 - 9; http://dx.doi.org/10.1006/bbrc.1999.1778; PMID: 10581193
- Kojima T, Kokai Y, Chiba H, Yamamoto M, Mochizuki Y, Sawada N. Cx32 but not Cx26 is associated with tight junctions in primary cultures of rat hepatocytes. Exp Cell Res 2001; 263:193 - 201; http://dx.doi.org/10.1006/excr.2000.5103; PMID: 11161718
- Nagasawa K, Chiba H, Fujita H, Kojima T, Saito T, Endo T, et al. Possible involvement of gap junctions in the barrier function of tight junctions of brain and lung endothelial cells. J Cell Physiol 2006; 208:123 - 32; http://dx.doi.org/10.1002/jcp.20647; PMID: 16547974
- Murata M, Kojima T, Yamamoto T, Go M, Takano K, Chiba H, et al. Tight junction protein MAGI-1 is up-regulated by transfection with connexin 32 in an immortalized mouse hepatic cell line: cDNA microarray analysis. Cell Tissue Res 2005; 319:341 - 7; http://dx.doi.org/10.1007/s00441-004-1017-0; PMID: 15558322
- Morita H, Katsuno T, Hoshimoto A, Hirano N, Saito Y, Suzuki Y. Connexin 26-mediated gap junctional intercellular communication suppresses paracellular permeability of human intestinal epithelial cell monolayers. Exp Cell Res 2004; 298:1 - 8; http://dx.doi.org/10.1016/j.yexcr.2004.03.046; PMID: 15242756
- Pérez-Armendariz EM, Romano MC, Luna J, Miranda C, Bennett MV, Moreno AP. Characterization of gap junctions between pairs of Leydig cells from mouse testis. Am J Physiol 1994; 267:C570 - 80; PMID: 7521131
- Varanda WA, de Carvalho AC. Intercellular communication between mouse Leydig cells. Am J Physiol 1994; 267:C563 - 9; PMID: 8074190
- Kahiri CN, Khalil MW, Tekpetey F, Kidder GM. Leydig cell function in mice lacking connexin43. Reproduction 2006; 132:607 - 16; http://dx.doi.org/10.1530/rep.1.01234; PMID: 17008472
- Orgebin-Crist MC. Maturation of spermatozoa in the rabbit epididymis: delayed fertilization in does inseminated with epididymal spermatozoa. J Reprod Fertil 1968; 16:29 - 33; http://dx.doi.org/10.1530/jrf.0.0160029; PMID: 5691162
- Bedford JM. Changes in fine structure of the rabbit sperm head during passage through the epididymis. J Anat 1965; 99:891 - 906; PMID: 5867174
- Cyr DG, Finnson K, Dufresne J, Gregory M. Cellular interactions and the blood-epididymal barrier. In: Robaire B, Hinton BT, eds. The Epididymis: from Molecules to Clinical Practice. New York: Plenum Press, 2002:103-18.
- Dubé É, Cyr DG. The Blood-Epididymis Barrier. In: Cheng CY, ed. Blood-Tissue Barriers. Cambridge, MA: Landes Bioscience, 2011:(in press).
- Robaire B, Hinton BT, Orgebin-Crist MC. The epididymis. In: JD N, ed. Knobil and Neill's Physiology of Reproduction. New York: Elsevier, 2006:1071-148.
- Robaire BHL. Efferent ducts, epididymis, and vas deferens: Structure, functions, and their regulation. New York: Raven Press Limited, 1988.
- Wong PDY, Gong XD, Leung GPH, Cheuk BLY. Formation of the epididymal fluid environment. In: Robaire B, Hinton BT, eds. The Epididymis: from Molecules to Clinical Practice. New York: Plenum Press, 2002:119-30.
