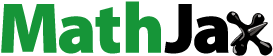
Abstract
Limited bioavailability of green tea polyphenols hampered their delivery to tumor and hence therapeutic effectiveness. This study investigated the antitumor activity of polyphenon-E (PE) encapsulated into chitosan nanoparticles (CSNPs) in Ehrlich solid tumor in mice. CSNPs-PE, with a particle size of 53–69 nm showed 83% entrapment efficiency and a sustained release of PE in pH = 7.4 at 37 °C. The data demonstrated a higher percentage of released drug in case of less crosslinked formulations. Ehrlich ascites carcinoma (EAC) cells (2.5 × 106/0.2 ml/mouse) were injected subcutaneously in the back of mice. Oral administration of CSNPs-PE for 30 days produced a significant decrease in tumor volume (53%) and weight (60%) compared with free PE and voids CSNPs (72%). Compared with free PE and control, cell cycle revealed G0/G1 arrest associated with decrease in proliferating cell nuclear antigen (PCNA). In tumor tissue of CSNPs-PE treated mice, compared with free PE, there were; 1) induction of Bax and p53, 2) activation of caspases-3,-8 and -9, and CD95, 3) decrease in Bcl-2 expression of 4) inhibition of VEGF and CD31 expressions in tumor tissue. In conclusion, encapsulation of PE into CSNPs provided a good platform for cancer chemotherapy and raised existing application of different polyphenols for nanochemotherapy/prevention.
Introduction
The therapeutic potential of natural products against cancer growth and proliferation is limited by their poor oral bioavailability and subsequent delivery to the tumor. Nanotechnology-based oral delivery of green tea polyphenols can overcome the limitation of conventional treatment due to physiochemical prosperities of the nanoparticles [Citation1]. Epigallocatechin gallate encapsulated into chitosan nanoparticles (CSNPs) had a remarkable inhibitory effect on human melanoma cell growth both in vitro and in vivo [Citation2,Citation3] . Chitosan (CS) is a natural polysaccharide obtained from hard outer skeleton of crustaceans [Citation4]. It has superior physicochemical and biological characteristics, and consequently a wide range of biomedical applications [Citation5,Citation6] . CSNPs also improved plasma exposure of green tea extract through an enhancement of intestinal stability [Citation7].
Cancer is the leading disease worldwide with the high mortality rate in human. The main target in cancer chemotherapy and prevention is induction of cell cycle arrest, apoptosis and inhibition angiogenesis [Citation8]. Meanwhile, nature provides plenty of medicinal products that consider as a great source of nontoxic drugs for cancer treatment. Plant derived compounds rich in polyphenols proved to possess great therapeutic characteristics by targeting molecular pathways of apoptosis [Citation9]. Among natural dietary compounds is green tea polyphenols which showed impressive chemopreventive potential in several cancer types [Citation10] including prostate cancer [Citation11], human melanoma cells [Citation3] and lung cancer [Citation12].
Polyphenon-E (PE) is green tea extract contains 60% pure catechins with high EGCG content [Citation13,Citation14] . It composed of (−)-Epigallocatechin gallate (EGCG), 65%; (−)-Epigallocatechin (EGC), 4%; (−)-epicatechin, 9%; (−)-epicatechin-3-gallate, 6%; (−)-gallocatechin-3-gallate, 4%; (−)-catechin-3-gallate, 0.2%; gallocatechin, 0.2%; catechins, 1.1% and caffeine, 0.7% [Citation15]. PE remarkably down-regulated several miRNA expression profile in MCF-7 cells [Citation16], induced apoptosis in human bladder cancer cells [Citation17] and inhibited metastatic progression of prostate cancer [Citation18]. It also inhibited N-ethyl-N-hydroxyethylnitrosamine induce carcinogenicity on renal cells [Citation13].
Ehrlich tumor is the most common model of cancer that initially appeared as spontaneous breast cancer in mice then used as an experimental transplantable tumor in mice [Citation19]. The oncostatic effect of green tea catechins was shown in many types of cancers, while its effect on Ehrlich cell carcinoma was not fully studied. Therefore, the current study investigated the antitumor efficiency of oral treatment with polyphenon-E encapsulated into CSNPs on solid Ehrlich tumor model in mice.
