Abstract
A cloud point extraction procedure is proposed for preconcentration of trace amounts of palladium (II), silver (I) and gold (III) in aqueous medium. The metal ions in the initial aqueous solution were extracted using the non-ionic surfactant, Triton X-114 after complex formation with 4-(p-chlorophenyl)-1-(pyridin-2-yl)thiosemicarbazide at pH 6.0 in the presence of 0.3 mol L−1 sodium sulfate as a salting-out agent at 25 °C. Dilution of the surfactant-rich phase with acidified methanol was performed after phase separation, and the metal ions were determined by electrothermal atomic absorption spectrometry. The main factors affecting extraction procedure, such as pH, concentration of the ligand, and amount of Triton X-114 were studied in detail. Under the optimum experimental conditions, the calibration graphs were linear upto 125, 50 and 100 μg L−1 and the enrichment factors were 52, 46 and 56 for palladium, silver and gold, respectively. The limits of detection, based on three times of standard deviation of blank signal by 10 replicate measurements divided by the slope of calibration curves were 0.12, 0.08 and 0.30 μg L−1 for palladium, silver and gold, respectively. The accuracy of the results was verified by analyzing spiked water samples. The proposed method has been applied for the determination of the metal ions in soil and rock samples with satisfactory results.
1 Introduction
Noble metals such as palladium (Pd), silver (Ag) and gold (Au) have many applications in our life [Citation1,Citation2]. These metals occur in the environment at trace and ultratrace levels. Therefore, a very sensitive method is necessary for the accurate and precise determinations. For this purpose, several analytical methods have been developed to measure these ions in real samples including: spectrophotometry, flame atomic absorption spectrometry (FAAS), inductively coupled plasma-mass spectrometry (ICP-MS), inductively coupled plasma-atomic emission spectrometry (ICP-AES), and electrochemical methods.
Electrothermal atomic absorption spectrometry (ETAAS) is a good technique for the determination of ultra-trace amounts of heavy metals in several types of samples due to its sensitivity. But by reason of ultra-low concentration of metals and high concentration of interfering matrix components in most real samples, ETAAS often requires a suitable pretreatment step.
A number of separation/preconcentration methods have been reported for trace metal determinations: these include solid phase extraction [Citation3Citation[4]Citation[5]–Citation6], co-precipitation [Citation7,Citation8], column extraction [Citation9,Citation10], ion-selective electrode [Citation11,Citation12], liquid–liquid extraction [Citation13Citation[14]–Citation15] and cloud point extraction (CPE) [Citation16Citation[17]Citation[18]Citation[19]Citation[20]Citation[21]Citation[22]Citation[23]Citation[24]Citation[25]–Citation26]. CPE has attracted a considerable attention because it complies with the “Green Chemistry” principle [Citation27], as the amount of organic solvent is much less than that of traditional liquid extraction. Moreover, it is simple, cheap, highly efficient, and rapid and of lower toxicity than those used organic solvents.
The cloud point is the temperature above which aqueous solutions of non-ionic surfactants become turbid. Specifically, above the cloud point the solution is separated into two phases: a rich phase containing a high surfactant concentration in a small volume and a poor phase with a surfactant concentration close to the critical micelle concentration [Citation28,Citation29]. Accordingly, any metal ions that either directly interact with micelles or after prerequisite binding with hydrophobic chelating ligand, can be extracted from the parent solution by CPE procedure. Trace elements can be extracted to the surfactant-rich phase usually after formation of a hydrophobic complex with an appropriate chelating agent [Citation30].
In the present study, we applied CPE for the separation and preconcentration of Pd(II), Ag(I) and Au(III) ions from aqueous solutions by using Triton X-114 as a nonionic surfactant. A thioamide derivative, 4-(p-chlorophenyl)-1-(pyridin-2-yl)thiosemicarbazide (HCPTS), was used as the chelating agent. The factors influencing the efficiency of CPE such as effects of pH, Triton X-114, HCPTS concentrations were systematically studied. Also, we introduced a salting-out procedure that enables the room temperature phase separation. The developed CPE procedure was successfully applied to determine the concentration of Pd(II), Ag(I) and Au(III) ions in some environmental samples after the method was validated.
