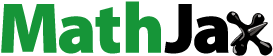
Abstract
In this paper, mathematical models of biofilteration of mixtures of hydrophilic (methanol) and hydrophobic (α-pinene) volatile organic compounds (VOC's) biofilters were discussed. The model proposed here is based on the mass transfer in air–biofilm interface and chemical oxidation in the air stream phase. An approximate analytical expression of concentration profiles of methanol and α-pinene in air stream and biofilm phase have been derived using the Adomian decomposition method (ADM) for all possible values of parameters. Furthermore, in this work, the numerical simulation of the problem is also reported using the Matlab program to investigate the dynamics of the system. Graphical results are presented and discussed quantitatively to illustrate the solution. Good agreement between the analytical and numerical data is noted.
1 Introduction
Different cleaning technologies of gaseous effluents have been developed. Among these technologies, biological methods are increasingly applied for the treatment of air polluted by a wide variety of pollutants. Biofilteration is certainly the most commonly used biological gas treatment technology. Biofilteration involves naturally occurring microorganisms immobilized in the form of biofilm on a porous medium such as peat, soil, compost, synthetic substances or their combination.
The medium provides to the microorganisms a hospitable environment in terms of oxygen, temperature, moisture, nutrients and pH. As the polluted airstream passes through the filter-bed, pollutants are transferred from the vapor phase to the biofilm developing on the packing particles [Citation1,Citation2].
Recently Li et al. [Citation3] as well as other research groups [Citation4–Citation[5]Citation[6]Citation[7]Citation[8]Citation[9]Citation10] have investigated emissions of VOCs into the atmosphere. Currently, biological control processes have become an established technology for air pollution control. Biological control processes have many advantages over traditional methods such as lower operating fees and less secondary pollution, which is rather true for the removal of readily biodegradable VOCs at low concentrations, so these processes are investigated largely and widely. Bioreactors for VOC removal can be classified as biofilters, bio scrubbers, biotrickling filters, or rotating drum biofilters, and choice of reactors should be based on many factors including the characteristics of the target VOCs [Citation11–Citation[12]Citation15].
In order to control the emission of volatile organic compounds (VOC) like methanol, α-pinene, etc. from industries, biofilters are being used nowadays instead of chemical complex absorption method [Citation16–Citation[17]Citation[18]Citation[19]Citation20]. Biofilters offer two major advantages to an energy-starved country like India. A mathematical model is describing the dynamic physical and biological processes occurring in a packed trickle-bed air biofilters to analyze the relationship between biofilter performance and biomass accumulation in the reactor [Citation4].
For the treatment of mixed VOCs [Citation21–Citation[22]Citation23], the presence of methanol and α-pinene in the air stream significantly influenced the removal of pollutants. The removal capacity for methanol and α-pinene per unit volume of the bed decreased linearly with increasing loading rates of methanol and α-pinene. The presence of this easily biodegradable compound suppressed the growth of the methanol and α-pinene degrading microbial community, thereby decreasing methanol and α-pinene removal capacity of the biofilters. Some researchers have studied the biofitration of pure methanol [Citation24–Citation[25]Citation26] and pure α-pinene [Citation27,Citation28].
Recently, few researchers have studied the biofitration of mixtures of pure methanol and pure α-pinene. Also a few researchers have tried to examine the treatment of mixtures of hydrophobic and hydrophilic VOCs and to understand the interactions between these compounds despite the fact that this situation exists in larger amount of air emissions. Mohseni and Allen [Citation16] developed a mathematical model for methanol and α-pinene removal in VOC's biofilteration. Lim et al. [Citation29] developed the steady state solution of biofilter model only for the limiting cases (first order and zero order kinetics). Also Lim et al. [Citation30,Citation31] obtained the non-steady solution of biofilter model using numerical methods. Recently some authors [Citation32,Citation33] solved the non-linear problems using fractional reduced differential transform method (FRDTM). To the best of our knowledge, to date, a rigorous analytical expression of concentrations of substrate in the biofilm phase and air phase has been reported. The purpose of this communication is to derive approximate analytical expressions for the concentrations in both the phases using the Adomian decomposition method [Citation34–Citation[35]Citation40].