Materials and methods
Chemicals
The polyphenon-E (PE), chitosan, (CS) of medium MW, % N-deacetylation about 81%, as determined by elemental analysis, and sodium triphosphate pentabasic (STPP) were purchased from Sigma Aldrich (St. Louis, MO). Glacial acetic acid, phosphate-buffered saline (PBS), and all other reagents were of analytical grade. Anti PCNA, VEGF, CD31, P53, CD95, BcL-2, BAX, and caspases 3, 8 and 9 antibodies were obtained from Thermo Scientific. Mouse monoclonal antibody (Cat No MS-106-P0, USA), (Thermo scientific, Rabbit polyclonal antibody, Cat No RB-9031-P0, USA), (Thermo scientific, Rabbit polyclonal antibody, Cat No RB-10333-P0, USA), (rabbit IgG antibody Lot No: GR 110207–3 abcam USA), (Mouse IgG1 primary antibody Lot No: 5120612021 Milteny Biotec), (mouse IgG1 antibody CodeNo: F7053 DAKOA/S Denmark), (monoclonal IgG antibody Lot No: GR142124-1 abcam USA), (Cat No 51-68654X Lot:16374 Rabbit IgG BD Biosciences USA), (Rabbit monoclonal primary antibody ab 32125 Lot No: YH080907D abcam USA and goat pAb to Rabbit IgG as a secondary antibody (Lot No: 15232–1 abcam USA), and (Rabbit monoclonal IgG antibody Lot No: GR63053-2 abcam USA), respectively.
Preparation of polyphenon-E loaded CSNPs
Chitosan nanoparticles (CSNPs) were prepared using ionotropic gelation technique [Citation20] with a slight modification. Briefly, an aqueous CS solution (0.1% w/v) was obtained by dissolving CS in 0.1% w/v acetic acid. Then, 0.1% STPP aqueous solution was added drop wise to CS solutions under mild magnetic stirring for 20 min. Opalescent suspensions were formed spontaneously at room temperature which were further examined as the hydrogel NPs. The PE-60 loaded CSNPs were prepared using the same procedure but with dissolving a 250 mg/kg body weight of the PE-60 in the CS solution.
Morphology and particle size measurements
The morphology and particles size measurements of the developed hydrogel NPs were performed by transmission electron microscopy (TEM, JEOL, JEM-1230, Japan Ltd.) at accelerating voltage of 200 kV. Samples were prepared for imaging by drying the NPs on a copper grid that is coated with a thin layer of carbon. The size of the plain and PE-60-loaded CSNPs was also determined by dynamic light scattering (DLS) (Malvern nanosizer, Malvern Instruments Ltd., Worcestershire, UK).
Swelling behavior of NPs
The swelling behavior of the freeze-dried plain CSNPs was studied by incubating certain amount of the dry particles (10–15 mg) in an Eppendorf tube containing 1.5 ml of PBS (pH 7.4) with shaking at 120 rpm at 37 °C. At certain intervals, swollen samples were separated from the PBS solution and weighted to calculate their swelling percentages using the following relationship:where Wo is the initial weight and Wt is the weight of the swollen particles at time t. The swelling data represents the average ± SD from three independent swelling experiments.
Drug entrapment efficiency
The entrapment efficiency (EE%) of PE-60 was determined via separation of the PE-60-loaded CSNPs from a certain volume of their aqueous suspension by ultra-centrifugation at 14,000g and 4 °C for 20 min (SORALL® Bioguge Stratos Ultracentrifuge) followed by determination of the unloaded amount of PE-60 remaining free in the supernatant using UV-Vis spectrophotometry (Evolution™ 300 UV-Vis spectrophotometer, Thermo Scientific, USA) at λmax of 442 nm. The entrapment efficiency (EE%) of the PE-60 was then calculated according to the following relationship:where mi and mr are the amounts (mg) of the PE-60 initially loaded and remained in the NPs, respectively.
