Abstract
Crohn’s disease (CD) and ulcerative colitis (UC), collectively called inflammatory bowel disease (IBD), are immune-mediated conditions characterized by a chronic inflammation of the gut. Their precise etiology is unknown, although an increased intestinal permeability has been shown to play a central role in the pathogenesis of IBD. The intestinal epithelium provides the largest interface between the external environment and the host, and is thus a crucial regulation site of innate and adaptive immunity. Zonulin is one of the few known physiological mediators of paracellular intestinal permeability. It was found upregulated in different immune diseases like Celiac disease and Type 1 Diabetes (T1D). Recently, human zonulin was identified as prehaptoglobin-2 (pre-HP2) which before only had been regarded as the inactive precursor for HP2. Haptoglobin (HP) is a hemoglobin-binding protein with immunomodulatory properties. Its gene harbors a common polymorphism with 2 different alleles: HP1 and HP2. Allele HP2 and genotype HP22 has been shown to be overrepresented in different immune diseases like Rheumatoid Arthritis (RA), Systemic Lupus Erythematosus (SLE) and T1D, and has also been found to be more frequent in patients with IBD (UC and CD) than in healthy controls.
In order to get some clues about the mechanism of action of HP(2) in IBD pathogenesis, we here review the current state of knowledge about zonulin and haptoglobin structure and function, and their plausible role in immune mediated diseases with an emphasis on IBD.
Introduction: role of barrier integrity/intestinal permeability in IBD etiology
The single cell layer of the intestinal epithelium forms the largest barrier between the external environment and the host. The intestinal mucosa is in continuous contact with microorganisms and dietary antigens, creating a crucial site for regulation of both innate and adaptive immunity.Citation1 Since the plasma membrane is virtually impermeable to hydrophilic solutes in the absence of specific transporters, passive flux of small water-soluble solutes and ions occurs mainly via the paracellular route, which is controlled by the apical junctional complex.Citation2,Citation3 Tight junctions, the principal part of the junctional complex, are composed of transmembrane proteins of which occludin and the claudins are the best characterized, and peripheral membrane proteins like zonula-occludens 1 (ZO-1) which link the transmembrane tight junction-related proteins to the perijunctional cytoskeleton.Citation4-Citation6 Initially regarded as static intercellular sealing structures, tight junctions are increasingly recognized as flexible gatekeepers regulating the flow across the epithelium.Citation7
An abnormal barrier function, as reflected by increased intestinal permeability, has been implicated in a variety of conditions like Celiac disease and Type 1 Diabetes.Citation8-Citation10 An increased intestinal permeability has also been shown to play a central role in the pathogenesis of Crohn’s disease (CD) and ulcerative colitis (UC), jointly called inflammatory bowel disease (IBD).Citation11-Citation16 IBD is a chronic, relapsing, inflammatory disorder of the gastrointestinal tract. It is a complex and polygenic disease that affects an increasing number of people in Western countries and more recently also in Asia.Citation17,Citation18 The current accepted hypothesis for its pathogenesis is that a dysregulated immune response occurs to the commensal microbiota in the gut in a genetically susceptible subject.Citation19,Citation20 It is thus plausible that an impaired intestinal epithelial barrier allows for the interaction between an unprocessed antigen and the mucosal immune system hereby leading to an abnormal immune response. It is however unclear whether the barrier dysfunction observed in IBD patients is a primary event or a consequence of the mucosal inflammation. Several studies suggest that an epithelial barrier dysfunction may have a primary role in triggering disease: IBD animal models have shown that increased intestinal permeability is a very early event in disease pathogenesis and precedes the development of disease.Citation21 Also, a persisting increased intestinal permeability was shown to be a risk factor for relapse in CD.Citation16 Finally, a proportion of unaffected first-degree relatives of IBD patients show an increased intestinal permeability.Citation12,Citation13 First-degree relatives of patients with CD have the highest risk to also develop the disease. Although it is yet unclear if at-risk individuals with an abnormal intestinal permeability indeed go on developing the disease, there is an interesting case report of a young symptom-free girl who showed an abnormal intestinal permeability and whose brother had CD.Citation22 She had a negative workup thus showing no evidence of CD, but then went on to develop CD 7 y later. This suggests a link between genetic susceptibility for the disease and abnormal intestinal permeability, and the subsequent development of the disease.
Zonulin and Haptoglobin
Structure and function of zonulin
In contrast to increasingly detailed knowledge about the structure of the tight junction, surprisingly little is known about the regulation of its permeability.
In a series of seminal papers, zonulin was identified as an important physiological regulator of paracellular intestinal permeability. This work started more than 20 y ago when Fasano and colleagues described zonula occludens toxin (Zot) as a second enterotoxin in Vibrio cholerae.Citation23,Citation24 Zot was responsible for mild diarrhea in volunteers who were vaccinated with an attenuated V. cholerae strain in which the A subunit of the conventional cholera-toxin, encoded by ctxA, was eliminated. The 45kDa Zot toxin is localized in the outer membrane of the bacterium and a 12kDa C-terminal fragment is secreted after cleavage at amino acid 288.Citation25 The biologically active sequence was localized to the first six amino acids of the newly-formed NH2-terminal part.Citation26 When the supernatans of the attenuated cholera strain or purified Zot was applied on rabbit ileum in Ussing chambers, a reversible decrease in transepithelial resistance was observed.Citation24,Citation27 Similarly, administration of purified Zot during isolated loop perfusion in rabbits reversibly shifted intestinal fluid handling from absorption to secretion and increased the passage of large molecules like insulin, cyclosporine A, immunoglobulins and 4kDa PEG in the small intestine but not in the colon.Citation27-Citation29 When co-administered with Zot, oral insulin effectively lowered blood insulin in diabetic BB/Wor rats with kinetics similar to subcutaneous administration suggesting increased paracellular passage.Citation29 The binding of the Zot in the ileum and jejunum showed a decreasing gradient from the villus tip to the crypt and was absent in the colon.Citation27,Citation30
Since Zot acted in a non-cytotoxic and reversible manner, it was serendipitously hypothesized that a eukaryotic analog of Zot may be operative in the (patho)physiological regulation of the tight junction. Using specific anti-Zot antibodies, a single protein with a molecular weight of 47kDa was detected in human intestinal tissue.Citation9,Citation31 This human intestinal Zot analog was named zonulin due to its actions on the zonula occludens or tight junction. Similar to its prokaryotic analog, affinity-purified zonulin reversibly lowered the transepithelial resistance in the small intestine and not in the colon of non-human primates.Citation31 Moreover, the zonulin receptor was shown to be present at the apical surface only, since basolateral administration failed to induce alterations in intestinal permeability.
