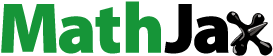
Abstract
The muskmelon peel, an abundant common food waste material, has been gainfully utilized for the production of biochar. The biosorptive characteristics of muskmelon peel biochar towards copper(II) and zinc(II) from water are investigated. The biochar is characterized by infrared spectroscopy (FT-IR), scanning electron microscopy (SEM) and energy dispersive X-ray (EDX) studies. The various process parameters for the removal of copper(II) and zinc(II) using biochar are optimized. The maximum biosorption of Cu(II) and Zn(II) is attained at pH 7. Maximum Langmuir adsorption capacity (qm) are 78.74 for copper(II) and 72.99 mg/g for zinc(II) at 303 K. Langmuir isotherm is found to best fit the equilibrium data indicating homogeneous adsorption of metal ions onto the biochar surface. The pseudo-second order kinetic model describes the data best indicating adsorption of one molecule of metal ions onto two surface sites. Thermodynamic parameters suggest the adsorption process to be spontaneous and exothermic. Both liquid-film and intra-particle diffusions controll the overall kinetics of the adsorption process. Biochar proved to be an inexpensive and efficient adsorbent for the removal of titled metals from liquid phase.
Introduction
The growing industrialization has resulted in an increased influx of industrial effluents containing heavy metals into the aqueous streams. Electroplating and metal surface treatment processes produce large quantity of wastewater containing heavy metals, which include copper(II) and zinc(II). The presence of copper(II) and zinc(II) in aquatic environment, even at low concentrations, is potentially harmful to human health. Copper(II), beyond the maximum concentration limit (0.25 mg/L) can cause liver damage, Wilson disease, insomnia, and depression, while a person exposed to zinc(II) (exposure limit, 0.80 mg/L) is likely to suffer from neurological disorders, lethargy, and thirst. Considering the toxicity and harmful effects of these metals, it is essential to pretreat metals bearing wastewater before discharging into aquatic system.
Several techniques available for heavy metals removal from wastewater such as reverse osmosis [Citation1], electrocoagulation [Citation2], ultrafiltration [Citation3], dialysis/electrodialysis [Citation4], and solvent extraction [Citation5] are either costly or less effective due to their own limitations. However, the treatment of aqueous effluents using adsorption of metals onto low cost adsorbents [Citation6Citation[7]Citation[8]Citation[9]Citation[10]Citation[11]–Citation12] is regarded as an efficient and economical alternative. Biosorption using many agricultural biomass has attracted recent attention because of various functional groups available on the surface of biosorbents for the removal of heavy metals/dyes even at very low concentration from aqueous system [Citation13,Citation14] .
In recent past, biochar derived from many agricultural wastes has been used as an effective, efficient, and low cost adsorbent for the removal of heavy metals and organic contaminants from water and soil [Citation15] because of its highly porous structure, large surface area, high functional groups and mineral components [Citation16]. Biochars are mainly applied for enhancing soil fertility and crops productivity, reducing emission of green house gases, waste management and energy production [Citation17,Citation18] . Biochars are usually prepared by the pyrolysis of carbon rich agro-solid waste materials. Various agro-wastes have been used as the precursor for the preparation of biochars and utilized for the removal of heavy metals from water, which include corn straw [Citation19], rice straw [Citation20] straws of peanut, soybean and canola [Citation21] sugar beet tailing [Citation22,Citation23] , oak wood and oak bark [Citation24], rice husks, olive pomace, orange waste and compost [Citation25].
The surface properties, yield and adsorption capacity of biochar mainly depend upon the type of precursor (lignocellulosic content) and pyrolytic conditions [Citation26,Citation27] . Slow pyrolysis of biomass at low temperature (400–500 °C) with slow heating rate (0.1–1 °C/s) at large resident time 5–30 min favors high yield of biochar. In intermediate pyrolysis with pyrolytic temperature (500–650 °C), heating rate (0.1–10 °C/min) and resident time 300–1000 s, 15–25% biochar is obtained. Similarly, the pyrolysis at higher temperature (850–1250 °C), heating rate (10–200 °C/s) and small residence time (1–10 s) generally yields 15–25% of biochar. It has been reported [Citation28] that slow pyrolysis generally results in biochars with surface area of 1.8–56 m2/g), while during fast pyrolysis the biochars usually possess surface area between 7 and 50 m2/g [Citation29].