2 Mathematical modeling of the boundary value problem
The mathematical model relating the biofiltration of blends of hydrophilic and hydrophobic VOCs is based on the biophysical model proposed by Mohseni and Allen [Citation16]. It includes two main processes of diffusion of the compounds methanol and α-pinene through the biofilm and their degradation in the biofilm. Fig. 1 illustrates a schematic diagram of a single particle, in the biofilter, covered with a uniform layer of biofilm in which the simultaneous biodegradation of methanol and α-pinene takes place. The experimental setup for the biofilteration of this organic compound is given in Fig. 2.

2.1 Mass balance in the biofilm phase
The removal of methanol and α-pinene in the biofilm at steady state is described by the following system of non-linear differential equations (Mohseni and Allen [Citation16]):(1)
(1)
(2)
(2) where Sm and Sprepresent the concentration of methanol and α-pinene respectively.
are maximum specific growth rate, half saturation constant, yield coefficient, effective diffusion coefficient and the distance respectively. Subscripts m and p represent methanol and α-pinene respectively. The dry cell density in the biofilm X represents the overall population of microorganisms that consist of methanol and α-pinene degraders. The coefficient for the effect of methanol on α-pinene biodegradation is defined as follows:
(3)
(3) where Ki and Cm are the inhibition constant and the concentration of methanol in the air phase respectively. The boundary conditions are
(4)
(4)
(5)
(5)
2.2 Mass balance in gas phase
The concentrations of methanol and α-pinene in the air, along the biofilter column, are described by(6)
(6)
(7)
(7) where Cm and Cp represent the concentration of methanol and α- pinene in the air phase.
are the superficial gas velocity, biofilm surface area, effective diffusivity of methanol, effective diffusivity of α-pinene and position along the height of the biofilters respectively. The corresponding initial conditions are
(8)
(8) where the subscript i represents the concentration of the VOCs at the biofilters inlet.
2.3 Dimensionless mass balance equation in the biofilm phase
The non-linear differential Eqs. Equation(1)(1)
(1) and Equation(2)
(2)
(2) are made dimensionless by defining the following dimensionless parameters:
(9)
(9)
(10)
(10)
Using the above dimensionless variables, Eqs. Equation(1)(1)
(1) and Equation(2)
(2)
(2) reduce to the following dimensionless form:
(11)
(11)
(12)
(12)
The corresponding boundary conditions for the above Eqs. Equation(11)(11)
(11) and Equation(12)
(12)
(12) can be expressed as
(13)
(13)
(14)
(14)
2.4 Dimensionless mass balance in the gas phase
The differential Eqs. Equation(6)(6)
(6) and Equation(7)
(7)
(7) are made dimensionless by defining the following parameters:
(15)
(15)
Using Eq. Equation(15)(15)
(15) , Eqs. Equation(6)
(6)
(6) and Equation(7)
(7)
(7) can be expressed in the dimensionless form as follows:
(16)
(16)
(17)
(17)
The corresponding initial conditions for the above Eqs. Equation(16)(16)
(16) and Equation(17)
(17)
(17) can be expressed as
(18)
(18)
3 Analytical expression for the concentration of methanol and α-pinene using the Adomian decomposition method (ADM)
In recent years, many authors have applied the ADM [Citation35–Citation[36]Citation[37]Citation[38]Citation[39]Citation40] to various problems and demonstrated the efficiency of the ADM for handling non-linear and solving various chemistry and engineering problems. Using ADM (refer to Appendix A), we can obtain the concentration of methanol and α-pinene in the biofilm phase (see Appendix B) as follows:(19)
(19)
(20)
(20)
Also solving Eqs. (6–7) and (16–17) using the analytical method, we can obtain the concentration of methanol and α-pinene in the air phase.(21)
(21)
(22)
(22)
4 Analytical expression for the concentrations of methanol and α-pinene for unsaturated (first order) kinetics
Now we consider the limiting case where the substrate concentrations of methanol and α-pinene in biofilm phase are relatively low. In this case . Eqs. Equation(1)
(1)
(1) and Equation(2)
(2)
(2) now reduce to the following form.