Comparison of the N-terminal end of zonulin and the active fragment of ZotCitation26 revealed a conserved common motif.Citation25,Citation31 A synthetic octapeptide (GGVLVQPG), named FZI/0,Citation26,Citation32 AT1001Citation33,Citation34 and recently larazotide,Citation35-Citation37 corresponding to the amino acids 8–15 of this fragment, did not affect permeability, measured as transepithelial electrical resistance of rabbit ileum in Ussing chambers.Citation26 However, pretreatment with AT1001 offered a significant protection against the effect of subsequent treatment with purified Zot or zonulin.Citation26,Citation31 Conversely, a synthetic hexapeptide (FCIGRL), named AT1002, comprising the first six amino-acids of the active Zot fragment (aa 288–293), reproduced the effect of Zot and zonulin on paracellular permeability by increasing in vivo (quantified by a lactulose/rhamnose urinary excretion test after gavage of AT1002) and in vitro (transepithelial electrical resistance) permeability of murine and rat small intestine.Citation38,Citation39 AT1002 is currently being studied for applications to enhance oral drug absorption.Citation28,Citation40,Citation41
So far, gluten and bacteria (commensals and pathogenic) have been identified as triggers for small intestinal, luminal zonulin release from intact intestinal tissue and epithelial cell monolayers.Citation42,Citation43 The effect of the bacterial strains on intestinal permeability correlated with luminal secretion of zonulin and could be blocked by AT1001 pretreatment.Citation42 The increased paracellular permeability leads to increased intraluminal water secretion, possibly as part of the host innate immune response preventing bacterial colonization of the small intestine.Citation27,Citation42
The molecular mechanism through which zonulin enhances small intestinal permeability is still incompletely elucidated. In the initial report of Zot, a decrease in the number of freeze fracture strands and the number of strand intersections was shown, indicating a disassembly of the tight junction.Citation24 At the molecular level, Zot was demonstrated to induce polymerization of G-actin to F-actin in a protein kinase C α (PKCα) dependent manner, suggesting that contraction of the cytoskeleton is involved in the permeability-enhancing effect.Citation30 Follow-up studies demonstrated that Zot, zonulin and AT1002 operate through activation of the proteinase-activated receptor 2 (PAR2) and subsequent transactivation of the epidermal growth factor receptor (EGFR).Citation38,Citation44 PAR2 is activated by proteolytic cleavage of the N-terminus of this G-protein coupled receptor, uncovering a tethered ligand which activates the receptor. The sequence of the active fragment of Zot (FCIGRL) is reminiscent of the activating peptide (SLIGRL) for PAR2, which has been shown to increase intestinal permeability through activation of PAR2 without receptor cleavage.Citation45,Citation46 Recombinant zonulin and AT1002 did not alter permeability in PAR2-knockout mice or in Caco2-cells in which PAR2 was silenced.Citation38,Citation47 Moreover, phosphorylation, i.e., activation, of EGFR was reduced in Caco2 cells with silenced PAR2 upon stimulation with recombinant zonulin. Further, the barrier-disrupting effect of zonulin was blocked by an EGFR-specific protein tyrosine kinase inhibitor.Citation47 Recently, Goldblum et al. reported that this signaling cascade, activated by the prokaryotic zonulin-analog Zot, ultimately leads to a dissociation of ZO-1 from its transmembrane binding partners occludin and claudin-1 and its cytoskeletal binding partner myosin 1C in intestinal epithelial cells.Citation38 Moreover, ZO-1 and occludin were shown to be displaced from the tight junction. A similar mechanism can be hypothesized for zonulin, but these data are currently missing. Intriguingly, most of the data on PAR2-activation and permeability were performed in the colon,Citation46 a region where zonulin is not active, and a recent paper failed to find an effect of jejunal PAR2-activation on paracellular permeability.Citation48 This apparent contradiction between the effect of zonulin and the distribution of its putative receptor PAR2 needs to be clarified in future studies. Further, it is unclear how the zonulin-regulated pathway relates to the recently described pathways of intestinal paracellular flux.Citation6,Citation49,Citation50 At least two different routes of intestinal paracellular flux are operative: a large-capacity pathway for small solutes (less than 4Å) and water, which was termed the pore pathway, and a small-capacity pathway for larger molecules, the leak pathway.Citation6 The pore pathway is mainly regulated by the expression of claudins, while passage through the leak pathway depends on phosphorylation of the myosin II regulatory light chain by myosin light chain kinase (MLCK) and subsequent contraction of the perijunctional actin-myosin ring. Although passage through the zonulin-regulated pathway involves molecules with a radius exceeding the dimensions of the pore pathway, preliminary reports suggest that MLCK is not involved since a reduction of pMLC was observed after AT1002 application on Caco2 cells.Citation39 In contrast to the light chain of myosin 2,Citation6 a phosphorylation of myosin1C was reported to be involved in the zonulin pathway.Citation38
Structure and function of Haptoglobin
Recently, Fasano’s group identified the molecular identity of zonulin as prehaptoglobin-2 (pre-HP2).Citation47 The gene coding for Haptoglobin (HP) is located on chromosome 16q22, and harbors a common polymorphism consisting of two structural alleles: HP1 and HP2. HP2 is the product of a non-homologous intragenic duplication of exons 3 and 4 of HP1Citation51 (), resulting in three possible genotypes – HP11, HP21 and HP22. HP can be detected in the serum of all mammals, but this particular polymorphism has been reported only in humans.Citation52 The three genotypes correspond to three different HP phenotypes. HP contains two types of polypeptide chains: a β chain, which is common to the three phenotypes, and an α1 or α2 chain, which differentiates HP11 (α1) from HP22 (α2). The three major phenotypes of HP are: an α1–β dimer for HP11; multiple α2–β units (cyclic polymer) for HP22; and a combination of α1–β dimer with multiple α2–β units (linear polymer) for HP21.Citation53,Citation54
Figure 1. Structure of the human HP gene. The human HP2 allele arose by duplication of exons 3 and 4 of the HP1 allele. HP, Haptoglobin; kb, kilobase
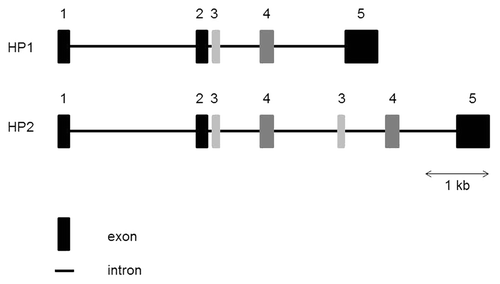
Western blot analysis of human sera with anti-Zot primary antibody demonstrated three different bands: a 9kDa protein band, an 18 kDa protein band and a fainter 45kDa band. Through tandem mass spectrometry (MS/MS), the 9kDa band was identified as the α1-chain of HP1 and the 18 kDa band as the α2-chain of HP2. The ~45kDa protein, which was previously shown to represent zonulin,Citation31 was only present in individuals with a single 18 kDa band, i.e., homozygous for HP2 (HP22). NH2-terminal sequencing and MS/MS analysis revealed the protein as pre-HP2.Citation47 shows the structure of pre-HP1 and pre-HP2, and HP1 and HP2, and how the different proteins can be visualized on western blot. Tripathi et al. further substantiated the functional identity by producing pre-HP2 as a recombinant protein in a baculovirus expression system. Single-chain pre-HP2, but not the two-chain cleaved HP2, dose-dependently increased intestinal permeability when applied ex vivo in a murine small intestine indicator system and also in vivo, characterized by an increase in the lactulose-mannitol ratio in the urine after gavaging the respective sugars in pre-HP2 treated mice.Citation47 These series of experiments confirms the unusual feature of the protein precursor pre-HP2 exerting a completely different function as the mature cleaved HP protein.