Muskmelon (Cucumis melo L.) fruit belonging to Cucurbitaceae family, commonly known as kharbuja in India, is abundantly available during summers. The edible portion of the ripe fruit is consumed while the peel is discarded as a biowaste. The major constituent of muskmelon peel is cellulose, protein and polysaccharides (pectic acid) with –OH and –COOH functional groups, which may bind with metal ions. Recently, the muskmelon peel chemically modified with calcium hydroxide has been studied for the removal of Pb(II) from aqueous solution [Citation30] with adsorption capacity of 167.8 mg/g. However, muskmelon peel has not been utilized as a precursor for production of its biochar.
In this study, various operating conditions in batch process such as biochar dose, contact time, initial Cu(II) and Zn(II) concentration, initial solution pH and temperature are optimized. The biosorption efficiency of muskmelon (Cucumis melo L.) peel biochar for copper and zinc from water has been assessed. The isotherm, kinetics and thermodynamics of the biosorption process has been evaluated from the experimental data. The surface properties of adsorbent are examined by FTIR and SEM analysis to evaluate the surface functionality and morphology of the biosorbent.
Experimental
Materials
Muskmelon peel was collected from the fruit juice and shake shop (Jamia Nagar, New Delhi, India). CuSO4·5H2O, ZnSO4·7H2O, NaOH and HCl was obtained from Merck, India.
Preparation of muskmelon peel biochar
The musk melon peel was washed with distilled water to remove adhering dust particles/impurities, and dried in oven at 333 K. The dried biomass was grinded in a mechanical grinder to a particle size of 1–3 mm. For the preparation of biochar, muskmelon peel (200 g) was pyrolysed at 873 K in a Muffle furnace (Matrix Scientific, India) with heating rate of 278 K/min under inert atmosphere (N2 gas) for 1 h, and cooled to room temperature. The black solid char was crushed with mortar and pestle, washed with distilled water, sieved to less than 75 µm particle size, and stored in an airtight reagent bottle.
Preparation of adsorbate solution
The stock solution (250 mg/L) of copper sulphate pentahydrate [CuSO4·5H2O] and zinc sulphate heptahydrate [ZnSO4·7H2O] were prepared by dissolving 0.982 g and 1.099 g of the respective salt in double distilled water (1 L). The required solutions were prepared by diluting the stock solution with double distilled water.
Characterization
The surface morphology and elemental composition of biochar was evaluated by using a Carl Zeiss (Sigma 5.05, Germany) scanning electron microscopy conjugated with BRUKER energy dispersive X-ray analyzer (EDX). FTIR spectra (4000–400 cm−1) of the biochar were run on a Perkin-Elmer spectrometer (model BX spectrum, United States).
Batch equilibrium studies
Batch adsorption experiments were carried out in a series of 50 mL erlenmeyer flasks. Each flask was filled with 25 mL metal solution of desired concentration (50 mg/L) and adjusted to the desired pH. A known amount (0.02 g) of biochar was added to each flask and kept in isothermal shaker (303 K) at 200 rpm until equilibrium was reached. The supernatant of reaction mixture was separated by centrifugation. The equilibrium concentration of metal ion was determined by AAS (Perkin Elmer, AAnalyst 200). The equilibrium adsorption capacity (qe, mg/g) and% removal was determined by Eqs. Equation(1)(1)
(1) and Equation(2)
(2)
(2) :
(1)
(1)
(2)
(2) where C and Ce is the initial and equilibrium concentration of metal ions solution (mg/L), V is the volume of metal solution (L), and m is the mass of adsorbent (g).
All experiments were carried out in triplicate and average values are reported.