- Cyr DG, Robaire B, Hermo L. Structure and turnover of junctional complexes between principal cells of the rat epididymis. Microsc Res Tech 1995; 30:54 - 66; http://dx.doi.org/10.1002/jemt.1070300105; PMID: 7711320
- Gregory M, Dufresne J, Hermo L, Cyr D. Claudin-1 is not restricted to tight junctions in the rat epididymis. Endocrinology 2001; 142:854 - 63; http://dx.doi.org/10.1210/en.142.2.854; PMID: 11159859
- Gregory M, Cyr DG. Identification of multiple claudins in the rat epididymis. Mol Reprod Dev 2006; 73:580 - 8; http://dx.doi.org/10.1002/mrd.20467; PMID: 16489631
- Dubé E, Chan PT, Hermo L, Cyr DG. Gene expression profiling and its relevance to the blood-epididymal barrier in the human epididymis. Biol Reprod 2007; 76:1034 - 44; http://dx.doi.org/10.1095/biolreprod.106.059246; PMID: 17287494
- Anderson JM, Van Itallie CM. Physiology and function of the tight junction. Cold Spring Harb Perspect Biol 2009; 1:a002584; http://dx.doi.org/10.1101/cshperspect.a002584; PMID: 20066090
- Friend DS, Gilula NB. Variations in tight and gap junctions in mammalian tissues. J Cell Biol 1972; 53:758 - 76; http://dx.doi.org/10.1083/jcb.53.3.758; PMID: 4337577
- Pelletier RM. Freeze-fracture study of cell junctions in the epididymis and vas deferens of a seasonal breeder: the mink (Mustela vison). Microsc Res Tech 1995; 30:37 - 53; http://dx.doi.org/10.1002/jemt.1070300104; PMID: 7711319
- Cyr DG, Hermo L, Laird DW. Immunocytochemical localization and regulation of connexin43 in the adult rat epididymis. Endocrinology 1996; 137:1474 - 84; http://dx.doi.org/10.1210/en.137.4.1474; PMID: 8625926
- Alkafafy M, Elnasharty M, Sayed-Ahmed A, Abdrabou M. Immunohistochemical studies of the epididymal duct in Egyptian water buffalo (Bubalus bubalis). Acta Histochem 2011; 113:96 - 102; http://dx.doi.org/10.1016/j.acthis.2009.08.004; PMID: 19836061
- Hejmej A, Kotula-Balak M, Sadowska J, Bilińska B. Expression of connexin 43 protein in testes, epididymides and prostates of stallions. Equine Vet J 2007; 39:122 - 7; http://dx.doi.org/10.2746/042516407X169393; PMID: 17378440
- Dufresne J, Finnson KW, Gregory M, Cyr DG. Expression of multiple connexins in the rat epididymis indicates a complex regulation of gap junctional communication. Am J Physiol Cell Physiol 2003; 284:C33 - 43; PMID: 12388089
- Friend DS, Gilula NB. A distinctive cell contact in the rat adrenal cortex. J Cell Biol 1972; 53:148 - 63; http://dx.doi.org/10.1083/jcb.53.1.148; PMID: 5013594
- Sun EL, Flickinger CJ. Development of cell types and of regional differences in the postnatal rat epididymis. Am J Anat 1979; 154:27 - 55; http://dx.doi.org/10.1002/aja.1001540104; PMID: 760488
- Hermo L, Barin K, Robaire B. Structural differentiation of the epithelial cells of the testicular excurrent duct system of rats during postnatal development. Anat Rec 1992; 233:205 - 28; http://dx.doi.org/10.1002/ar.1092330205; PMID: 1605386
- Brissette JL, Kumar NM, Gilula NB, Hall JE, Dotto GP. Switch in gap junction protein expression is associated with selective changes in junctional permeability during keratinocyte differentiation. Proc Natl Acad Sci U S A 1994; 91:6453 - 7; http://dx.doi.org/10.1073/pnas.91.14.6453; PMID: 8022804
- Araki Y, Suzuki K, Matusik RJ, Obinata M, Orgebin-Crist MC. Immortalized epididymal cell lines from transgenic mice overexpressing temperature-sensitive simian virus 40 large T-antigen gene. J Androl 2002; 23:854 - 69; PMID: 12399533