Figure 2. The structure of pre-HP1 and pre-HP2, and HP1 and HP2 is depicted on the right. It is also shown how the different proteins can be visualized on western blot (left side). (Adapted fromCitation8). HP, Haptoglobin; MW, molecular weight; kDa, kilo dalton
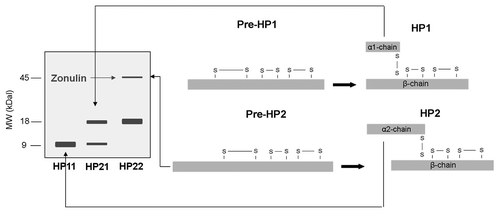
HP is an acute phase α-sialoglycoprotein with hemoglobin-binding capacity. Binding to hemoglobin prevents iron loss and subsequent kidney damage during hemolysis.Citation55 Hemoglobin–HP complexes are removed by binding to the CD163 receptor, which is expressed on the surface of monocytes and macrophages.Citation52 Functional differences between HP1 and HP2 in their ability to protect against hemoglobin-driven oxidative stress have been shown to have important clinical significance. For example, HP22 individuals with diabetes mellitus appear to be at significantly higher risk of microvascular and macrovascular complications.Citation56,Citation57
HP also has been demonstrated to modulate several aspects of the innate and adaptive immune response and to have anti-inflammatory activities.Citation58,Citation59 Hp has the ability to suppress monocyte production of tumor necrosis factor (TNF), interleukin (IL)-10 and IL-12.Citation60 In vivo, in a model of lipopolysaccharide (LPS)-induced bronchopulmonary hyper-reactivity and endotoxic shock, Hp knockout mice were more sensitive than their wild-type counterparts.Citation60 Also, a protective effect of Hp against experimental autoimmune encephalomyelitis, a model characterized by raised pro-inflammatory cytokines such as interferon (IFN)γ, IL-23, IL-12 and IL-17 in the brain, was shown.Citation58 Altogether, Hp seems to have a protective role in reducing the severity of Th1/17-mediated inflammatory processes.
HP is mainly produced by hepatocytes, and to a lesser extent by cells in other tissues such as lung, skin and kidney, under inflammatory conditions. Its synthesis is induced by IL-6, IL-1β and TNF.
In vitro studies have shown some functional differences between HP1 and HP2 protein products, with HP1 having a superior anti-inflammatory effect compared with HP2,Citation61-Citation63 but the exact functional significance of this polymorphism is not well understood. As the HP polymorphism is only present in humans, it is difficult to directly link mouse and human data. Hp in mice is believed to resemble the human HP11, with over 90% homology with the human Hp1 allele.Citation64 Wild-type mice would thus behave more like HP11 in humans, although this remains speculative. To disentangle the difference between HP1 and HP2 in mice in the context of IBD it will be important to study mice genetically modified at the Hp locus to harbor either the Hp1 or Hp2 allele.Citation65
Role of haptoglobin in IBD and other intestinal and immune disorders
Preclinical data
As mentioned above, Hp has been shown to have a protective effect against LPS-induced bronchopulmonary hyper-reactivity and endotoxic shock,Citation60 and against autoimmune encephalomyelitisCitation58 in mouse models.
Studies in mouse models also showed that Hp provides a protective effect against experimentally induced (by dextran sodium sulfate (DSS) and Oxazolone (Oxa)) colitis: Hp knockout mice have more weight loss and higher macroscopic and histological scores as compared with their wild-type littermates.Citation66 Hp thus plays an important modulatory and protective role in inflammatory colitis in experimental models. One way of action is by inhibiting the production of several cytokines (IL-17, IFNγ, TNF and IL-6 in DSS-induced colitis, and IL-13 in Oxa-induced colitis).Citation66 Furthermore, wild-type but not knockout serum inhibited IL-17 production, suggesting that Hp knockout mice preferentially develop Th17 immune responses and that Hp modulates Th17 cells.Citation66 However, these findings do not exclude the possibility that Hp also affects other steps in the inflammatory cascade.
Clinical data
Evidence from genetic studies
Given the immunomodulatory properties of HP, the gene has been studied as a candidate gene in inflammatory diseases such as rheumatoid arthritis (RA), systemic lupus erythematosus (SLE), primary sclerosing cholangitis (PSC) and diabetes mellitus type 2 (T2D), where the HP22 genotype was shown to be over-represented.Citation52,Citation67-Citation69 Two smaller studies looking at the HP polymorphism in Crohn’s disease have produced conflicting resultsCitation70,Citation71: Maza et al. found that HP11 was significantly less common in CD, similar to what was found in other immune-based diseases. However, Papp et al. found a higher frequency of HP11 in CD. In a well-powered study from our group, we found that HP2 is a risk allele for IBD, with a higher frequency in both CD and UC compared with controls (). HP2 was also over-transmitted to affected offspring in CD trios (affected child + parents). In UC trios, the over-transmission—although numerically present—lacked significance, probably because of the smaller size of this specific group. As stated above, murine Hp is believed to resemble mostly human HP1,Citation64 meaning that wild-type mice would thus behave more like Hp11 in humans. Mouse model studies showed that Hp knockout mice are more susceptible to experimentally induced colitis than their wild-type littermatesCitation66 which would fit with the protective effect of the HP1 allele as seen in IBD patients.
Table 1. Distribution of HP alleles and genotypes in healthy controls, CD and UC
In Primary Sclerosing Cholangitis (PSC), a chronic liver disease characterized by inflammation and fibrosis of the intra- and extrahepatic bile ducts and often found as comorbidity in IBD, we found that HP2 and HP22 were more common in patients with this condition than in controls, as was also reported by Papp et al.Citation71 After exclusion of patients who also had IBD, HP22 was still the most common among patients, but the differences between the genotypes were no longer significant, suggesting that either the differences observed were mainly driven by IBD or that the PSC-only study (n = 85 PSC patients) had insufficient power because of small groups.Citation66
The HP locus was not picked up in any of the genome-wide association studies performed in IBD or in the more recently published CD genome-wide association meta-analyses.Citation72-Citation75 This might be explained by the fact that this polymorphism is not a single-nucleotide polymorphism, but an intragenic duplication, which may not have been covered by the single-nucleotide polymorphisms selected on the 6q22 region. However, the region was present in two genome-wide linkage studiesCitation76,Citation77 and also in a genome-wide linkage study meta-analysis.Citation78
Evidence from serological studies
In clinical settings, HP levels are used as a marker for hemolysis.Citation79 Serum levels of HP increase during inflammatory processes.Citation80 A study by Lubega et al. showed that although HP had poor predictive values to discriminate between patients with and without active disease, there was a significant difference in HP levels between active and inactive patients, both in CD and in UC.Citation81
Interestingly, we found that serum levels of HP are significantly lower in healthy individuals with genotype HP22 than in healthy individuals with genotype HP11 and HP21 (): HP11: 1.38 g/l; HP21: 1.38 g/l; HP22: 0.89 g/l. The majority of the samples however fell within the normal range (0.3–2 g/l). It is however possible that we underestimated the real differences between the genotypes: The different subtypes of HP have different molecular mass and different structures depending on the genotype, and these differences can affect serum levels. Moreover, the concentrations of HP22 in inflamed tissues may also be lower because of the higher molecular mass and therefore lower tendency to leave the vessels.