Results and discussion
Characterization of adsorbent
FTIR studies
The FTIR spectral studies were performed to elucidate the active surface functional groups, which may provide binding sites for the metal ions. A strong band observed at 3405 cm−1 in the IR spectrum of the muskmeolon peel may be assigned to O–H stretching vibration of inter and intramolecular hydrogen bonded phenols and carboxylic acids. The bands at 2930 and 2850 cm−1 are ascribed to aliphatic C–H of carboxylic acid. The strong bands at 1660 and 1410 cm−1 are ascribed to C=O antisymmetric and symmetric stretching vibration in carboxylate group and C–H in plane bending vibration of lignin. A weak peak at 1540 cm−1 is due to C=C stretching vibration of conjugated aromatic ring of lignin. The peaks at 1250 and 1040 cm−1 may be due to C–OH vibrations of carboxylic acids and alcohol groups of cellulose. The shoulder at 800–400 cm−1 corresponds to C=C–H vibration of benzene ring (a). However, in the biochar, the O–H stretching band, of a decreased intensity, appears at 3400 cm−1, which may be due to cleaving of phenolic groups and dehydration of peel structure. The band observed at 2930 cm−1 in the muskmelon peel shifts to 2920 cm−1, whereas the new weak peaks at 1733 and 1573 cm−1 may be attributed to the formation of carbonate and/or carbonate-carboxyl group during pyrolysis. The broad shoulder at 1170 cm−1 is observed due to the rupture of cellulosic/hemicellulosic groups (b). In the spectra of Cu(II)- and Zn(II)-loaded biochar the O–H stretching band at 3400 cm−1 is shifted to 3440 and 3436 cm−1, while the peak at 1170 cm−1 disappears. Similarly, the peak at 1573 cm−1 is observed at 1600 and 1585 cm−1 after the adsorption of Cu(II) and Zn(II), respectively. The new peaks at 1400 (1390) and 1038 (1050) cm−1 suggest an appreciable interaction of the surface functional groups with Zn(Cu) metal ions ((c) and (d).
SEM and EDX studies
The SEM images (mag. 20,00 KX and 50,00 KX) of raw muskmelon peel exhibit homogeneous and smooth surface with porous structures (a and b). The images of biochar, however, display heterogeneous surface with enlarged porous structures (c and d). The SEM micrographs of metal loaded biochar, however, show that the surface is covered with copper and zinc molecules (e to h). The EDS spectra of biochar and metal loaded biochar is shown in . The main constituents of muskmelon biochar (wt%) are: C (59.28) and O (31.46) with traces of Mg (1.83), P (1.92), K (1.68), and Ca (3.84) (a). After biochar formation, the element wt% are: C (56.43) and O (41.77), Mg (0.40), K (0.79), and Ca (3.84). The P element disappears probably due to heat treatment resulting in the formation of surface voids. In the EDX analyses, after copper and zinc adsorption, new peaks of Cu (1.63 wt%) and Zn (9.50) appear, which indicates the adsorption of these metals on the surface of the biochar ().
Fig. 2 SEM images at different magnifications of raw muskmelon peel (a,b), biochar (c,d), Cu loaded biochar (e,f), and Zn-loaded biochar (g,h).
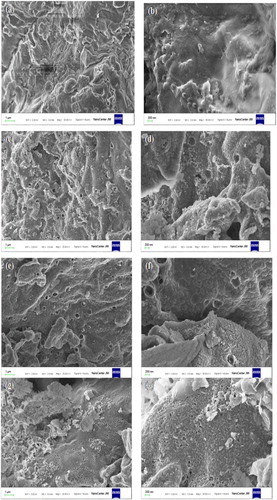
Fig. 3 EDS spectra of raw muskmelon peel (a), biochar (b), Cu-loaded (c), and Zn-loaded (d) biochar.
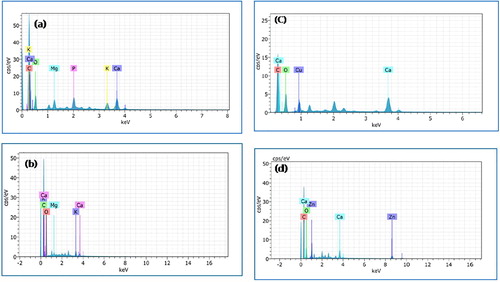
Table 1 Thermodynamic parameters.
Equilibrium adsorption studies
Effect of contact time
The optimum contact time for the removal of Cu(II) and Zn(II) by biochar was determined by varying the contact time between 10 and 140 min at initial metal concentration (50 mg/L), dose (0.8 g/L), pH (7) and 303 K. It was observed () that the percent removal of Cu(II) increases from 44.4 to 90.2 and Zn(II) from 35.4 to 83.5 with increasing contact time, attaining equilibrium at 120 min (90.2 for Cu(II) and 83.5 for Zn(II) (). The initial rapid uptake of metal ions may be ascribed to the presence of large number of vacant sites available for metal ions, and afterwards the remaining free metal ions are difficult to be occupied, because of repulsive forces between the free and adsorbed metal ions.