(23)
(23)
(24)
(24)
The analytical expression for concentrations of methanol and α-pinene in the biofilm phase becomes(25)
(25)
(26)
(26)
Using Eqs. (6–7) and (25–26) we obtain the analytical expression of the concentrations of methanol and α-pinene in the air phase.(27)
(27)
(28)
(28)
5 Analytical solutions for the concentrations of methanol and α-pinene for saturated (zero order) kinetics
Next we consider the limiting case where the substrate concentrations of methanol and α-pinene in biofilm phase is relatively high. In this case and Eqs. Equation(1)
(1)
(1) and Equation(2)
(2)
(2) reduce to the following form.
(29)
(29)
(30)
(30)
Then the analytical expressions for concentration of methanol and α-pinene in the biofilm phase are as follows:(31)
(31)
(32)
(32)
Using Eqs. (6–7) and (31–32), we obtain the analytical expression of the concentrations of methanol and α-pinene in the air phase.(33)
(33)
(34)
(34)
6 Removal ratio of methanol and α-pinene
The percentage of the methanol removal ratio is(35)
(35) where
are the initial (before treatment) and the final (after treatment) concentrations of methanol in the air phase, respectively. The percentage of the α-pinene removal ratio is
(36)
(36) where
and
are the initial (before treatment) and the final (after treatment) concentrations of α-pinene in the air phase respectively.
7 Numerical simulation
In order to investigate the accuracy of the ADM solution with a finite number of terms, the system of differential equations was solved numerically. To show the efficiency of the present method, our analytical results are compared with numerical results graphically. The analytical solution of the concentrations of methanol and α-pinene in air phase and biofilm phase are compared with simulation results in Figs. 3–Fig. 4Fig. 56. Upon comparison, it gives a satisfactory agreement for all values of the dimensionless parameters . The detailed Matlab program for numerical simulation is provided in Appendices C and D.
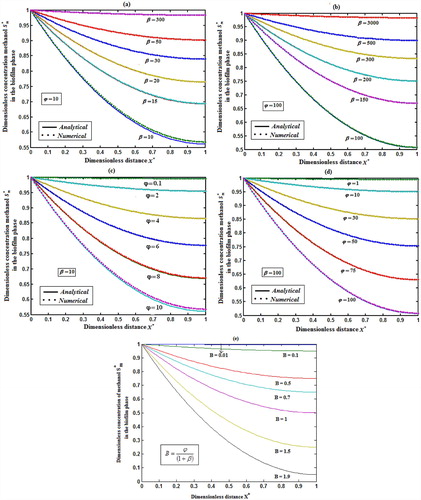
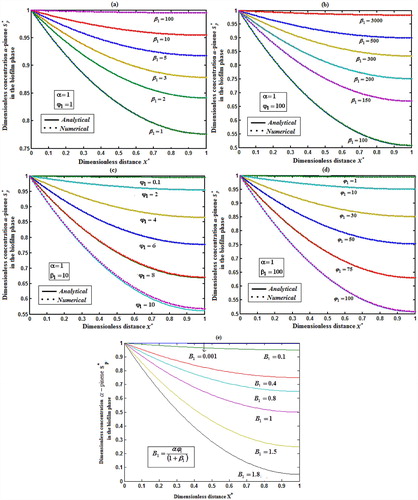
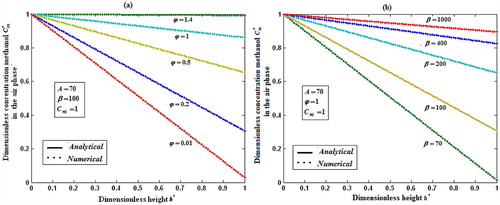
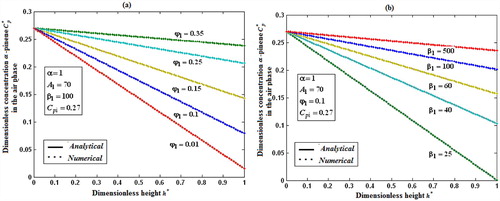
8 Results and discussion
Eqs. (19–22) represent the simple and new analytical expression of the concentrations of methanol and α-pinene in biofilm phase and in the air phase
respectively. The concentrations of methanol and α-pinene in the biofilm phase and the air phase depend upon the parameters φ and β. The variation in the dimensionless variable φ can be achieved by varying either the thickness or dry cell density of the biofilm. The parameter β depends upon the initial concentration and half saturation constant.