Role of pre-haptoglobin (zonulin) in GI disorders
Celiac Disease
Celiac disease is an immune-mediated enteropathy, triggered by dietary gluten in genetically predisposed individuals.Citation82 Changes in intestinal permeability have been implicated in pathogenesis of celiac disease, but the underlying mechanism was unclear.Citation83,Citation84 In the initial description of zonulin as the eukaryotic analog of Zot, increased zonulin protein concentration in patients with active celiac disease was reported.Citation9 They went on to demonstrate that exposure of IEC-6 intestinal cells to gliadin induced zonulin secretion and a AT1001-sensitive, PKCα-dependent actin polymerization and dissociation between ZO-1 and occludin, similar to what had been described for Zot and AT1002.Citation38,Citation43 Paracellular permeability of rabbit small intestine mounted in Ussing chambers, was increased by adding gliadin to the mucosal compartment, but not when added to the serosal side. This effect of gliadin on permeability was abolished by AT1001 pre-treatment.Citation43 Similarly, gliadin induced zonulin release and higher permeability in vitro in biopsies of both healthy volunteers and patients with quiescent celiac disease.Citation85 However, both the baseline permeability and permeability after addition of gliadin were higher in patients with celiac disease and the luminal zonulin release was more pronounced and prolonged in patients. In follow-up studies, the chemokine receptor CXCR3 was shown to be the epithelial receptor for gliadin with subsequent recruitment of the adaptor protein MyD88.Citation86
To confirm the contribution of zonulin to early permeability changes in celiac disease, 20 patients on gluten-free diet were randomized to a 3 d AT1001 treatment (12mg once daily) or placebo with a 2.5g oral gluten challenge on the second day.Citation34 Intestinal permeability was tested daily by a lactulose-mannitol urinary excretion test. In the placebo group a significant 70% increase in intestinal permeability was observed after gluten challenge in contrast to the AT1001 treated patients in whom no changes in permeability were observed. However, the difference between both groups failed to reach statistical significance. IFNγ increased in both treatment groups without a significant difference between groups. Leffler et al. evaluated a two week treatment with different doses of larazotide (AT1001), ranging from 0.5 to 8mg tid, with daily gluten challenge in 86 celiac disease patients on a gluten-free diet.Citation35 The primary endpoint, a difference in intestinal permeability, was not met in this study because of a large variability of the lactulose-mannitol ratios in this study. However, significantly less gastrointestinal symptoms were observed in patients in the active treatment arm.Citation35 In a second study from the same group, evaluating 1, 4 and 8 mg of larazotide tid vs. placebo in 184 patients with daily gluten challenge for 6 wk, gastrointestinal symptoms and anti-tissue transglutaminase IgA antibodies were lower in the active treatment groups. In contrast, and similar to the previous study, the urinary lactulose-mannitol ratio was similar in both groups.Citation87
Taken together, these data suggest the involvement of zonulin in gluten-induced intestinal hyperpermeability in patients with celiac disease. Randomized clinical trials suggest that gluten-induced symptoms were reduced by the zonulin-antagonist larazotide. However, no effect on the lactulose-mannitol ratio, a measure for in vivo permeability, was observed. Whether this is related to shortcomings of the measurement technique or whether other effects apart from its effect on permeability play a role in the reduction of symptoms, remains to be demonstrated.
Inflammatory Bowel Disease
Clinical data on zonulin and IBD are currently not available. Arrieta et al. evaluated the effect of AT1001 in interleukin (IL) 10 knockout mice.Citation33 This model is characterized by increased small intestinal permeability,Citation88 preceding a chronic, patchy colitis which is dependent on luminal factors since germ-free animals are protected from disease.Citation89,Citation90 AT1001 treatment started from weaning age dose-dependently reduced intestinal permeability. Moreover, colitis was prevented in the animals treated with high dose AT1001, in which small intestinal permeability was also reduced and comparable to wild-type animals. To confirm that the conferred protection was not due an effect on colonic permeability, the AT1002 was evaluated in wild-type and IL10−/− mice. AT1002 increased small intestinal permeability but did not affect the colonic barrier function, confirming earlier data on the distribution of the zonulin-receptor.Citation33
Extra-intestinal disorders
The zonulin pathway has also been implicated in the pathogenesis of type 1 diabetes,Citation10,Citation32,Citation91 metabolic syndrome,Citation92,Citation93 sepsisCitation94 and animal models of acute lung injury.Citation95 A detailed discussion of the extra-intestinal effects of zonulin is beyond the scope of the current review.
Conclusion
With the identification and confirmation of HP2 as a risk allele for inflammatory bowel disease, the next question to answer is how HP affects disease pathogenesis. One possible explanation is linked to the immunomodulatory effect of HP. Based on mouse model studies it can be hypothesized that Hp has a role in reducing the severity of inflammation and modulating IL-17 production, a cytokine thought to be highly important in the pathogenesis of IBD. However, another hypothesis could be related to zonulin. With zonulin being a major physiological mediator of paracellular intestinal permeability, and its identification as pre-HP2, it is not unlikely that carriers of the zonulin gene (i.e., individuals with genotype HP21 or HP22) could possibly have an increased risk to develop IBD, because of the permeating effect of zonulin on the intestinal barrier. Translational and functional studies addressing the role of zonulin in IBD, and its relation with intestinal permeability, will be important to further answer this intriguing question.
shows a summary diagram of the different ways HP(2) could be involved in IBD pathogenesis.
References