Effect of initial metal concentrations
Batch experiment is to study the effect of initial metal concentration on the percentage removal of copper(II) and zinc(II) by biochar was carried out at fixed contact time (120 min), dose (0.8 g/L), temperature (303 K) and pH (7) but varying the initial concentration from 30 to 60 mg/L. It is found that the adsorption is dependent on initial metal concentrations with percentage uptake of copper(II) and zinc(II) decreasing from 99 to 89.5 and 96.6 to 83.5, respectively, with increasing initial metal concentrations (). However, the adsorption capacity increases from 37.1 to 70.8 mg/g for copper(II) and 36.2 to 71.4 mg/g for zinc(II). The optimum adsorption takes place at 60 mg/L.
Effect of biochar dose
In this study, the biochar dose was varied from 0.2 to 1.2 g/L at optimized contact time (120 min) and initial metal concentration (50 mg/L). The variation in percentage removal of copper(II) and zinc(II) together with biochar adsorption capacity is depicted in (), which indicates that the percent adsorption of copper(II) and zinc(II) increases from 41.3 to 89. 2 and 52 to 85.2 with increasing dose (), with maximum percentage removal occurring at 0.8 g/L. This might be attributed to the greater availability of the active sites for adsorption or increase in the surface area at higher amount of the adsorbent dose.
Effect of pH
The effect of pH on the removal of Cu(II) and Zn(II) was evaluated at varying pH (2–9) keeping other parameters constant. The amount of uptake (%) increases from 42.0 to 93.3 and 39.5 to 86.0 for copper and zinc, respectively between pH 2 and 9, due to decrease in competition between H3O+ and metal cations (). At pH above pHzpc (6.5), surface attains a negative charge while at pH below pHzpc the surface acquires a positive charge. With increase in the initial solution pH, copper(II) and zinc(II) exist in a variety of species such as Cu2(OH)22+, Cu3(OH)42+, CuOH+, Cu2(OH)3+, Cu(OH)2 and Zn2+, Zn(OH)+, Zn(OH)2 and Zn(OH)3−. Below pH 7, copper(II) and zinc(II) exist predominantly as divalent cations and pH > 7, metal cations may precipitate due to formation of hydroxyl species. The maximum adsorption occurs at pH 7.
Effect of temperature and thermodynamic studies
The effect of temperature on the removal of copper(II) and zinc(II) by biochar was studied at 303, 308 and 313 K, and is shown in . The removal of metals (%) decreases with increase in temperature, indicating the adsorption process to be exothermic. The increase in temperature increases the solubility and decreases the chemical potential of the adsorbate, a controlling factor in the adsorption process, thereby resulting in a decrease in adsorption.
To understand the kinetics of adsorption, the energy and entropy considerations are important. Negative ΔG° indicates the spontaneity of the adsorption process. ΔH° is used to identify the exothermic or endothermic nature of adsorption. A positive value of ΔS° indicates increased randomness of adsorbate molecules on the solid surface than in the solution.
The change in enthalpy (ΔH°) and entropy (ΔS°) were determined from the slope and intercept, respectively of the vant Hoff’s plot of log(qe/Ce) vs 1/T (). The free energy change (ΔG°) was calculated using the Gibb’s equation (Eqs. Equation(3)(3)
(3) and Equation(4)
(4)
(4) ).
(3)
(3)
(4)
(4)
The negative ΔH° values indicate the exothermic nature of the adsorption of copper(II) and zinc(II) onto biochar. The ΔH° values, 32.712 for Cu(II) and 18.039 kJ/mol for Zn(II), are found to be lower than 40 kJ/mol, supporting physisorption [Citation31] (). The negative ΔS° values suggest the probability of favorable adsorption. The decrease in spontaneity (ΔG° becoming less negative) adsorption process with increase in temperature indicates more feasible adsorption at lower temperature.
Equilibrium studies
Equilibrium studies explain the ratio of the amount of adsorbate adsorbed and that remaining in solution at a constant temperature when equilibrium has been achieved. Langmuir [Citation32], Freundlich [Citation33] and Dubinin-Radushkevich [Citation34] isotherm models were applied to the experimental data to determine the best fit model.