- Kirchhoff C, Araki Y, Huhtaniemi I, Matusik RJ, Osterhoff C, Poutanen M, et al. Immortalization by large T-antigen of the adult epididymal duct epithelium. Mol Cell Endocrinol 2004; 216:83 - 94; http://dx.doi.org/10.1016/j.mce.2003.10.073; PMID: 15109748
- Sipilä P, Shariatmadari R, Huhtaniemi IT, Poutanen M. Immortalization of epididymal epithelium in transgenic mice expressing simian virus 40 T antigen: characterization of cell lines and regulation of the polyoma enhancer activator 3. Endocrinology 2004; 145:437 - 46; http://dx.doi.org/10.1210/en.2003-0831; PMID: 14527890
- Telgmann R, Brosens JJ, Käppler-Hanno K, Ivell R, Kirchhoff C. Epididymal epithelium immortalized by simian virus 40 large T antigen: a model to study epididymal gene expression. Mol Hum Reprod 2001; 7:935 - 45; http://dx.doi.org/10.1093/molehr/7.10.935; PMID: 11574662
- Dufresne J, St-Pierre N, Viger RS, Hermo L, Cyr DG. Characterization of a novel rat epididymal cell line to study epididymal function. Endocrinology 2005; 146:4710 - 20; http://dx.doi.org/10.1210/en.2004-1634; PMID: 16099865
- Dube E, Hermo L, Chan PT, Cyr DG. Alterations in the human blood-epididymis barrier in obstructive azoospermia and the development of novel epididymal cell lines from infertile men. Biol Reprod 2010; 83:584 - 96; http://dx.doi.org/10.1095/biolreprod.110.084459; PMID: 20505168
- Dubé E, Dufresne J, Chan PT, Hermo L, Cyr DG. Assessing the role of claudins in maintaining the integrity of epididymal tight junctions using novel human epididymal cell lines. Biol Reprod 2010; 82:1119 - 28; http://dx.doi.org/10.1095/biolreprod.109.083196; PMID: 20164436
- Lydka M, Kopera-Sobota I, Kotula-Balak M, Chojnacka K, Zak D, Bilinska B. Morphological and functional alterations in adult boar epididymis: Effects of prenatal and postnatal administration of flutamide. Acta Vet Scand 2011; 53:12; http://dx.doi.org/10.1186/1751-0147-53-12; PMID: 21342526
- Hamzeh M, Robaire B. Identification of early response genes and pathway activated by androgens in the initial segment and caput regions of the regressed rat epididymis. Endocrinology 2010; 151:4504 - 14; http://dx.doi.org/10.1210/en.2010-0023; PMID: 20660069
- Hardy MP, Sharma RS, Arambepola NK, Sottas CM, Russell LD, Bunick D, et al. Increased proliferation of Leydig cells induced by neonatal hypothyroidism in the rat. J Androl 1996; 17:231 - 8; PMID: 8792213
- van Haaster LH, de Jong FH, Docter R, de Rooij DG. High neonatal triiodothyronine levels reduce the period of Sertoli cell proliferation and accelerate tubular lumen formation in the rat testis, and increase serum inhibin levels. Endocrinology 1993; 133:755 - 60; http://dx.doi.org/10.1210/en.133.2.755; PMID: 8344214
- Van Haaster LH, De Jong FH, Docter R, De Rooij DG. The effect of hypothyroidism on Sertoli cell proliferation and differentiation and hormone levels during testicular development in the rat. Endocrinology 1992; 131:1574 - 6; http://dx.doi.org/10.1210/en.131.3.1574; PMID: 1505485
- Teerds KJ, de Rooij DG, de Jong FH, van Haaster LH. Development of the adult-type Leydig cell population in the rat is affected by neonatal thyroid hormone levels. Biol Reprod 1998; 59:344 - 50; http://dx.doi.org/10.1095/biolreprod59.2.344; PMID: 9687306
- Churko JM, Shao Q, Gong XQ, Swoboda KJ, Bai D, Sampson J, et al. Human dermal fibroblasts derived from oculodentodigital dysplasia patients suggest that patients may have wound-healing defects. Hum Mutat 2011; 32:456 - 66; http://dx.doi.org/10.1002/humu.21472; PMID: 21305658
- Thompson RJ, Macvicar BA. Connexin and pannexin hemichannels of neurons and astrocytes. Channels (Austin) 2008; 2:81 - 6; http://dx.doi.org/10.4161/chan.2.2.6003; PMID: 18849665