- Turner JR. Intestinal mucosal barrier function in health and disease. Nat Rev Immunol 2009; 9:799 - 809; http://dx.doi.org/10.1038/nri2653; PMID: 19855405
- Powell DW. Barrier function of epithelia. Am J Physiol 1981; 241:G275 - 88; PMID: 7032321
- Laukoetter MG, Bruewer M, Nusrat A. Regulation of the intestinal epithelial barrier by the apical junctional complex. Curr Opin Gastroenterol 2006; 22:85 - 9; http://dx.doi.org/10.1097/01.mog.0000203864.48255.4f; PMID: 16462161
- Fanning AS, Jameson BJ, Jesaitis LA, Anderson JM. The tight junction protein ZO-1 establishes a link between the transmembrane protein occludin and the actin cytoskeleton. J Biol Chem 1998; 273:29745 - 53; http://dx.doi.org/10.1074/jbc.273.45.29745; PMID: 9792688
- Van Itallie CM, Anderson JM. Claudins and epithelial paracellular transport. Annu Rev Physiol 2006; 68:403 - 29; http://dx.doi.org/10.1146/annurev.physiol.68.040104.131404; PMID: 16460278
- Shen L, Weber CR, Raleigh DR, Yu D, Turner JR. Tight junction pore and leak pathways: a dynamic duo. Annu Rev Physiol 2011; 73:283 - 309; http://dx.doi.org/10.1146/annurev-physiol-012110-142150; PMID: 20936941
- Shen L, Weber CR, Turner JR. The tight junction protein complex undergoes rapid and continuous molecular remodeling at steady state. J Cell Biol 2008; 181:683 - 95; http://dx.doi.org/10.1083/jcb.200711165; PMID: 18474622
- Fasano A. Zonulin and its regulation of intestinal barrier function: the biological door to inflammation, autoimmunity, and cancer. Physiol Rev 2011; 91:151 - 75; http://dx.doi.org/10.1152/physrev.00003.2008; PMID: 21248165
- Fasano A, Not T, Wang W, Uzzau S, Berti I, Tommasini A, Goldblum SE. Zonulin, a newly discovered modulator of intestinal permeability, and its expression in coeliac disease. Lancet 2000; 355:1518 - 9; http://dx.doi.org/10.1016/S0140-6736(00)02169-3; PMID: 10801176
- Sapone A, de Magistris L, Pietzak M, Clemente MG, Tripathi A, Cucca F, Lampis R, Kryszak D, Cartenì M, Generoso M, et al. Zonulin upregulation is associated with increased gut permeability in subjects with type 1 diabetes and their relatives. Diabetes 2006; 55:1443 - 9; http://dx.doi.org/10.2337/db05-1593; PMID: 16644703
- Buhner S, Buning C, Genschel J, Kling K, Herrmann D, Dignass A, Kuechler I, Krueger S, Schmidt HH, Lochs H. Genetic basis for increased intestinal permeability in families with Crohn’s disease: role of CARD15 3020insC mutation?. Gut 2006; 55:342 - 7; http://dx.doi.org/10.1136/gut.2005.065557; PMID: 16000642
- Peeters M, Geypens B, Claus D, Nevens H, Ghoos Y, Verbeke G, Baert F, Vermeire S, Vlietinck R, Rutgeerts P. Clustering of increased small intestinal permeability in families with Crohn’s disease. Gastroenterology 1997; 113:802 - 7; http://dx.doi.org/10.1016/S0016-5085(97)70174-4; PMID: 9287971
- Teahon K, Smethurst P, Levi AJ, Menzies IS, Bjarnason I. Intestinal permeability in patients with Crohn’s disease and their first degree relatives. Gut 1992; 33:320 - 3; http://dx.doi.org/10.1136/gut.33.3.320; PMID: 1568650
- Büning C, Geissler N, Prager M, Sturm A, Baumgart DC, Büttner J, Bühner S, Haas V, Lochs H. Increased small intestinal permeability in ulcerative colitis: rather genetic than environmental and a risk factor for extensive disease?. Inflamm Bowel Dis 2012; 18:1932 - 9; http://dx.doi.org/10.1002/ibd.22909; PMID: 22344959
- Gerova VA, Stoynov SG, Katsarov DS, Svinarov DA. Increased intestinal permeability in inflammatory bowel diseases assessed by iohexol test. World J Gastroenterol 2011; 17:2211 - 5; PMID: 21633531
- Wyatt J, Vogelsang H, Hübl W, Waldhöer T, Lochs H. Intestinal permeability and the prediction of relapse in Crohn’s disease. Lancet 1993; 341:1437 - 9; http://dx.doi.org/10.1016/0140-6736(93)90882-H; PMID: 8099141
- Ng SC, Tang W, Ching JY, Wong M, Chow CM, Hui AJ, Wong TC, Leung VK, Tsang SW, Yu HH, et al, Asia–Pacific Crohn’s and Colitis Epidemiologic Study (ACCESS) Study Group. Incidence and phenotype of inflammatory bowel disease based on results from the Asia-pacific Crohn’s and colitis epidemiology study. Gastroenterology 2013; 145:158 - 65, e2; http://dx.doi.org/10.1053/j.gastro.2013.04.007; PMID: 23583432
- Molodecky NA, Soon IS, Rabi DM, Ghali WA, Ferris M, Chernoff G, Benchimol EI, Panaccione R, Ghosh S, Barkema HW, et al. Increasing incidence and prevalence of the inflammatory bowel diseases with time, based on systematic review. Gastroenterology 2012; 142:46 - 54, e42, quiz e30; http://dx.doi.org/10.1053/j.gastro.2011.10.001; PMID: 22001864
- Bouma G, Strober W. The immunological and genetic basis of inflammatory bowel disease. Nat Rev Immunol 2003; 3:521 - 33; http://dx.doi.org/10.1038/nri1132; PMID: 12876555
- Xavier RJ, Podolsky DK. Unravelling the pathogenesis of inflammatory bowel disease. Nature 2007; 448:427 - 34; http://dx.doi.org/10.1038/nature06005; PMID: 17653185
- Madsen KL, Malfair D, Gray D, Doyle JS, Jewell LD, Fedorak RN. Interleukin-10 gene-deficient mice develop a primary intestinal permeability defect in response to enteric microflora. Inflamm Bowel Dis 1999; 5:262 - 70; http://dx.doi.org/10.1097/00054725-199911000-00004; PMID: 10579119
- Irvine EJ, Marshall JK. Increased intestinal permeability precedes the onset of Crohn’s disease in a subject with familial risk. Gastroenterology 2000; 119:1740 - 4; http://dx.doi.org/10.1053/gast.2000.20231; PMID: 11113095
- Baudry B, Fasano A, Ketley J, Kaper JB. Cloning of a gene (zot) encoding a new toxin produced by Vibrio cholerae. Infect Immun 1992; 60:428 - 34; PMID: 1730472
- Fasano A, Baudry B, Pumplin DW, Wasserman SS, Tall BD, Ketley JM, Kaper JB. Vibrio cholerae produces a second enterotoxin, which affects intestinal tight junctions. Proc Natl Acad Sci U S A 1991; 88:5242 - 6; http://dx.doi.org/10.1073/pnas.88.12.5242; PMID: 2052603
- Uzzau S, Cappuccinelli P, Fasano A. Expression of Vibrio cholerae zonula occludens toxin and analysis of its subcellular localization. Microb Pathog 1999; 27:377 - 85; http://dx.doi.org/10.1006/mpat.1999.0312; PMID: 10588910
- Di Pierro M, Lu R, Uzzau S, Wang W, Margaretten K, Pazzani C, Maimone F, Fasano A. Zonula occludens toxin structure-function analysis. Identification of the fragment biologically active on tight junctions and of the zonulin receptor binding domain. J Biol Chem 2001; 276:19160 - 5; http://dx.doi.org/10.1074/jbc.M009674200; PMID: 11278543