In vitro release study
The in vitro cumulative release study of PE-60 from the developed CSNPs was carried out in PBS (pH 7.4). Briefly, the PE60-loaded CSNPs were separated from the prepared aqueous suspension by ultra-centrifugation at 14,000g and 4 °C for 20 min (SORALL® Bioguge Stratos Ultracentrifuge) followed by lyophilization of the precipitate. Then, the release study was carried out through incubating pre-weighed samples of the dry powder (10–15 mg) while shaking at 120 rpm in 2 ml of buffer solutions, PBS (pH 7.4) at 37 °C. After incubation, 100 µl aliquots were removed from the buffer solution to be analyzed by UV-Vis spectrophotometry (Evolution™ 300 UV-Vis spectrophotometer, Thermo Scientific, USA) at λmax of 442 nm. The withdrawn sample was replaced with equal volume of fresh buffer solution to keep the volume of release fluid constant. The collected data represents the mean ± SD from three independent release experiments.
Animal grouping
The current study was conducted on female albino mice weighing 25–30 gm purchased from Cairo Oncology center. They were housed in standard sized cages under standard laboratory conditions (26 °C, 12 h light/dark cycle). Ehrlich Ascites carcinoma cells were obtained from Cairo oncology center. Treatment of animals was carried out following the guide for care and use of experimental animals according to Institutional Animal Ethics Committee (IAEC) of Mansoura University. All animal were injected with 2 × 106 Ehrlich ascites carcinoma cells (EAC) (0.2 ml/mice) subcutaneously on the back of each moce. Day of injection was assigned as day 0. On day 5 the animals were randomized and divided into 4 groups each containing 10 animals: Control group (C), which mice were transplanted with EAC cells. The second group was orally administrated with 0.1 ml chitosan nanoparticles (Cs) on day 5 after transplantation 3 times a week for 30 days. The third group (CSNPs-PE) was administrated with CSNPs-PE on day 5 of EAC cells injection 3 times a week for 30 days with total volume 0.1 ml orally using stomach tube. The forth group (PE) was orally administrated with 0.1 ml free polyphenon-E (PE) on day 5 after transplantation 3 times a week for 30 days. In all cases the concentration of the PE is 250 mg/kg body weight.
After completion of the experimental period of 30 days, overnight fasted animals were sacrificed and tumors were excised.
Tumor weight and volume
A noninvasive standard method for determination of tumor volume was used [Citation21]. Tumor volume was measured using external caliper starting from 10th day after transplantation of tumor cells and subsequently recorded every week along the experimental period of 30 days. Tumor volume was estimated by the modified ellipsoidal formula.
After completion of experimental period of 30 days, overnight fasted animals were sacrificed and tumors were excised and weighted.
Each tumor was divided into two portions for immunecytochemical, biochemical and molecular investigations.
Molecular determination
Solid tumor preparation for Flow Cytometry was performed by mechanical dissociation technique [Citation22].
Cell cycle analysis
The obtained tumor cells were permeabilized, fixed then nuclear DNA was labeled with propidium iodide (PI) using Cycle TEST PLUS DNA reagent kit [Citation23]. The distribution of nuclear DNA of cell cycle phase was determined on FACS, fluorescence detector using Cell Quest software (Becton Dickinson). A total of 10,000 events was acquired and analysis of flow cytometric data was performed using Mod Fit software. A histogram of DNA content versus counts has been displayed.
Apoptosis analysis
Annexin-V FITC/PI, ApoAlert kit from Clontech (Palo Alto, CA) was used to distinguish between apoptosis and necrosis according to the instructions described by the manufacturer. Before staining, cells were fixed using 2% paraformaldehyed and permeabilized with Saponin, (0.5% v/v in PBS, pH 7.4). The cells were washed and suspended in PBS (pH 7.4) with 0.2% BSA, divided into aliquots for flow cytometric analyses. The flow cytometry analyses were performed on an FACSCaliburTM cytometer (BD Biosciences, San Jose, CA) using CellQuest Pro software (Becton Dickinson) for data acquisition and analysis [Citation24]. A total of 10,000 events were acquired and the cells were properly gated for analysis.