Langmuir isotherm model
If the experimental data fits the Langmuir model it indicates an energetically homogeneous adsorption sites and a uniform coverage of adsorbate molecules onto adsorbent surface without any interaction between adsorbed molecules. The linear form of the Langmuir isotherm model is expressed as follows:(5)
(5) where b is the Langmuir constant related to the energy of adsorption, and qm is the maximum adsorption capacity (mg/g).
The monolayer adsorption capacity (qm) and Langmuir constant (b) representing affinity of adsorbate to adsorbent were calculated from the slope and intercept of the Ce/qe vs Ce plot (), and are given in .
Table 2 Various isotherm parameters.
The dimensionless separation factor (RL) [Citation35], can be used to predict the type of adsorption (favorable, linear, unfavourable or irreversible).(6)
(6)
If RL > 1, the adsorption is unfavorable; if 0 < RL < 1, the adsorption is favorable; and if RL = 0, the adsorption is irreversible. The affinity of adsorption of metal ions is in the 0.054–0.075 range for Cu(II) and 0.013–0.015 for Zn(II), which suggests that the adsorption process is highly favorable.
Correlation coefficients (R2) of all the studied isotherm models are compared to find out the best fit model (), which indicate that the adsorption data best follows the Langmuir isotherm model. A comparison of qm values for copper (79.36 mg/g) and zinc (72.99 mg/g) with other reported adsorbents () suggests that muskmelon peel biochar is superior to many of these adsorbents. Higher values of b for copper(II) compared to zinc(II) revealed that the biochar has higher affinity towards copper(II).
Table 3 Adsorption capacity (qm) of reported adsorbents for Cu(II) and Zn(II) uptake.
Freundlich isotherm
This model is based on heterogeneous distribution of adsorption sites on the surface of an adsorbent. The linear form of the Freundlich equation is as follows:(7)
(7) where kf and nf are the Freundlich isotherm constants related to adsorption capacity and adsorption intensity, respectively. The Freundlich parameters, kf and nf were obtained from the slope and the intercept of the log qe vs log Ce plots (). The values of kf and nf along with R2 are shown in . The lower R2 values indicate that the adsorption does not follow Freundlich model. The values of nf < 1 suggest more favorable physical adsorption.
Dubinin–Radushkevich (D–R) isotherm
The D–R isotherm model assumes multilayer adsorption, which generally involves weak van der Waals forces, indicative of physical adsorption process. The model equation is given as follows:(8)
(8) where qD is the maximum adsorption capacity (mg/g), β is the activity coefficient related to mean adsorption energy (mol2/kJ2) and ε is the Polanyi potential.
The values of qD and β were calculated from the slope and intercept of lnqe vs ε2 plot (), while that of ε was calculated using Eq. Equation(9)(9)
(9) .
(9)
(9) where R is the gas constant in kJ/(mol/K) and T is the temperature (K). The mean free energy of adsorption (E) was estimated using Eq. Equation(10)
(10)
(10) .
(10)
(10)
The values of E (1.0–1.12 for Cu(II) and 2.23–3.53 kJ/mol for Zn(II)) are found in the range 0–8 kJ/mol, indicating the adsorption process to be physical in nature. The values of R2 (0.897–0.902 for Cu(II) and 0.763–0.804 for Zn(II), however, suggest a poor fit of the model.
Kinetic studies
Pseudo-first order and pseudo-second order
The dynamics of the adsorption was investigated using Lagergren’s pseudo-first order [Citation55] and pseudo-second order equations [Citation56]. The pseudo-first order model and pseudo-second order model can be expressed as:(11)
(11)
(12)
(12) where qt (mg/g), is the amounts of metal ions adsorbed at time t, k1 and k2 is the pseudo-first-order (1/min) and pseudo-second-order rate constant (g/mg/min).
The k1 and k2 were calculated from the intercept of the corresponding plots of log(qe − qt) vs t, () and t/q vs t (), and are tabulated in along with correlation coefficients, qe(calc) and qe(exp) values. The higher R2 values and close agreement between qe(calc) and qe(exp) values for the pseudo-second order rate equation suggest the suitability of the model indicating adsorption of one dye molecule onto two surface sites.
Table 4 Pseudo-first order and pseudo-second order model parameters.