- Fasano A, Uzzau S, Fiore C, Margaretten K. The enterotoxic effect of zonula occludens toxin on rabbit small intestine involves the paracellular pathway. Gastroenterology 1997; 112:839 - 46; http://dx.doi.org/10.1053/gast.1997.v112.pm9041245; PMID: 9041245
- Song KH, Fasano A, Eddington ND. Effect of the six-mer synthetic peptide (AT1002) fragment of zonula occludens toxin on the intestinal absorption of cyclosporin A. Int J Pharm 2008; 351:8 - 14; http://dx.doi.org/10.1016/j.ijpharm.2007.09.011; PMID: 17954018
- Fasano A, Uzzau S. Modulation of intestinal tight junctions by Zonula occludens toxin permits enteral administration of insulin and other macromolecules in an animal model. J Clin Invest 1997; 99:1158 - 64; http://dx.doi.org/10.1172/JCI119271; PMID: 9077522
- Fasano A, Fiorentini C, Donelli G, Uzzau S, Kaper JB, Margaretten K, Ding X, Guandalini S, Comstock L, Goldblum SE. Zonula occludens toxin modulates tight junctions through protein kinase C-dependent actin reorganization, in vitro. J Clin Invest 1995; 96:710 - 20; http://dx.doi.org/10.1172/JCI118114; PMID: 7635964
- Wang W, Uzzau S, Goldblum SE, Fasano A. Human zonulin, a potential modulator of intestinal tight junctions. J Cell Sci 2000; 113:4435 - 40; PMID: 11082037
- Watts T, Berti I, Sapone A, Gerarduzzi T, Not T, Zielke R, Fasano A. Role of the intestinal tight junction modulator zonulin in the pathogenesis of type I diabetes in BB diabetic-prone rats. Proc Natl Acad Sci U S A 2005; 102:2916 - 21; http://dx.doi.org/10.1073/pnas.0500178102; PMID: 15710870
- Arrieta MC, Madsen K, Doyle J, Meddings J. Reducing small intestinal permeability attenuates colitis in the IL10 gene-deficient mouse. Gut 2009; 58:41 - 8; http://dx.doi.org/10.1136/gut.2008.150888; PMID: 18829978
- Paterson BM, Lammers KM, Arrieta MC, Fasano A, Meddings JB. The safety, tolerance, pharmacokinetic and pharmacodynamic effects of single doses of AT-1001 in coeliac disease subjects: a proof of concept study. Aliment Pharmacol Ther 2007; 26:757 - 66; http://dx.doi.org/10.1111/j.1365-2036.2007.03413.x; PMID: 17697209
- Leffler DA, Kelly CP, Abdallah HZ, Colatrella AM, Harris LA, Leon F, Arterburn LA, Paterson BM, Lan ZH, Murray JA. A randomized, double-blind study of larazotide acetate to prevent the activation of celiac disease during gluten challenge. Am J Gastroenterol 2012; 107:1554 - 62; http://dx.doi.org/10.1038/ajg.2012.211; PMID: 22825365
- Gopalakrishnan S, Durai M, Kitchens K, Tamiz AP, Somerville R, Ginski M, Paterson BM, Murray JA, Verdu EF, Alkan SS, et al. Larazotide acetate regulates epithelial tight junctions in vitro and in vivo. Peptides 2012; 35:86 - 94; http://dx.doi.org/10.1016/j.peptides.2012.02.015; PMID: 22401908
- Kelly CP, Green PH, Murray JA, Dimarino A, Colatrella A, Leffler DA, Alexander T, Arsenescu R, Leon F, Jiang JG, et al, Larazotide Acetate Celiac Disease Study Group. Larazotide acetate in patients with coeliac disease undergoing a gluten challenge: a randomised placebo-controlled study. Aliment Pharmacol Ther 2013; 37:252 - 62; http://dx.doi.org/10.1111/apt.12147; PMID: 23163616
- Goldblum SE, Rai U, Tripathi A, Thakar M, De Leo L, Di Toro N, Not T, Ramachandran R, Puche AC, Hollenberg MD, et al. The active Zot domain (aa 288-293) increases ZO-1 and myosin 1C serine/threonine phosphorylation, alters interaction between ZO-1 and its binding partners, and induces tight junction disassembly through proteinase activated receptor 2 activation. FASEB J 2011; 25:144 - 58; http://dx.doi.org/10.1096/fj.10-158972; PMID: 20852064
- Gopalakrishnan S, Pandey N, Tamiz AP, Vere J, Carrasco R, Somerville R, Tripathi A, Ginski M, Paterson BM, Alkan SS. Mechanism of action of ZOT-derived peptide AT-1002, a tight junction regulator and absorption enhancer. Int J Pharm 2009; 365:121 - 30; http://dx.doi.org/10.1016/j.ijpharm.2008.08.047; PMID: 18832018
- Li M, Oliver E, Kitchens KM, Vere J, Alkan SS, Tamiz AP. Structure-activity relationship studies of permeability modulating peptide AT-1002. Bioorg Med Chem Lett 2008; 18:4584 - 6; http://dx.doi.org/10.1016/j.bmcl.2008.07.028; PMID: 18667315
- Song KH, Eddington ND. The impact of AT1002 on the delivery of ritonavir in the presence of bioadhesive polymer, carrageenan. Arch Pharm Res 2012; 35:937 - 43; http://dx.doi.org/10.1007/s12272-012-0520-1; PMID: 22644862
- El Asmar R, Panigrahi P, Bamford P, Berti I, Not T, Coppa GV, Catassi C, Fasano A. Host-dependent zonulin secretion causes the impairment of the small intestine barrier function after bacterial exposure. Gastroenterology 2002; 123:1607 - 15; http://dx.doi.org/10.1053/gast.2002.36578; PMID: 12404235
- Clemente MG, De Virgiliis S, Kang JS, Macatagney R, Musu MP, Di Pierro MR, Drago S, Congia M, Fasano A. Early effects of gliadin on enterocyte intracellular signalling involved in intestinal barrier function. Gut 2003; 52:218 - 23; http://dx.doi.org/10.1136/gut.52.2.218; PMID: 12524403
- Tripathi A, Lammers KM, Goldblum S, Shea-Donohue T, Netzel-Arnett S, Buzza MS, Antalis TM, Vogel SN, Zhao A, Yang S, et al. Identification of human zonulin, a physiological modulator of tight junctions, as prehaptoglobin-2. Proc Natl Acad Sci U S A 2009; 106:16799 - 804; http://dx.doi.org/10.1073/pnas.0906773106; PMID: 19805376
- Macfarlane SR, Seatter MJ, Kanke T, Hunter GD, Plevin R. Proteinase-activated receptors. Pharmacol Rev 2001; 53:245 - 82; PMID: 11356985
- Bueno L, Fioramonti J. Protease-activated receptor 2 and gut permeability: a review. Neurogastroenterol Motil 2008; 20:580 - 7; http://dx.doi.org/10.1111/j.1365-2982.2008.01139.x; PMID: 18482083
- Tripathi A, Lammers KM, Goldblum S, Shea-Donohue T, Netzel-Arnett S, Buzza MS, Antalis TM, Vogel SN, Zhao A, Yang S, et al. Identification of human zonulin, a physiological modulator of tight junctions, as prehaptoglobin-2. Proc Natl Acad Sci U S A 2009; 106:16799 - 804; http://dx.doi.org/10.1073/pnas.0906773106; PMID: 19805376
- Fernández-Blanco JA, Hollenberg MD, Martínez V, Vergara P. PAR-2-mediated control of barrier function and motility differs between early and late phases of postinfectious gut dysfunction in the rat. Am J Physiol Gastrointest Liver Physiol 2013; 304:G390 - 400; http://dx.doi.org/10.1152/ajpgi.00387.2012; PMID: 23238933
- Van Itallie CM, Holmes J, Bridges A, Gookin JL, Coccaro MR, Proctor W, Colegio OR, Anderson JM. The density of small tight junction pores varies among cell types and is increased by expression of claudin-2. J Cell Sci 2008; 121:298 - 305; http://dx.doi.org/10.1242/jcs.021485; PMID: 18198187