- Spray DC, Ye ZC, Ransom BR. Functional connexin “hemichannels”: a critical appraisal. Glia 2006; 54:758 - 73; http://dx.doi.org/10.1002/glia.20429; PMID: 17006904
- Willecke K, Eiberger J, Degen J, Eckardt D, Romualdi A, Güldenagel M, et al. Structural and functional diversity of connexin genes in the mouse and human genome. Biol Chem 2002; 383:725 - 37; http://dx.doi.org/10.1515/BC.2002.076; PMID: 12108537
- Sonntag S, Söhl G, Dobrowolski R, Zhang J, Theis M, Winterhager E, et al. Mouse lens connexin23 (Gje1) does not form functional gap junction channels but causes enhanced ATP release from HeLa cells. Eur J Cell Biol 2009; 88:65 - 77; http://dx.doi.org/10.1016/j.ejcb.2008.08.004; PMID: 18849090
- Jiang JX, Cherian PP. Hemichannels formed by connexin 43 play an important role in the release of prostaglandin E(2) by osteocytes in response to mechanical strain. Cell Commun Adhes 2003; 10:259 - 64; PMID: 14681026
- Burra S, Jiang JX. Regulation of cellular function by connexin hemichannels. Int J Biochem Mol Biol 2011; 2:119 - 28; PMID: 21968837
- Scemes E, Suadicani SO, Dahl G, Spray DC. Connexin and pannexin mediated cell-cell communication. Neuron Glia Biol 2007; 3:199 - 208; http://dx.doi.org/10.1017/S1740925X08000069; PMID: 18634611
- Srinivas M, Calderon DP, Kronengold J, Verselis VK. Regulation of connexin hemichannels by monovalent cations. J Gen Physiol 2006; 127:67 - 75; http://dx.doi.org/10.1085/jgp.200509397; PMID: 16380444
- Sipos A, Vargas SL, Toma I, Hanner F, Willecke K, Peti-Peterdi J. Connexin 30 deficiency impairs renal tubular ATP release and pressure natriuresis. J Am Soc Nephrol 2009; 20:1724 - 32; http://dx.doi.org/10.1681/ASN.2008101099; PMID: 19478095
- Hanner F, Schnichels M, Zheng-Fischhöfer Q, Yang LE, Toma I, Willecke K, et al. Connexin 30.3 is expressed in the kidney but not regulated by dietary salt or high blood pressure. Cell Commun Adhes 2008; 15:219 - 30; http://dx.doi.org/10.1080/15419060802013836; PMID: 18649192
- Contreras JE, Sáez JC, Bukauskas FF, Bennett MV. Gating and regulation of connexin 43 (Cx43) hemichannels. Proc Natl Acad Sci U S A 2003; 100:11388 - 93; http://dx.doi.org/10.1073/pnas.1434298100; PMID: 13130072
- Leung GP, Cheung KH, Leung CT, Tsang MW, Wong PY. Regulation of epididymal principal cell functions by basal cells: role of transient receptor potential (Trp) proteins and cyclooxygenase-1 (COX-1). Mol Cell Endocrinol 2004; 216:5 - 13; http://dx.doi.org/10.1016/j.mce.2003.10.077; PMID: 15109739
- Contreras JE, Sánchez HA, Véliz LP, Bukauskas FF, Bennett MV, Sáez JC. Role of connexin-based gap junction channels and hemichannels in ischemia-induced cell death in nervous tissue. Brain Res Brain Res Rev 2004; 47:290 - 303; http://dx.doi.org/10.1016/j.brainresrev.2004.08.002; PMID: 15572178
- Schock SC, Leblanc D, Hakim AM, Thompson CS. ATP release by way of connexin 36 hemichannels mediates ischemic tolerance in vitro. Biochem Biophys Res Commun 2008; 368:138 - 44; http://dx.doi.org/10.1016/j.bbrc.2008.01.054; PMID: 18211823
- John SA, Kondo R, Wang SY, Goldhaber JI, Weiss JN. Connexin-43 hemichannels opened by metabolic inhibition. J Biol Chem 1999; 274:236 - 40; http://dx.doi.org/10.1074/jbc.274.1.236; PMID: 9867835
- Contreras JE, Sáez JC, Bukauskas FF, Bennett MV. Functioning of cx43 hemichannels demonstrated by single channel properties. Cell Commun Adhes 2003; 10:245 - 9; PMID: 14681024
- Saez JC, Retamal MA, Basilio D, Bukauskas FF, Bennett MV. Connexin-based gap junction hemichannels: gating mechanisms. Biochim Biophys Acta 2005; 1711:215-24.