Liquid–film and intra–particle diffusions
The adsorption rate constant for liquid–film diffusion, Kfd (1/min) and the intra–particle diffusion rate constant, ki (mg/g/min1/2) were calculated from the slope of the corresponding plots of ln(1 − F) vs t [Citation57] and qt vs t0.5 [Citation58] as:(13)
(13)
(14)
(14) where kfd (1/min) is the film-diffusion rate constant, and F is the fractional attainment of equilibrium (F = qt/qe) at time t, ki (mg/g min0.5) is intra-particle diffusion rate constant, Ci is a constant (mg/g) which gives an idea about the thickness of the boundary layer on the adsorbent surface.
The liquid-film diffusion plots are linear (), but do not pass through the origin so it may not be the only controlling factor in determining the kinetics of the process. The intra-particle diffusion plots () have the three steps of adsorption, i) an initial curved portion attributed to the bulk diffusion ii), a linear portion to the intra-particle diffusion, and iii) a plateau due to the equilibrium. The magnitudes of kfd, ki and the corresponding regression coefficients are listed in . The ki values indicate substantial diffusion of copper(II) and zinc(II). It may be inferred that both the liquid-film and intra-particle diffusion are be controlling the adsorption kinetics.
Table 5 Liquid-film and intra-particle diffusion model parameters.
Conclusions
The process parameters for removal of copper and zinc using biochar are optimized. Maximum Langmuir adsorption capacity (qm) are 78.74 for copper(II) and 72.99 mg/g for zinc(II) at 303 K. The values of RL between zero and unity supported favorable adsorption. Langmuir isotherm is found to best fit the equilibrium data indicating homogeneous adsorption onto the biochar surface. The pseudo-second order kinetic model describes the data best indicating adsorption of one molecule of metal ions onto two surface sites. Thermodynamic parameters suggest the adsorption process to be spontaneous and exothermic. Both liquid-film and intra-particle diffusions controll the overall kinetics of the adsorption process. Biochar proved to be an inexpensive and efficient adsorbent for the removal of titled metals from aqueous solution.
References
- T.A.KurniawanG.Y.ChanW.H.LoS.BabelPhysico–chemical treatment techniques for wastewater laden with heavy metalsChem Eng J118120068398
- I.AliT.A.KhanM.AsimRemoval of arsenate from groundwater by electrocoagulation methodEnviron Sci Poll Res195201216681676
- G.GhoshP.K.BhattacharyaHexavalent chromium ion removal through micellar enhanced ultrafiltrationChem Eng J119120064553
- I.A.KhattabM.F.ShaffeiN.A.ShaabanH.S.HusseinS.A.El-RehimElectrochemical removal of copper ions from dilute solutions using packed bed electrode. Part IIEgypt J Petrol2212013205210
- M.ČernáUse of solvent extraction for the removal of heavy metals from liquid wastesEnviron Monit Assess3421995151162
- T.A.KhanM.NazirE.A.KhanMagnetically modified multiwalled carbon nanotubes for the adsorption of bismarck brown R and Cd(II) from aqueous solution: batch and column studiesDesalination Water Treat1120151937419390
- T.A.KhanM.NazirE.A.KhanU.RiazMultiwalled carbon nanotube–polyurethane (MWCNT/PU) composite adsorbent for safranin T and Pb(II) removal from aqueous solution: batch and fixed-bed studiesJ Mol Liq2122015467479
- T.A.KhanV.V.SinghRemoval of cadmium(II), lead(II), and chromium(VI) ions from aqueous solution using clayToxicol Environ Chem928201014351446
- T.A.SalehV.K.GuptaColumn with CNT/magnesium oxide composite for lead(II) removal from waterEnviron Sci Poll Res194201212241228
- V.K.GuptaS.AgarwalT.A.SalehSynthesis and characterization of alumina-coated carbon nanotubes and their application for lead removalJ Hazard Mater185120111723