- Watson CJ, Hoare CJ, Garrod DR, Carlson GL, Warhurst G. Interferon-gamma selectively increases epithelial permeability to large molecules by activating different populations of paracellular pores. J Cell Sci 2005; 118:5221 - 30; http://dx.doi.org/10.1242/jcs.02630; PMID: 16249235
- Maeda N, Yang F, Barnett DR, Bowman BH, Smithies O. Duplication within the haptoglobin Hp2 gene. Nature 1984; 309:131 - 5; http://dx.doi.org/10.1038/309131a0; PMID: 6325933
- Levy AP, Asleh R, Blum S, Levy NS, Miller-Lotan R, Kalet-Litman S, Anbinder Y, Lache O, Nakhoul FM, Asaf R, et al. Haptoglobin: basic and clinical aspects. Antioxid Redox Signal 2010; 12:293 - 304; http://dx.doi.org/10.1089/ars.2009.2793; PMID: 19659435
- Koch W, Latz W, Eichinger M, Roguin A, Levy AP, Schömig A, Kastrati A. Genotyping of the common haptoglobin Hp 1/2 polymorphism based on PCR. Clin Chem 2002; 48:1377 - 82; PMID: 12194911
- Soejima M, Koda Y. TaqMan-based real-time PCR for genotyping common polymorphisms of haptoglobin (HP1 and HP2). Clin Chem 2008; 54:1908 - 13; http://dx.doi.org/10.1373/clinchem.2008.113126; PMID: 18787013
- Sadrzadeh SM, Bozorgmehr J. Haptoglobin phenotypes in health and disorders. Am J Clin Pathol 2004; 121:Suppl S97 - 104; PMID: 15298155
- Levy AP, Hochberg I, Jablonski K, Resnick HE, Lee ET, Best L, Howard BV, Strong Heart Study. Haptoglobin phenotype is an independent risk factor for cardiovascular disease in individuals with diabetes: The Strong Heart Study. J Am Coll Cardiol 2002; 40:1984 - 90; http://dx.doi.org/10.1016/S0735-1097(02)02534-2; PMID: 12475459
- Suleiman M, Aronson D, Asleh R, Kapeliovich MR, Roguin A, Meisel SR, Shochat M, Sulieman A, Reisner SA, Markiewicz W, et al. Haptoglobin polymorphism predicts 30-day mortality and heart failure in patients with diabetes and acute myocardial infarction. Diabetes 2005; 54:2802 - 6; http://dx.doi.org/10.2337/diabetes.54.9.2802; PMID: 16123372
- Galicia G, Maes W, Verbinnen B, Kasran A, Bullens D, Arredouani M, Ceuppens JL. Haptoglobin deficiency facilitates the development of autoimmune inflammation. Eur J Immunol 2009; 39:3404 - 12; http://dx.doi.org/10.1002/eji.200939291; PMID: 19795414
- Van Vlierberghe H, Langlois M, Delanghe J. Haptoglobin polymorphisms and iron homeostasis in health and in disease. Clin Chim Acta 2004; 345:35 - 42; http://dx.doi.org/10.1016/j.cccn.2004.03.016; PMID: 15193975
- Arredouani MS, Kasran A, Vanoirbeek JA, Berger FG, Baumann H, Ceuppens JL. Haptoglobin dampens endotoxin-induced inflammatory effects both in vitro and in vivo. Immunology 2005; 114:263 - 71; http://dx.doi.org/10.1111/j.1365-2567.2004.02071.x; PMID: 15667571
- Arredouani M, Matthijs P, Van Hoeyveld E, Kasran A, Baumann H, Ceuppens JL, Stevens E. Haptoglobin directly affects T cells and suppresses T helper cell type 2 cytokine release. Immunology 2003; 108:144 - 51; http://dx.doi.org/10.1046/j.1365-2567.2003.01569.x; PMID: 12562322
- Arredouani M, Matthys P, Kasran A, Baumann H, Ceuppen JL. Haptoglobin and the Th1/Th2 balance: hints from in vitro and in vivo studies. Redox Rep 2001; 6:369 - 71; http://dx.doi.org/10.1179/135100001101536481; PMID: 11865978
- Guetta J, Strauss M, Levy NS, Fahoum L, Levy AP. Haptoglobin genotype modulates the balance of Th1/Th2 cytokines produced by macrophages exposed to free hemoglobin. Atherosclerosis 2007; 191:48 - 53; http://dx.doi.org/10.1016/j.atherosclerosis.2006.04.032; PMID: 16820150
- Yang F, Linehan LA, Friedrichs WE, Lalley PA, Sakaguchi AY, Bowman BH. Characterization of the mouse haptoglobin gene. Genomics 1993; 18:374 - 80; http://dx.doi.org/10.1006/geno.1993.1478; PMID: 8288241
- Miller-Lotan R, Miller B, Nakhoul F, Aronson D, Asaf R, Levy AP. Retinal capillary basement membrane thickness in diabetic mice genetically modified at the haptoglobin locus. Diabetes Metab Res Rev 2007; 23:152 - 6; http://dx.doi.org/10.1002/dmrr.654; PMID: 16742000
- Márquez L, Shen C, Cleynen I, De Hertogh G, Van Steen K, Machiels K, Perrier C, Ballet V, Organe S, Ferrante M, et al. Effects of haptoglobin polymorphisms and deficiency on susceptibility to inflammatory bowel disease and on severity of murine colitis. Gut 2012; 61:528 - 34; http://dx.doi.org/10.1136/gut.2011.240978; PMID: 21708824
- Dayan L, Levy AP, Blum S, Miller-Lotan R, Melman U, Alshiek J, Jacob G. Haptoglobin genotype and endothelial function in diabetes mellitus: a pilot study. Eur J Appl Physiol 2009; 106:639 - 44; http://dx.doi.org/10.1007/s00421-009-1048-z; PMID: 19347351
- Langlois MR, Delanghe JR. Biological and clinical significance of haptoglobin polymorphism in humans. Clin Chem 1996; 42:1589 - 600; PMID: 8855140
- Pavón EJ, Muñoz P, Lario A, Longobardo V, Carrascal M, Abián J, Martin AB, Arias SA, Callejas-Rubio JL, Sola R, et al. Proteomic analysis of plasma from patients with systemic lupus erythematosus: increased presence of haptoglobin alpha2 polypeptide chains over the alpha1 isoforms. Proteomics 2006; 6:Suppl 1 S282 - 92; http://dx.doi.org/10.1002/pmic.200500404; PMID: 16544281
- Maza I, Miller-Lotan R, Levy AP, Nesher S, Karban A, Eliakim R. The association of Haptoglobin polymorphism with Crohn’s disease in Israel. J Crohns Colitis 2008; 2:214 - 8; http://dx.doi.org/10.1016/j.crohns.2008.03.005; PMID: 21172213
- Papp M, Lakatos PL, Palatka K, Foldi I, Udvardy M, Harsfalvi J, Tornai I, Vitalis Z, Dinya T, Kovacs A, et al, Hungarian IBD Study Group. Haptoglobin polymorphisms are associated with Crohn’s disease, disease behavior, and extraintestinal manifestations in Hungarian patients. Dig Dis Sci 2007; 52:1279 - 84; http://dx.doi.org/10.1007/s10620-006-9615-1; PMID: 17357835
- Anderson CA, Boucher G, Lees CW, Franke A, D’Amato M, Taylor KD, Lee JC, Goyette P, Imielinski M, Latiano A, et al. Meta-analysis identifies 29 additional ulcerative colitis risk loci, increasing the number of confirmed associations to 47. Nat Genet 2011; 43:246 - 52; http://dx.doi.org/10.1038/ng.764; PMID: 21297633
- Barrett JC, Hansoul S, Nicolae DL, Cho JH, Duerr RH, Rioux JD, Brant SR, Silverberg MS, Taylor KD, Barmada MM, et al, NIDDK IBD Genetics Consortium, Belgian-French IBD Consortium, Wellcome Trust Case Control Consortium. Genome-wide association defines more than 30 distinct susceptibility loci for Crohn’s disease. Nat Genet 2008; 40:955 - 62; http://dx.doi.org/10.1038/ng.175; PMID: 18587394