2 Experimental
2.1 Reagents and solutions
All aqueous solutions were prepared with ultrapure water that had been obtained by Milli-Q water purification system (Millipore, Bedford, MA, USA). Reagents used were of analytical grade from Sigma–Aldrich (St. Loius, MO, USA) or Merck (Darmstadt, Germany). The laboratory glassware was kept overnight in 10% v/v HNO3 solution. Before the use, the glassware was washed with deionized water and dried in a dust free environment. The standard solutions of Au3+ were prepared from 1000 mg L−1 Au standard stock solution. The Pd(II) solution was prepared by dissolving 0.1664 g of PdCl2 in 100 mL 0.1 mol L−1 HCl solution and was standardized gravimetrically by dimethylglyoxime method [Citation31]. A stock solution of 1000 mg L−1 Ag+ was prepared by dissolving 0.1575 g silver nitrate in 0.1 mol L−1 HNO3 and diluting to 100 mL in a volumetric flask. It was standardized by using standard NaCl solution [Citation31]. Working solutions were freshly prepared from the stock solution by dilutions with deionized water. Triton X-114 was used without further purification. HCPTS was prepared from 2-hydrazinopyridine and p-chlorophenylisothiocyanate as previously reported [Citation32]. One mmol L−1 solution of HCPTS was prepared by dissolving appropriate amount in 100 mL ethanol. Buffer solutions were prepared by 1 mol L−1 hydrochloric acid – 1 mol L−1 sodium acetate (pH 0.5–3.5); 0.2 mol L−1 acetic acid – 0.2 mol L−1 sodium acetate (pH 4.0–7.0); 2 mol L−1 ammonium chloride – 2 mol L−1 ammonium hydroxide (pH 7.5–10.0).
2.2 Apparatus
A Perkin Elmer atomic absorption spectrophotometer (Model AAnalyst 800) with a longitudinal Zeeman background correction furnished with a transversely heated graphite atomizer (THGA) was used for determination of the metal ions. Sample solutions were injected into the atomizer using AS-800 auto sampler. The sample injection volume was 20 μL. The system is equipped with win Lab 32 software. The instrumental parameters and temperature program for the graphite atomizer were summarized in . The solution pH was adjusted using Hanna instrument model 8519 digital pH meter. A centrifuge model of CH90-2 (Hinotek Technology Co. Ltd., China) was used to accelerate the phase separation process. A thermostatic bath was operated to reach the cloud point temperature when the room temperature was below 25 °C. Digestion of the geological samples was carried out in a CEM MDS 2000 microwave digestion system (Matthews, NC, USA).
Table 1 Instrumental parameters for determination of Pd, Ag and Au using ETAAS.
2.3 Recommended procedure of CPE
For the CPE, the following procedure was followed: into aliquots of a solution containing the analyte, add sodium sulfate solution (0.3 mol L−1) to reach the appropriate ionic strength then buffer the solution to pH 6.0. The metal ions were then complexed with HCPTS (5 × 10−5 mol L−1) followed by the addition of Triton X-114 at 0.05% (w/v) concentration. The extraction was carried out at room temperature (25 °C). The phase separation is accelerated by centrifuging at 4000 rpm for 10 min. The phases were cooled down in an ice bath in order to increase the viscosity of the surfactant rich phase. The bulk aqueous phase was decanted by inverting the tube and dried in water bath. The surfactant rich phase in the tube was made up to 1.0 ml by adding mixture of methanol/conc. HNO3 (5:1). The samples were introduced into ETAAS for the determination of the selected metal ions.
2.4 Preparation of real samples
2.4.1 Water samples
The water samples were filtered firstly through filter paper to separate the coarse particles and suspended matter and secondly through a Millipore cellulose nitrate membrane (pore size 0.45 μm), acidified to pH 1 with HNO3 and stored in a refrigerator in a dark polyethylene bottle. 10 ml water sample was subjected to the CPE methodology as described above.
2.4.2 Geological samples
The road dust and rock samples were collected from different area in Sakakah city, Saudi Arabia. The samples were dried at 90 °C for 2 h, ground, passed through a sieve of 120 meshes and homogenized. 5 mL of concentrated HNO3, 2 mL of concentrated HF and 2 mL of deionized water were added to 0.5 g of the sample in a Teflon vessel and digested in the microwave digestion oven by applying the heating program shown in . Finally, 20 mL of 5% w/v boric acid was added, to neutralize excess HF, and the solution was filtered and brought to a final volume of 50 mL with deionized water. 10 ml aliquots of the final clear solution obtained were analyzed by the prescribed procedure.