Fig. 3 represents the concentration of methanol in the biofilm phase versus dimensionless distance X* for different values of φ and β. From Fig. 3a, b, it is inferred that the concentration of methanol increases when the initial concentration of methanol β increases for the fixed values of φ. For large value of dimensionless parameter β, the concentration of methanol remains constant. In Fig. 3c, d, we present the concentration of methanol in the biofilm phase for various values of φ and for some fixed values of β. Maximum specific growth rate of methanol biodegradation φ decreases the concentration of methanol slowly and reaches the constant level. The minimum value of
are
and
respectively.
Fig. 4 exhibits the concentration of α-pinene in the biofilm phase versus dimensionless distance X* for different values of
. From Fig. 4a, b, it is inferred that the concentration of α-pinene increases when the initial concentration of α-pinene
increases for the fixed values of dry cell density φ1. For large value of β1, the concentration of α-pinene is uniform. In Fig. 4c, d, we show that the concentration of α-pinene in the biofilm phase for various values of cell density φ1 and for some fixed values of dimensionless parameter β1. From this figure, we conclude that the concentration of α-pinene
increases when thickness of the film decreases. The concentration of α-pinene is equal to one
when
.
Fig. 5a, b shows the dimensionless concentration of methanol versus dimensionless height h*. From Fig. 5a, it is described that the concentration of methanol slowly reaches the constant when the biofilm thickness or φ increases. In Fig. 5b, it is labeled that the concentration of methanol decreases when half saturation constant of methanol β decreases for the fixed value of other parameter.
Fig. 6a, b demonstrates the concentration of α-pinene in the air phase versus dimensionless height h*. From Fig. 6a, it is inferred that the concentration of α-pinene slowly reaches a constant level when the diffusion coefficient φ1 increases for the fixed value of other parameter. In Fig. 6b, it is labeled that the concentration of α-pinene attains the steady state values when the initial concentration of α-pinene
increases.
Fig. 7 shows the profile of dimension concentration of methanol and α-pinene in the air phase versus height h for some fixed value of the parameters. From these figures it is inferred that the concentration is linearly proportional to the height of the biofilter. And also the concentration of decrease when the height of the biofilter increases. Fig. 8a, b illustrates the removal ratio of methanol and α-pinene in the air phase. From this figure it is observed that the removal ratio is directly proportional to the inlet loading. Our analytical results are compared with the experimental result and excellent agreement is noted.