- Locovei S, Wang J, Dahl G. Activation of pannexin 1 channels by ATP through P2Y receptors and by cytoplasmic calcium. FEBS Lett 2006; 580:239 - 44; http://dx.doi.org/10.1016/j.febslet.2005.12.004; PMID: 16364313
- Locovei S, Bao L, Dahl G. Pannexin 1 in erythrocytes: function without a gap. Proc Natl Acad Sci U S A 2006; 103:7655 - 9; http://dx.doi.org/10.1073/pnas.0601037103; PMID: 16682648
- Scemes E. Nature of plasmalemmal functional “hemichannels”. Biochim Biophys Acta 2011; In press PMID: 21703226
- Boassa D, Qiu F, Dahl G, Sosinsky G. Trafficking dynamics of glycosylated pannexin 1 proteins. Cell Commun Adhes 2008; 15:119 - 32; http://dx.doi.org/10.1080/15419060802013885; PMID: 18649184
- Penuela S, Bhalla R, Gong XQ, Cowan KN, Celetti SJ, Cowan BJ, et al. Pannexin 1 and pannexin 3 are glycoproteins that exhibit many distinct characteristics from the connexin family of gap junction proteins. J Cell Sci 2007; 120:3772 - 83; http://dx.doi.org/10.1242/jcs.009514; PMID: 17925379
- Yen MR, Saier MH Jr.. Gap junctional proteins of animals: the innexin/pannexin superfamily. Prog Biophys Mol Biol 2007; 94:5 - 14; http://dx.doi.org/10.1016/j.pbiomolbio.2007.03.006; PMID: 17507077
- Shestopalov VI, Panchin Y. Pannexins and gap junction protein diversity. Cell Mol Life Sci 2008; 65:376 - 94; http://dx.doi.org/10.1007/s00018-007-7200-1; PMID: 17982731
- D’hondt C, Ponsaerts R, De Smedt H, Bultynck G, Himpens B. Pannexins, distant relatives of the connexin family with specific cellular functions?. Bioessays 2009; 31:953 - 74; http://dx.doi.org/10.1002/bies.200800236; PMID: 19644918
- Bao L, Samuels S, Locovei S, Macagno ER, Muller KJ, Dahl G. Innexins form two types of channels. FEBS Lett 2007; 581:5703 - 8; http://dx.doi.org/10.1016/j.febslet.2007.11.030; PMID: 18035059
- Baranova A, Ivanov D, Petrash N, Pestova A, Skoblov M, Kelmanson I, et al. The mammalian pannexin family is homologous to the invertebrate innexin gap junction proteins. Genomics 2004; 83:706 - 16; http://dx.doi.org/10.1016/j.ygeno.2003.09.025; PMID: 15028292
- Bruzzone R, Barbe MT, Jakob NJ, Monyer H. Pharmacological properties of homomeric and heteromeric pannexin hemichannels expressed in Xenopus oocytes. J Neurochem 2005; 92:1033 - 43; http://dx.doi.org/10.1111/j.1471-4159.2004.02947.x; PMID: 15715654
- Sosinsky GE, Boassa D, Dermietzel R, Duffy HS, Laird DW, MacVicar B, et al. Pannexin channels are not gap junction hemichannels. Channels (Austin) 2011; 5:193 - 7; http://dx.doi.org/10.4161/chan.5.3.15765; PMID: 21532340
- Barbe MT, Monyer H, Bruzzone R. Cell-cell communication beyond connexins: the pannexin channels. Physiology (Bethesda) 2006; 21:103 - 14; http://dx.doi.org/10.1152/physiol.00048.2005; PMID: 16565476
- Penuela S, Bhalla R, Nag K, Laird DW. Glycosylation regulates pannexin intermixing and cellular localization. Mol Biol Cell 2009; 20:4313 - 23; http://dx.doi.org/10.1091/mbc.E09-01-0067; PMID: 19692571
- Bruzzone R, Hormuzdi SG, Barbe MT, Herb A, Monyer H. Pannexins, a family of gap junction proteins expressed in brain. Proc Natl Acad Sci U S A 2003; 100:13644 - 9; http://dx.doi.org/10.1073/pnas.2233464100; PMID: 14597722
- Vanden Abeele F, Bidaux G, Gordienko D, Beck B, Panchin YV, Baranova AV, et al. Functional implications of calcium permeability of the channel formed by pannexin 1. J Cell Biol 2006; 174:535 - 46; http://dx.doi.org/10.1083/jcb.200601115; PMID: 16908669
- Huang YJ, Maruyama Y, Dvoryanchikov G, Pereira E, Chaudhari N, Roper SD. The role of pannexin 1 hemichannels in ATP release and cell-cell communication in mouse taste buds. Proc Natl Acad Sci U S A 2007; 104:6436 - 41; http://dx.doi.org/10.1073/pnas.0611280104; PMID: 17389364