Table 2 Microwave digestion program for the digestion of the geological samples.
3 Result and discussion
3.1 Optimization of the CPE procedure
In order to obtain maximum extraction efficiency by CPE method, several parameters need to be taken into account before analysis of the real samples. The most important are: (1) pH of the sample solution, (2) ligand concentration, (3) surfactant concentration, (4) salting-out effect.
3.1.1 Effect of pH
The pH is a very critical parameter for both the coacervation of the micelles and the complexation of the ligand with the metal ions. The effect of the pH on metal ions extraction was assessed by varying the pH from 1 to 10 by using the suitable buffer. As can be seen from , the optimal extraction efficiency was observed for pH values ranging from 6.0 to 8.0, which is lower than the precipitation of the hydroxide of most transition metals [Citation33]. The optimal extraction is probably due to the formation of a HCPTS neutral complex species at this pH range. This explanation was confirmed by our previous study [Citation34]. For the rest of the optimization process, a pH of 6.0 was used.
3.1.2 Effect of ligand concentration
Chelating reagents are extracted probably due to a specific interaction, such as hydrogen bonding, between functional groups on the chelating reagents and ether oxygen or terminal OH group of the non-ionic surfactant and chelates are extracted into the hydrophobic part of aggregated micelles [Citation30]. In this work, HCPTS was used as a chelating agent because thiosemicarbazides produce sufficiently hydrophobic complexes with most of transition metals [Citation34Citation[35]–Citation37]. A 50 mL solution containing 20 μg L−1 of the metal ion, 0.05% (w/v) Triton X-114, at a medium buffer of pH 6.0 containing various amounts of HCPTS (10−6 – 10−4 mol L−1), was subjected to the CPE process. The results are shown in . It can be seen that the extraction recovery for the metal ions increases with increasing the ligand concentration and remains constant above 5 × 10−5 mol L−1. Therefore, a concentration of 5 × 10−5 mol L−1 of HCPTS was selected as optimal value in this work.
3.1.3 Effect of Triton X-114 concentration
Triton X-114 was chosen because of its commercial availability in a high purified homogeneous form, low toxicological properties and cost. Also, the high density of the surfactant rich phase facilitates phase separation by centrifugation. Additionally, the cloud point (23–26 °C) of Triton X-114 permits its use in the preconcentration of a large number of molecules and chelates. The variation on the extraction recovery, as a function of the Triton X-114 concentration is expressed in , when 50 ml solution containing cations and all the reagents in the presence of 0.01–0.1% (w/v) Triton X-114 was extracted.
Fig. 3 Effect of the surfactant concentration on the extraction recovery of Pd, Ag and Au ions. Conditions: 20 μg L−1 metal ions; 0.3 mol L−1 Na2SO4; pH, 6.0; 50 ml aqueous solution; 5 × 10−5 mol L−1 HCPTS; 25 °C.
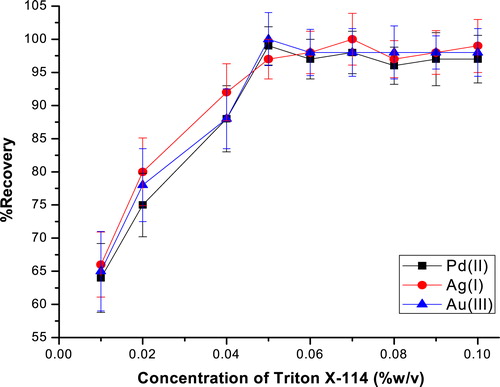
As highlighted in , the recovery is maximum as the Triton X-114 concentration was 0.05% (w/v). At lower concentrations, the extraction efficiency of the complexes was low, due to the inadequacy of the assembly to entrap the complex. Hence, a concentration of 0.05% (w/v) was chosen for further studies.