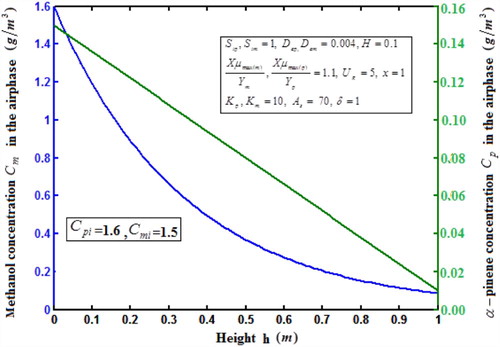
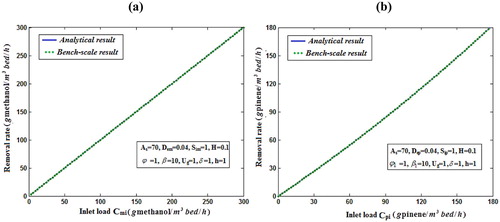
9 Conclusion
In this paper, the non-linear differential equations in biofiltration model have been solved analytically. Approximate analytical expressions pertaining to the concentrations of methanol and α-pinene in the biofilm phase for all the values of parameters are obtained using the Adomian decomposition method. This solution of the concentrations of methanol and α-pinene in the biofilm phase and air phase are compared with the numerical simulation results. This model is also validated using experimental results. These analytical results provide a good understanding of the system and the optimization of the parameters in biofiltration model.
Acknowledgements
This work was supported by the Department of Science and Technology (DST) (No. SB/S1/PC-50/2012). The authors are thankful to The Principal, The Madura College, Madurai and The Secretary, Madura College Board, Madurai for their encouragement.
References
- S.P.P.OttengrafBiological system for waste gas eliminationTibtech51987132136
- A.H.WaniR.M.R.BranionA.K.LauBiofiltration: a promising and cost effective control technology for odours VOC's and air toxicJ Environ Sci Health A32199720272055
- LiG.W.HuH.Y.HaoJ.M.ZhangH.Q.Use of biological activated carbon to degrade benzene and toluene in a biofilterEnviron Sci23520021318
- SunY.M.QuanX.YangF.L.ChenJ.W.LinQ.Y.Effect of organic gas components on biofitration and microbial density in biofilterJ Appl Environ Biol11120058285
- YangC.P.M.T.SuidanZhuX.Q.B.J.KimComparison of single-layer and multi-layer rotating drum biofilters for VOC removalEnviron Prog22220038794
- YangC.P.M.T.SuidanZhuX.Q.B.J.KimBiomass accumulation patterns for removing volatile organic compounds in rotating drum biofiltersWat Sci Technol48820038996
- YangC.P.M.T.SuidanZhuX.Q.B.J.KimRemoval of a volatile organic compound in a hybrid rotating drum biofilterJ Environ Eng13032004282291
- YangC.P.ChenH.ZengG.YuG.LuoS.Biomass accumulation and control strategies in gas biofitrationBiotechnol Adv282010531540
- M.M.TonekaboniBiofitration of hydrophilic and hydrophobic volatile organic compounds using wood-based media1998University of TorontoToronto
- T.P.KumarRahulM.A.KumarB.ChandrajitBiofiltration of volatile organic compounds (VOCs) – an overviewRes J Chem Sci1820118392
- YangC.P.Rotating drum biofitration2004Department of Civil and Environmental Engineering, University of CincinnatiCincinnati, OH
- YangC.P.ChenH.ZengG.M.QuW.ZhongY.Y.ZhuX.Q.et alModeling biodegradation of toluene in rotating drum biofiltersWater Sci Technol5492006137144
- YangC.P.ChenH.ZengG.M.ZhuX.Q.M.T.SuidanPerformances of rotating drum biofilter for VOC removal at high organic loadingsJ Environ Sci2032008285290
- YangC.P.M.T.SuidanZhuX.Q.B.J.KimZengG.M.Effect of gas empty bed contact time on performances of various types of rotating drum biofilters for removal of VOCsWater Res4214200836413650
- ZhuX.Q.M.T.SuidanA.J.PrudenYangC.C.AlonsoB.J.Kimet alThe effect of substrate Henry's constant on biofilters performanceJ Air Waste Manage Assoc5442004409418
- M.MohseniD.G.AllenBiofiltration of mixtures of hydrophilic and hydrophobic volatile organic compoundsChem Eng Sci559200015451558
- E.M.Ramirez-LopezJ.Corona-HernandezF.J.Avelar-GonzalezF.OmilF.ThalassoBiofiltration of methanol in an organic biofilter using peanut shells as mediumBioresour Technol10120108791