- Franke A, McGovern DP, Barrett JC, Wang K, Radford-Smith GL, Ahmad T, Lees CW, Balschun T, Lee J, Roberts R, et al. Genome-wide meta-analysis increases to 71 the number of confirmed Crohn’s disease susceptibility loci. Nat Genet 2010; 42:1118 - 25; http://dx.doi.org/10.1038/ng.717; PMID: 21102463
- Jostins L, Ripke S, Weersma RK, Duerr RH, McGovern DP, Hui KY, Lee JC, Schumm LP, Sharma Y, Anderson CA, et al, International IBD Genetics Consortium (IIBDGC). Host-microbe interactions have shaped the genetic architecture of inflammatory bowel disease. Nature 2012; 491:119 - 24; http://dx.doi.org/10.1038/nature11582; PMID: 23128233
- Cho JH, Nicolae DL, Gold LH, Fields CT, LaBuda MC, Rohal PM, Pickles MR, Qin L, Fu Y, Mann JS, et al. Identification of novel susceptibility loci for inflammatory bowel disease on chromosomes 1p, 3q, and 4q: evidence for epistasis between 1p and IBD1. Proc Natl Acad Sci U S A 1998; 95:7502 - 7; http://dx.doi.org/10.1073/pnas.95.13.7502; PMID: 9636179
- Williams CN, Kocher K, Lander ES, Daly MJ, Rioux JD. Using a genome-wide scan and meta-analysis to identify a novel IBD locus and confirm previously identified IBD loci. Inflamm Bowel Dis 2002; 8:375 - 81; http://dx.doi.org/10.1097/00054725-200211000-00001; PMID: 12454612
- van Heel DA, Fisher SA, Kirby A, Daly MJ, Rioux JD, Lewis CM, Genome Scan Meta-Analysis Group of the IBD International Genetics Consortium. Inflammatory bowel disease susceptibility loci defined by genome scan meta-analysis of 1952 affected relative pairs. Hum Mol Genet 2004; 13:763 - 70; http://dx.doi.org/10.1093/hmg/ddh090; PMID: 14976156
- Tabbara IA. Hemolytic anemias. Diagnosis and management. Med Clin North Am 1992; 76:649 - 68; PMID: 1578962
- Morimatsu M, Syuto B, Shimada N, Fujinaga T, Yamamoto S, Saito M, Naiki M. Isolation and characterization of bovine haptoglobin from acute phase sera. J Biol Chem 1991; 266:11833 - 7; PMID: 1904872
- Lubega J, Davies TJ. A comparison of serum mucoprotein with serum alpha 1 acid glycoprotein, haptoglobin, and alpha 1 antitrypsin assays in monitoring inflammatory bowel disease. Clin Chim Acta 1990; 188:59 - 69; http://dx.doi.org/10.1016/0009-8981(90)90146-J; PMID: 2347083
- Fasano A, Catassi C. Clinical practice. Celiac disease. N Engl J Med 2012; 367:2419 - 26; http://dx.doi.org/10.1056/NEJMcp1113994; PMID: 23252527
- Schulzke JD, Bentzel CJ, Schulzke I, Riecken EO, Fromm M. Epithelial tight junction structure in the jejunum of children with acute and treated celiac sprue. Pediatr Res 1998; 43:435 - 41; http://dx.doi.org/10.1203/00006450-199804000-00001; PMID: 9544995
- Bjarnason I, Peters TJ, Veall N. A persistent defect in intestinal permeability in coeliac disease demonstrated by a 51Cr-labelled EDTA absorption test. Lancet 1983; 1:323 - 5; http://dx.doi.org/10.1016/S0140-6736(83)91628-8; PMID: 6130333
- Drago S, El Asmar R, Di Pierro M, Grazia Clemente M, Tripathi A, Sapone A, Thakar M, Iacono G, Carroccio A, D’Agate C, et al. Gliadin, zonulin and gut permeability: Effects on celiac and non-celiac intestinal mucosa and intestinal cell lines. Scand J Gastroenterol 2006; 41:408 - 19; http://dx.doi.org/10.1080/00365520500235334; PMID: 16635908
- Lammers KM, Lu R, Brownley J, Lu B, Gerard C, Thomas K, Rallabhandi P, Shea-Donohue T, Tamiz A, Alkan S, et al. Gliadin induces an increase in intestinal permeability and zonulin release by binding to the chemokine receptor CXCR3. Gastroenterology 2008; 135:194 - 204, e3; http://dx.doi.org/10.1053/j.gastro.2008.03.023; PMID: 18485912
- Kelly CP, Green PH, Murray JA, Dimarino A, Colatrella A, Leffler DA, Alexander T, Arsenescu R, Leon F, Jiang JG, et al, Larazotide Acetate Celiac Disease Study Group. Larazotide acetate in patients with coeliac disease undergoing a gluten challenge: a randomised placebo-controlled study. Aliment Pharmacol Ther 2013; 37:252 - 62; http://dx.doi.org/10.1111/apt.12147; PMID: 23163616
- Madsen K, Cornish A, Soper P, McKaigney C, Jijon H, Yachimec C, Doyle J, Jewell L, De Simone C. Probiotic bacteria enhance murine and human intestinal epithelial barrier function. Gastroenterology 2001; 121:580 - 91; http://dx.doi.org/10.1053/gast.2001.27224; PMID: 11522742
- Kühn R, Löhler J, Rennick D, Rajewsky K, Müller W. Interleukin-10-deficient mice develop chronic enterocolitis. Cell 1993; 75:263 - 74; http://dx.doi.org/10.1016/0092-8674(93)80068-P; PMID: 8402911
- Madsen KL, Malfair D, Gray D, Doyle JS, Jewell LD, Fedorak RN. Interleukin-10 gene-deficient mice develop a primary intestinal permeability defect in response to enteric microflora. Inflamm Bowel Dis 1999; 5:262 - 70; http://dx.doi.org/10.1097/00054725-199911000-00004; PMID: 10579119
- Visser JT, Lammers K, Hoogendijk A, Boer MW, Brugman S, Beijer-Liefers S, Zandvoort A, Harmsen H, Welling G, Stellaard F, et al. Restoration of impaired intestinal barrier function by the hydrolysed casein diet contributes to the prevention of type 1 diabetes in the diabetes-prone BioBreeding rat. Diabetologia 2010; 53:2621 - 8; http://dx.doi.org/10.1007/s00125-010-1903-9; PMID: 20853098
- Moreno-Navarrete JM, Sabater M, Ortega F, Ricart W, Fernández-Real JM. Circulating zonulin, a marker of intestinal permeability, is increased in association with obesity-associated insulin resistance. PLoS One 2012; 7:e37160; http://dx.doi.org/10.1371/journal.pone.0037160; PMID: 22629362
- Zak-Gołąb A, Kocełak P, Aptekorz M, Zientara M, Juszczyk L, Martirosian G, Chudek J, Olszanecka-Glinianowicz M. Gut microbiota, microinflammation, metabolic profile, and zonulin concentration in obese and normal weight subjects. Int J Endocrinol 2013; 2013:674106; http://dx.doi.org/10.1155/2013/674106; PMID: 23970898
- Klaus DA, Motal MC, Burger-Klepp U, Marschalek C, Schmidt EM, Lebherz-Eichinger D, Krenn CG, Roth GA. Increased plasma zonulin in patients with sepsis. Biochem Med (Zagreb) 2013; 23:107 - 11; http://dx.doi.org/10.11613/BM.2013.013; PMID: 23457771
- Rittirsch D, Flierl MA, Nadeau BA, Day DE, Huber-Lang MS, Grailer JJ, Zetoune FS, Andjelkovic AV, Fasano A, Ward PA. Zonulin as prehaptoglobin2 regulates lung permeability and activates the complement system. Am J Physiol Lung Cell Mol Physiol 2013; 304:L863 - 72; http://dx.doi.org/10.1152/ajplung.00196.2012; PMID: 23564505