- V.K.GuptaA.NayakCadmium removal and recovery from aqueous solutions by novel adsorbents prepared from orange peel and Fe2O3 nanoparticlesChem Eng J18020128190
- T.A.KhanA.A.MukhlifE.A.KhanD.K.SharmaIsotherm and kinetics modeling of Pb(II) and Cd(II) adsorptive uptake from aqueous solution by chemically modified green algal biomassModel Earth Syst Environ22016117
- V.K.GuptaS.K.SrivastavaD.MohanS.SharmaDesign parameters for fixed bed reactors of activated carbon developed from fertilizer waste for the removal of some heavy metal ionsWaste Manag1781998517522
- N.MohammadiH.KhaniV.K.GuptaE.AmerehS.AgarwalAdsorption process of methyl orange dye onto mesoporous carbon material–kinetic and thermodynamic studiesJ Colloid Interface Sci36222011457462
- G.WangA.LiM.LiSorption of nickel ions from aqueous solutions using activated carbon derived from walnut shell wasteDesalinat Water Treatment161–32010282289
- L.LonappanT.RouissiR.K.DasS.K.BrarA.A.RamirezM.VermaR.Y.SurampalliJ.R.ValeroAdsorption of methylene blue on biochar microparticles derived from different waste materialsWaste Manag492016537544
- K.YangJ.YangY.JiangW.WuD.LinCorrelations and adsorption mechanisms of aromatic compounds on a high heat temperature treated bamboo biocharEnviron Poll21020165764
- J.LehmannA handful of carbonNature44771412007143144
- X.ChenG.ChenL.ChenY.ChenJ.LehmannM.B.McBrideA.G.HayAdsorption of copper and zinc by biochars produced from pyrolysis of hardwood and corn straw in aqueous solutionBioresour Technol10219201188778884
- X.HanC.F.LiangT.Q.LiK.WangH.G.HuangX.E.YangSimultaneous removal of cadmium and sulfamethoxazole from aqueous solution by rice straw biocharJ Zhejiang Univ Sci B1472013640649
- X.J.TongJ.Y.LiJ.H.YuanR.K.XuAdsorption of Cu(II) by biochars generated from three crop strawsChem Eng J17222011828834
- X.DongL.Q.MaY.LiCharacteristics and mechanisms of hexavalent chromium removal by biochar from sugar beet tailingJ Hazard Mater19012011909915
- Y.YaoB.GaoM.InyangA.R.ZimmermanX.CaoP.PullammanappallilL.YangRemoval of phosphate from aqueous solution by biochar derived from anaerobically digested sugar beet tailingsJ Hazard Mater19012011501507
- D.MohanH.KumarA.SarswatM.Alexandre-FrancoC.U.PittmanCadmium and lead remediation using magnetic oak wood and oak bark fast pyrolysis bio-charsChem Eng J2362014513528
- F.M.PelleraA.GiannisD.KalderisK.AnastasiadouR.StegmannJ.Y.WangE.GidarakosAdsorption of Cu(II) ions from aqueous solutions on biochars prepared from agricultural by-productsJ Environ Manag96120123542
- Y.YaoB.GaoM.ZhangM.InyangA.R.ZimmermanEffect of biochar amendment on sorption and leaching of nitrate, ammonium, and phosphate in a sandy soilChemosphere8911201214671471
- L.ZhaoX.CaoO.MašekA.ZimmermanHeterogeneity of biochar properties as a function of feedstock sources and production temperaturesJ Hazard Mater256201319
- S.KlossF.ZehetnerA.DellantonioR.HamidF.OttnerV.LiedtkeM.SchwanningerM.H.GerzabekG.SojaCharacterization of slow pyrolysis biochars: effects of feedstocks and pyrolysis temperature on biochar propertiesJ Environ Quality41420129901000
- C.E.BrewerK.Schmidt-RohrJ.A.SatrioR.C.BrownCharacterization of biochar from fast pyrolysis and gasification systemsEnviron Prog Sustain Energy2832009386396
- K.HuangH.ZhuRemoval of Pb2+ from aqueous solution by adsorption on chemically modified muskmelon peelEnviron Sci Pollut Res207201344244434
- A.BhatnagarE.KumarA.K.MinochaB.H.JeonH.SongY.C.SeoRemoval of anionic dyes from water using Citrus limonum (lemon) peel: equilibrium studies and kinetic modelingSepar Sci Technol4422009316334
- I.LangmuirThe adsorption of gases on plane surfaces of glass, mica and platinumJ Am Chem Soc409191813611403
- H.M.F.FreundlichOver the adsorption in solutionJ Phys Chem571906385471
- M.M.DubininL.V.RadushkevichEquation of the characteristic curve of activated charcoalChem Zentr111947875