3.1.4 Salting out effect
Studies on the effects of some additives, such as anionic and non-ionic surfactants and electrolytes, as NaNO3, Na2SO4 and NaCl, on the cloud point behavior of non-ionic surfactants have been reported [Citation38,Citation39] It was observed that the presence of electrolytes decreases the cloud point (salting-out effect), resulting in a more efficient extraction. The lower cloud point is attributed to electrolytes promoting dehydration of the poly(oxyethylene) chains [Citation40]. According to Hiller et al. [Citation41] the salting-out phenomenon is directly related to desorption of ions to the hydrophilic parts of the micelles, increasing inter-attraction between micelles and consequently leading to the precipitation of surfactant molecules. Based on this discussion, the salting-out effect was studied in both the absence and presence of different concentrations of NaNO3, Na2SO4 and NaCl (0.05–1 mol L−1 each) at 25 °C (). It was found that Na2SO4 resulted in the highest recovery, and the recoveries increased with increasing the salt concentration until reach a maximum at concentration of 0.3 mol L−1. This effect may be due to the enhanced hydrophobic interactions among the surfactant aggregates and the analyte as well as the decrease in the cloud point temperature of Triton X-114 in the presence of Na2SO4. At higher concentrations than 0.4 mol L−1, the signals decreased considerably. High concentration of salt can increase the density of water drops accompanied by the surfactant rich phase, and hence disturb the phase separation. On the other hand, the absence of salt decreased the signal by about 55% (data not shown). This may be due to an increase in cloud point temperature leading to incomplete phase separation at 25 °C. Hence 0.3 M was chosen as the optimum concentration.
3.2 Analytical performance
Under the optimum conditions, performance characteristics were obtained by processing standard solutions of Pd(II), Ag(I) and Au(III), and the data is illustrated in . Calibration functions were linear at least up to 125 μg L−1, 50 μg L−1 and 100 μg L−1, for Pd, Ag and Au, respectively. Furthermore, for preconcentration of 50 ml of the working standard solutions based on the slope ratio of calibration curves with and without preconcentration, an enrichment factor of 52, 46 and 56 was obtained for Pd(II), Ag(I) and Au(III), respectively. To test the reproducibility of the proposed extraction method, the suggested procedure was repeated seven times under optimum conditions. The relative standard deviation (R.S.D.) was measured to be 3.4%, 4.7%, and 2.2% for Pd, Ag and Au, respectively.
Table 3 Analytical figures of merit for the proposed method.
Limits of detection (LOD) and quantification (LOQ) for each element were determined according to the expressions at 3 s/m and 10 s/m, respectively (s being, the standard deviation of 10 consecutive blanks and m the slope of the calibration curve). LODs were 0.12 μg L−1 for Pd, 0.08 μg L−1 for Ag and 0.30 μg L−1 for Au and LOQs were 0.45 μg L−1 for Pd, 0.29 μg L−1 for Ag and 1.13 μg L−1 for Au.
3.3 Effect of the potentially interfering ions
The efficiency of the suggested method in the extraction and preconcentration of the three ions in the presence of various cations and anions was examined by using a solution containing 20 μg L−1 of Pd, Ag and Au with addition of various concentrations of potential interferences. The tolerance level was defined as the maximum amount of foreign species producing an error of ±5% in Pd, Ag and Au determination. The tolerance level of each potentially interfering ion species was tested, and if interference occurred, the ratio was reduced until it ceased. As can be seen from , several species did not interfere even at high concentrations, showing that the method described is applicable to the analysis of Pd, Ag and Au ions in different samples.
Table 4 Tolerance limits of interfering ions.
3.4 Application
To test the accuracy of the proposed methodology for assaying the metal ions, it is applied for determination of these metal ions in spiked water samples from different sources. The recoveries of known amounts of Pd, Ag and Au ions added to the samples were examined by the proposed method. The results tabulated in show that the recovery of spiked samples is satisfactory reasonable, which indicates the capability of the method in the determination of the metal ions in real samples containing different matrices under the recommended conditions. To establish the validity of the proposed method, the described procedure was applied for the determination of Pd, Ag and Au in dust and rock samples. The obtained results were summarized in .
Table 5 Analysis of Pd, Ag and Au ions in spiked water samples by the proposed method.
Table 6 Analysis of Pd, Ag and Au ions in geological samples by the recommended procedure.
4 Conclusion
The lipophilicity of HCPTS and its tolerance for the interferences caused by the co-existing metal ions make it feasible for CPE and selective towards Pd, Ag and Au. It permits the separation process at room temperature with the aid of salting-out effect. Compared with traditional CPE (about 40 min for heating, incubation and cooling), the extraction time of the proposed method was very short (a few minutes). The developed procedure yielded good results in terms of selectivity, accuracy and precision for the rapid determination of Pd, Ag and Au in real samples. The proposed methodology was compared to other CPE methods reported recently in the literature [Citation16Citation[17]Citation[18]Citation[19]Citation[20]Citation[21]Citation[22]–Citation23]. The distinct features are summarized in . As can be seen, our results are comparable with, and in some cases better than, the reported ones.