- E.R.ReneM.E.LopezM.C.VeigaD.C.KennesSteady- and transient-state operation of a two-stage bioreactor for the treatment of a gaseous mixture of hydrogen sulphide, methanol and α-pineneJ Chem Technol Biotechnol8532010336348
- S.DhamwichukornG.T.Klein HeinzS.T.BagleyThermophilic biofiltration of methanol and alpha-pineneJ Ind Microbiol Biotechnol2632001127133
- A.M.SiddiquiM.HameedB.M.SiddiquiQ.K.GhoriUse of Adomian decomposition method in the study of parallel plate flow of a third grade fluidCommun Nonlinear Sci Numer Simul15201023882399
- E.R.ReneJinY.M.C.VeigaC.KennesTwo-stage gas-phase bioreactor for the combined removal of hydrogen sulphide, methanol and alpha-pineneEnviron Technol3012200912611272
- M.MohseniD.G.AllenTransient performance of biofilters treating mixtures of hydrophilic and hydrophobic volatile organic compoundsJ Air Waste Manag Assoc4912201114341441
- A.BerenjianN.ChanH.J.MalmiriVolatile organic compounds removal methods: a reviewAm J Biochem Biotechnol842012220229
- Z.ShareefdeenB.C.BaltzisY.S.OhR.BarthaBiofiltration of methanol vaporBiotechnol Bioeng411993512524
- R.PremkumarN.KrishnamohanRemoval of methanol from waste gas using biofiltrationJ Appl Sci Res611201018981907
- S.ArriagaM.A.SerranoA.P.Barba de la RosaMethanol vapor biofiltration coupled with continuous production of recombinant endochitinase Ech42 by Pichia PastorisProcess Biochem4712201223112316
- M.MohseniBiofiltration of alpha-pinene and its application to the treatment of pulp and paper air emissionsTappi J8181998205211
- I.O.CabezaR.LopezI.GiraldezR.M.StuetzM.J.DíazBiofiltration of α-pinene vapours using municipal solid waste (MSW) – pruning residues (P) composts as packing materialsChem Eng J2332013149158
- K.H.LimWaste air treatment with a biofilter for the case of excess adsorption capacityJ Chem Eng Jpn3462001766775
- K.H.LimE.J.LeeBiofilter modeling for waste air treatment: comparisons of inherent characteristics of biofilter modelsKorean J Chem Eng2022003315327
- E.J.LeeK.H.LimA dynamic adsorption model for the gas-phase biofilters treating ethanol: prediction and validationKorean J Chem Eng2910201213731381
- V.K.SrivastavaS.KumarM.K.AwasthiB.K.SinghTwo-dimensional time fractional-order biological population model and its analytical solutionEgypt J Basic Appl Sci120147176
- V.K.SrivastavaM.K.AwasthiS.KumarAnalytical approximations of two and three dimensional time fractional telegraphic equation by reduced differential transform methodEgypt J Basic Appl Sci1120146066
- J.BiazarE.BabolianR.IslamSolution of the system of ordinary differential equations by Adomian decomposition methodAppl Math Comput1472004713719
- A.R.AmaniJ.SadeghiAdomian decomposition method and two coupled scalar fields The International Conference Differential Geometry – Dynamical Systems Bucharest-Romania 2008; 11–18. Balkan Society of Geometers, Geometry Balkan Press2008
- G.AdomianR.RachAnalytic solution of non-linear boundary-value problems in several dimensions by decompositionJ Math Anal Appl1741993118137
- DuanJ.S.R.RachA new modification of the Adomian decomposition method for solving boundary value problems for higher order nonlinear differential equationsAppl Math Comput218201140904118