- K.R.HallL.C.EagletonA.AcrivosT.VermeulenPore-and solid-diffusion kinetics in fixed-bed adsorption under constant-pattern conditionsInd Eng Chem Fundam521966212223
- N.BasciE.KocadagistanB.KocadagistanBiosorption of copper(II) from aqueous solutions by wheat shellDesalination16422004135140
- S.DahiyaR.M.TripathiA.G.HegdeBiosorption of heavy metals and radionuclide from aqueous solutions by pre-treated arca shell biomassJ Hazard Mater15022008376386
- P.SenthilKumarS.RamalingamV.SathyaselvabalaS.D.KiruphaS.SivanesanRemoval of copper(II) ions from aqueous solution by adsorption using cashew nut shellDesalination266120116371
- A.GrimmR.ZanziE.BjörnbomA.L.CukiermanComparison of different types of biomasses for copper biosorptionBioresour Technol997200825592565
- E.PehlivanT.AltunŞ.ParlayiciModified barley straw as a potential biosorbent for removal of copper ions from aqueous solutionFood chem1354201222292234
- M.A.HossainH.H.NgoW.S.GuoT.SetiadiAdsorption and desorption of copper(II) ions onto garden grassBioresour Technol1212012386395
- F.J.Cerino-CórdovaP.E.Díaz-FloresR.B.García-ReyesE.Soto-RegaladoR.Gómez-GonzálezM.T.Garza-GonzálezE.Bustamante-AlcántaraBiosorption of Cu(II) and Pb(II) from aqueous solutions by chemically modified spent coffee grainsInt J Environ Sci Technol1032013611622
- Y.H.LiJ.DingZ.LuanZ.DiY.ZhuC.XuD.WuB.WeiCompetitive adsorption of Pb2+, Cu2+ and Cd2+ ions from aqueous solutions by multiwalled carbon nanotubesCarbon4114200327872792
- Y.LiF.LiuB.XiaQ.DuP.ZhangD.WangZ.WangY.XiaRemoval of copper from aqueous solution by carbon nanotube/calcium alginate compositesJ Hazard Mater17712010876880
- Y.GeZ.LiD.XiaoP.XiongN.YeSulfonated multi-walled carbon nanotubes for the removal of copper(II) from aqueous solutionsJ Ind Eng Chem204201417651771
- O.MoradiThe removal of ions by functionalized carbon nanotube: equilibrium, isotherms and thermodynamic studiesChem Biochem Eng Quart2522011229240
- V.C. SrivastavaI.D.MallI.M.MishraModelling individual and competitive adsorption of cadmium(II) and zinc(II) metal ions from aqueous solution onto bagasse fly ashSep Sci Technol4112200626852710
- V.C.SrivastavaI.D.MallI.M.MishraRemoval of cadmium(II) and zinc(II) metal ions from binary aqueous solution by rice husk ashColloid Surfaces A: Physicochem Eng Asp31222008172184
- G.P.RaoS.SatyaveniA.RameshK.SeshaiahK.S.MurthyN.V.ChoudarySorption of cadmium and zinc from aqueous solutions by zeolite 4A, zeolite 13X and bentoniteJ Environ Manag8132006265272
- S.R.ShuklaR.S.PaiAdsorption of Cu(II), Ni(II) and Zn(II) on modified jute fibresBioresour Technol9613200514301438
- M.G.da FonsecaM.M.de OliveiraL.N.ArakakiRemoval of cadmium, zinc, manganese and chromium cations from aqueous solution by a clay mineralJ Hazard Mater13712006288292
- A.KayaA.H.ÖrenAdsorption of zinc from aqueous solutions to bentoniteJ Hazard Mater12512005183189
- Guo XY, Liang S, Tian QH. Removal of heavy metal ions from aqueous solutions by adsorption using modified orange peel as adsorbent. In: Advanced Materials Research 2011 (Vol. 236, pp. 237–240). Trans Tech Publications.
- U.MaheshwariB.MathesanS.GuptaEfficient adsorbent for simultaneous removal of Cu(II), Zn(II) and Cr(VI): kinetic, thermodynamics and mass transfer mechanismProc Safety Environ Protect982015198210
- S.LagergrenAbout the theory of so-called adsorption of soluble substancesKung Svenska Vetenskapsakademiens Handl2441898139
- Y.S.HoG.McKayPseudo-second order model for sorption processesProcess Biochem341999451465
- G.E.BoydA.W.AdamsonL.S.MeyersThe exchange adsorption of ions from aqueous solutions by organic zeolites IIJ Am Chem Soc69194728362848
- W.J.WeberJ.C.MorrisKinetics of adsorption on carbon from solutionJ Sanit Eng Div Am Soc Civ Eng8919633160