- Bargiotas P, Monyer H, Schwaninger M. Hemichannels in cerebral ischemia. Curr Mol Med 2009; 9:186 - 94; http://dx.doi.org/10.2174/156652409787581646; PMID: 19275626
- D’hondt C, Ponsaerts R, De Smedt H, Vinken M, De Vuyst E, De Bock M, et al. Pannexin channels in ATP release and beyond: an unexpected rendezvous at the endoplasmic reticulum. Cell Signal 2011; 23:305 - 16; http://dx.doi.org/10.1016/j.cellsig.2010.07.018; PMID: 20688156
- Turmel P, Dufresne J, Hermo L, Smith CE, Penuela S, Laird DW, et al. Characterization of pannexin1 and pannexin3 and their regulation by androgens in the male reproductive tract of the adult rat. Mol Reprod Dev 2011; 78:124 - 38; http://dx.doi.org/10.1002/mrd.21280; PMID: 21337450
- Ma W, Hui H, Pelegrin P, Surprenant A. Pharmacological characterization of pannexin-1 currents expressed in mammalian cells. J Pharmacol Exp Ther 2009; 328:409 - 18; http://dx.doi.org/10.1124/jpet.108.146365; PMID: 19023039
- Hess RA. The efferent ductules: Structure and functions. In: Robaire B, Hinton BT, eds. The Epididymis: From Molecules to Clinical Practice. New Yourk: Plenum Press, 2002:49-80.
- Pointis G, Segretain D. Role of connexin-based gap junction channels in testis. Trends Endocrinol Metab 2005; 16:300 - 6; http://dx.doi.org/10.1016/j.tem.2005.07.001; PMID: 16054834
- Dvoriantchikova G, Ivanov D, Panchin Y, Shestopalov VI. Expression of pannexin family of proteins in the retina. FEBS Lett 2006; 580:2178 - 82; http://dx.doi.org/10.1016/j.febslet.2006.03.026; PMID: 16616526
- Cyr DG, Robaire B. Regulation of sulfated glycoprotein-2 (clusterin) messenger ribonucleic acid in the rat epididymis. Endocrinology 1992; 130:2160 - 6; http://dx.doi.org/10.1210/en.130.4.2160; PMID: 1547732
- Robaire B, Viger RS. Regulation of epididymal epithelial cell functions. Biol Reprod 1995; 52:226 - 36; http://dx.doi.org/10.1095/biolreprod52.2.226; PMID: 7711192
- Robaire B, Hamzeh M. Androgen action in the epididymis. J Androl 2011; 32:592 - 9; http://dx.doi.org/10.2164/jandrol.111.014266; PMID: 21764895
- Zhou WL, Zuo WL, Ruan YC, Wang Z, Du JY, Xiong Y, et al. The role of extracellular ATP in the male reproductive tract. Sheng Li Xue Bao 2007; 59:487 - 94; PMID: 17700968
- Veri JP, Hermo L, Robaire B. Immunocytochemical localization of the Yf subunit of glutathione S-transferase P shows regional variation in the staining of epithelial cells of the testis, efferent ducts, and epididymis of the male rat. J Androl 1993; 14:23 - 44; PMID: 8473235
- Shum WW, Da Silva N, McKee M, Smith PJ, Brown D, Breton S. Transepithelial projections from basal cells are luminal sensors in pseudostratified epithelia. Cell 2008; 135:1108 - 17; http://dx.doi.org/10.1016/j.cell.2008.10.020; PMID: 19070580
- Shum WW, Ruan YC, Da Silva N, Breton S. Establishment of cell-cell cross talk in the epididymis: control of luminal acidification. J Androl 2011; 32:576 - 86; http://dx.doi.org/10.2164/jandrol.111.012971; PMID: 21441423
- Wong PY, Chan HC, Leung PS, Chung YW, Wong YL, Lee WM, et al. Regulation of anion secretion by cyclo-oxygenase and prostanoids in cultured epididymal epithelia from the rat. J Physiol 1999; 514:809 - 20; http://dx.doi.org/10.1111/j.1469-7793.1999.809ad.x; PMID: 9882752
- Cheung KH, Leung GP, Leung MC, Shum WW, Zhou WL, Wong PY. Cell-cell interaction underlies formation of fluid in the male reproductive tract of the rat. J Gen Physiol 2005; 125:443 - 54; http://dx.doi.org/10.1085/jgp.200409205; PMID: 15851503