- R.MuthuramalingamS.KumarR.LakshmananAnalytical expressions for the concentration of nitric oxide removal in the gas and biofilm phase in a biotrickling filterJ Assoc Arab Univ Basic Appl Sci1820151928
- L.RajendranM.SivasankariAnalytical expression of concentration of VOC and oxygen in steady state in biofiltration modelAppl Math41201212
- S.MuthukaruppanR.SenthamaraiL.RajendranModeling of immobilized glucoamylase kinetics by flow calorimetryInt J Electrochem Sci7201291229137
Appendix A
Basic concepts of the Adomian decomposition method (ADM)
Consider the non-linear differential equation(A.1)
(A.1) with boundary conditions
(A.2)
(A.2) where N(y) is a non-linear function, g(x) is the given function and A, B and b are given constants. We propose the new differential operator, as below
(A.3)
(A.3)
So, Eq. Equation(A.1)(A.1)
(A.1) can be written as
(A.4)
(A.4)
The inverse operator is therefore considered as a two-fold integral operator (Duan and Rach [Citation37]), as below
(A.5)
(A.5)
Applying the inverse operator on both sides of Eq. Equation(A.4)
(A.4)
(A.4) yields
(A.6)
(A.6)
Using the boundary conditions Eq. Equation(A.2)(A.2)
(A.2) , Eq. Equation(A.6)
(A.6)
(A.6) becomes
(A.7)
(A.7)
The Adomian decomposition method introduces the solution y(x) and the non-linear function N(y) by infinite series(A.8)
(A.8) and
(A.9)
(A.9) where the components yn(x) of the solution y(x) will be determined recurrently and the Adomian polynomials An of N(y) are evaluated using the formula
(A.10)
(A.10) which gives
(A.11)
(A.11)
By substituting Eqs. Equation(A.8)(A.8)
(A.8) and Equation(A.9)
(A.9)
(A.9) in Eq. Equation(A.7)
(A.7)
(A.7) gives
(A.12)
(A.12)
Then equating the terms in the linear system of Eq. Equation(A.11)(A.11)
(A.11) gives the recurrent relation
(A.13)
(A.13) which gives
(A.14)
(A.14)
From Eqs. Equation(A.11)(A.11)
(A.11) and Equation(A.14)
(A.14)
(A.14) , we can determine the components yn(x), and hence the series solution of yn(x) in Eq. Equation(A.7)
(A.7)
(A.7) can be immediately obtained.
Appendix B
Analytical solution of Eqs. Equation(11)
(11)
(11) and Equation(12)
(12)
(12)
In this appendix, we have derived the solution of Eqs. Equation(11)(11)
(11) and Equation(12)
(12)
(12) using the Adomian decomposition method. Eq. Equation(11)
(11)
(11) can be written with the operator form
(B.1)
(B.1)
(B.2)
(B.2)
Applying the inverse operator on both sides of Eq. Equation(B.1)
(B.1)
(B.1) yields
(B.3)
(B.3) Where
and
. We let,
(B.4)
(B.4)
(B.5)
(B.5)
In view of Eqs. Equation(B.4)(B.4)
(B.4) and Equation(B.5)
(B.5)
(B.5) , Eq. Equation(B.3)
(B.3)
(B.3) gives
(B.6)
(B.6)
We identify the zeroth component as(B.7)
(B.7) and the remaining components as the recurrence relation
(B.8)
(B.8) where An are the Adomian polynomials of
. We can find the first few An as follows:
(B.9)
(B.9)
(B.10)
(B.10)
The remaining polynomials can be generated easily, and so,(B.11)
(B.11)
(B.12)
(B.12)
Adding Equation(B.11)(B.11)
(B.11) and Equation(B.12)
(B.12)
(B.12) , we get Eq. Equation(19)
(19)
(19) in the text. Similarly, we can apply the above method to find the solution of Eq. Equation(12)
(12)
(12) . Higher order iteration will be considered to improve the accuracy of the results.
Appendix C
Matlab program for the numerical solution of Eqs. Equation(11)
(11)
(11) and Equation(12)
(12)
(12)
Appendix D
Matlab program for the numerical solution of Eqs. Equation(16)
(16)
(16) and Equation(17)
(17)
(17)
Appendix E
Nomenclature
Table