Table 7 Comparison of the present method with previously reported ones.
Notes
Peer review under responsibility of Mansoura University.
References
- F.R.HartleyChemistry of the platinum group metals1991ElsevierAmsterdam
- N.N.Green WoodA.EarnshawChemistry of the elements2nd ed.1997Buttewirth HeinemannOxford
- Y.WuZ.JiangB.HuJ.DuanElectrothermal vaporization inductively coupled plasma atomic emission spectrometry determination of gold, palladium, and platinum using chelating resin YPA4 as both extractant and chemical modifierTalanta632004585592
- Y.QingY.HangR.WanjaulZ.JiangB.HuAdsorption behavior of Noble metal ions (Au, Ag, Pd) on nanometer-size titanium dioxide with ICP-AESAnal Sci19200314171420
- P.YinQ.XuR.QuG.ZhaoY.SunAdsorption of transition metal ions from aqueous solutions onto a novel silica gel matrix inorganic-organic composite materialJ Hazard Mater1732010710716
- R.ChandT.WatariK.InoueH.KawakitaH.N.LuitelD.ParajuliSelective adsorption of precious metal from hydrochloric acid solutions using porous carbon prepared from barley straw and rice huskMiner Eng22200912771282
- M.SoylakM.TuzenCoprecipitation of gold(III), palladium(II) and lead(II) for their flame atomic absorption spectrometric determinationsJ Hazard Mater1522008656661
- S.S.SawantSequential separation and spectrophotometric determination of osmium and platinum with 5-chloro-2-hydroxythiobenzhydrazideAnal Sci252009813818
- Q.HuX.YangZ.HuangJ.ChenG.YangSimultaneous determination of palladium, platinum, rhodium and gold by on-line solid phase extraction and high performance liquid chromatography with 5-(2-hydroxy-5-nitrophenylazo)thiorhodanine as pre-column derivatization regentsJ Chromatogr A109420057782
- J.M.SánchezO.ObrezkovV.SalvadóSeparation of some platinum group metal chelates with 8-hydroxyquinoline by various high-performance liquid chromatographic methodsJ Chromatogr A8712000217226
- M.B.GholivandM.MohammadiM.KhodadadianM.K.RofoueiNovel Platinum(II) Selective Membrane Electrode Based on 1,3-bis(2-cyanobenzene)triazeneTalanta782009922928
- V.K.GuptaR.N.GoyalR.A.SharmaComparative studies of ONNO-based ligands as ionophores for palladium ion-selective membrane sensorsTalanta782009484490
- S.KagayaD.TakataT.YoshimoriT.KanbaraK.TohdaA sensitive and selective method for determination of gold(III) based on electrothermal atomic absorption spectrometry in combination with dispersive liquid–liquid microextraction using dicyclohexylamineTalanta80201013641370
- Y.J.ParkD.J.FrayRecovery of high purity precious metals from printed circuit boardsJ Hazard Mater164200911521158
- L.PanZ.de ZhangSolvent extraction and separation of palladium(II) and platinum(IV) from hydrochloric acid medium with dibutyl sulfoxideMiner Eng22200912711276
- L.TavakoliY.YaminiH.EbrahimzadehA.NezhadaliS.ShariatiF.NourmohammadiDevelopment of cloud point extraction for simultaneous extraction and determination of gold and palladium using ICP-OESJ Hazard Mat1522008737743
- J.L.ManzooriH.Abdolmohammad-ZadehM.AmjadiUltra-trace determination of silver in water samples by electrothermal atomic absorption spectrometry after preconcentration with a ligand-less cloud point extraction methodologyJ Hazard Mater1442007458463
- Z.FanF.BaiDetermination of trace amounts of silver in various samples by electrothermal atomic absorption spectrometry after sample preparation using cloud point extractionAt Spectrosc2820073034
- M.da SilvaV.FrescuraA.CurtiusDetermination of noble metals in biological samples by electrothermal vaporization inductively coupled plasma mass spectrometry, following cloud point extractionSpectrochim Acta B56200119411949
- Y.LianW.ZhenZ.TaiY.YangJ.SongZ.LiCloud point extraction and flame atomic absorption spectrometry analysis of palladium, platinum, and gold ions from industrial polluted soilRare Met312012512516
- N.DalaliN.JavadiY.K.AgrawalOn-line incorporation of cloud point extraction in flame atomic absorption spectrometric determination of silverTurk J Chem322008561570
- H.TavallaliS.YazdandoustM.YazdandoustCloud Point extraction for the preconcentration of silver and palladium in real samples and determination by atomic absorption spectrometryClean – soil, Air, Water382010242247
- M.GhaediA.ShokrollahiK.NiknamE.NiknamA.NajibiM.SoylakCloud point extraction and flame atomic absorption spectrometric determination of cadmium(II), lead(II), palladium(II) silver(I) environ samplesJ Hazard Mater168200910221027
- W.I.MortadaA.Z.AliM.M.HassanienMixed micelle-mediated extraction of alizarin red S complexes of Zr(IV) and Hf(IV) ions prior to their determination by inductively coupled plasma-optical emission spectrometryAnal Meth5201352345240
- W.I.MortadaM.M.HassanienA.A.El-AsmySpeciation of platinum in blood plasma and urine by micelle-mediated extraction and graphite furnace atomic absorption spectrometryJ Trace Elem Med Biol272013267272
- W.I.MortadaM.M.HassanienA.A.El-AsmyCloud point extraction for the determination of trace amounts of Pt(IV) by graphite furnace atomic absorption spectrometryAnal Meth52013530535
- P.AnastasN.EghbaliGreen chemistry: principles and Practice, ChemSoc Rev392010301312
- M.GhaediA.ShokrollahiK.NiknamE.NiknamS.DerkiM.A.SoylakCloud point extraction procedure for preconcentration/flame atomic absorption spectro-metric determination of silver, zinc, and lead at sub-trace levels in environmental samplesJ AOAC Int922009907913
- H.FilikT.CengelR.ApakDetermination of molybdenum (VI) ion in seawater samplesJ Hazard Mater1692009766771
- C.B.OjedaF.S.RojasSeparation and preconcentration by a cloud Point extraction procedure for determination of metals: an overviewAnal Bioanal Chem3942009759782
- G.H.JefferyJ.BassettJ.MendhamR.C.DenneyVogel's textbook of quantitative chemical analysis5th ed.1989Longman Scientific and TechnicalNew York
- M.M.HassanienA.M.HassanW.I.MortadaA.A.El-AsmyA new thioamide derivative for separation and preconcentration of multi elements in aquatic environment by cloud point extractionAm J Anal Chem22011697709
- G.K.SchweitzerL.L.PesterfieldThe aqueous chemistry of the elements2010Oxford University PressNew York
- M.M.HassanienW.I.MortadaA.M.HassanA.A.El-AsmySynthesis, characterization and biological activities of 4-(p-chlorophenyl)-1-(pyridin-2-yl)thiosemicarbazide and its metal complexesJ Korean Chem Soc562012679691
- M.M.HassanienI.M.GabrM.H.Abdel-RhmanA.A.El-AsmySynthesis and structural investigation of mono and polynuclear copper complexes of 4-ethyl-1-(pyridin-2-yl) thiosemicarbazideSpectrochim Acta A Mol Biomol Spectrosc7120087379
- A.A.El-AsmyO.A.Al-GammalD.A.SaadS.E.GhazySynthesis, characterization, molecular modeling and eukaryotic DNA degradation of 1-(3,4-dihydroxybenzylidene)thiosemicarbazide complexesJ Mol Struct9342009922
- A.A.El-AsmyT.Y.Al-AnsiR.R.AminM.MounirSpectral, magnetic and electrical properties of 1-succinyl bis(4-phenylthiosemicarbazide) complexesPolyhedron9200920292034
- W.L.HinzeE.PramauroCritical review of surfactant-mediated phase separation (cloud-point extractions): theory and applicationsCrit Rev Anal Chem241993133177
- C.C.NascentesM.A.Z.ArrudaCloud point formation based on mixed micelles in the presence of electrolytes for cobalt extraction and preconcentrationTalanta612003759768
- J.K.ArmstrongB.Z.ChowdhryM.J.SnowdenS.A.LeharneEffect of sodium chloride upon micellization and phase separation transitions in aqueous solutions of triblock copolymers: a high-sensitivity differential scanning calorimetry studyLangmuir14199820042010
- G.Komaromy-HillerN.CalkinsR.WandruszkaChanges in polarity and aggregation number upon clouding of a nonionic detergent: effect of ionic surfactants and sodium chlorideLangmuir121996916920