Determination of proliferation marker, and pro- and anti-apoptotic proteins
For determination of the expression of p53, Bax, Bcl-2, CD95 and caspases 3 and 9, tumor cells were permeabilized and fixed as described earlier. Cells (1X106) from each group were incubated for 30 min with anti-Bcl-2 antibody, anti-Bax antibody for flow cytometry analysis of Bcl-2 and Bax, respectively. A mouse anti-P53 FITC, was used for P53 analysis by flow cytometry. In case of caspases 3, 8 and 9 FITC rabbit anti-active caspase 3, anti-caspase 8 antibody or rabbit monoclonal anti-caspase 9 (E23) antibody were used respectively. Cells were washed thoroughly with PBA/BSA, centrifuged at 400g for 5 min, re-suspended in 0.5% paraformaldehyde in PBS/BSA and analyzed on a Flow cytometer equipped with 488 nm Argon laser light source and a 515 nm band pass filter for FITC-fluorescence. A total of 10,000 events were acquired and the cells were properly gated for analysis.
Immunohistopathological assessment
Tumor tissues were fixed in 10% neutral-buffered formalin, and embedded in paraffin and 5 μm sections were prepared for staining. The proliferating cell nuclear antigen (PCNA), platelet endothelial cell adhesion molecule-1 (PCAM/CD31) and vascular endothelial growth factor (VEGF) were obtained from ThermoFisher Scientific, USA with the following specifications; (PCNA, Mouse monoclonal antibody, MS-106-P0, USA), (CD31, rabbit polyclonal antibody, RB-10333-R7) and (VEGF, rabbit polyclonal antibody, RB-9031-R7). Immunohisochemical studies were performed by incubating tumor sections with suitable dilutions 1:50–1:400 primary antibodies. Negative control sections were prepared without primary antibody. All tissue sections were inspected using light microscopy to obtain images using Olympus (IX51) microscope [Citation25].
The PCNA and VEGF were assessed and scored independently by two investigators using light microscopy. The numbers of positive cancer cell nuclei were scored in 400 cancer cells and the ratios of stained to total cells expressed as percentage were defined as PCNA or VEGF indices [Citation23]. Scoring of CD31 expression was performed on computer assisted digital image analysis (Digital morphometric study). Tissue sections were photographed using Olympus digital camera fitted on Olympus microscope. The images of tissue sections were analyzed using Video-Test Morphology software (Russia) adapted for percent area measurement and object counting.
Results
Preparation and characterization CSNPs and CSNPs-PE
Chitosan nanoparticles (CSNPs) were prepared using ionotropic gelation technique as illustrated in . The average particle size of the void CSNPs (A1 and A2) and the PE-loaded CSNPs (A1-D and A2-D) obtained at different STPP concentrations was determined using dynamic light scattering and shown in . The data show that increasing the STPP content tends to reduce the size of the resulting NPs. For instance, the size of the void CSNPs A1 has reduced from 21.4 ± 7.6 nm to 16.7 ± 9.2 nm upon doubling the volume of the crosslinking agent, STPP. The same result was also noted in the case of PE-loaded NPs (A1-D and A2-D). The incorporation of the bioactive constituent, PE has increased the particle size as compared to the corresponding plain CSNPs (). CSNPs-PE, with a particle size between 53 and 69 nm was determined by dynamic light scattering and transmission electron microscopy. These particle size results were also confirmed from the obtained TEM micrographs shown in (A and B). The TEM micrographs also demonstrated an almost spherical morphology of the prepared NPs. The entrapment efficiency (EE%) of PE in the developed CSNPs was determined and found to be in the range between 83.0 ± 2.1% and 86.3 ± 1.6% depending on the content of the crosslinking agent, STPP ().
Fig. 2 (A&B) Transmission electron micrographs of the prepared void (A2) nanoparticles, and catechin-loaded CS (A2-D) nanoparticles. (C) Swelling behavior of the CS nanoparticles of different STPP concentrations (A1 and A2) in PBS (pH 7.4) at 37 °C. (D) Cumulative release study of the loaded Catechin from the CS nanoparticles of different crosslinking extents (A1-D and A2-D) at 37 °C in PBS (pH 7.4). The values are average of three triplicates.
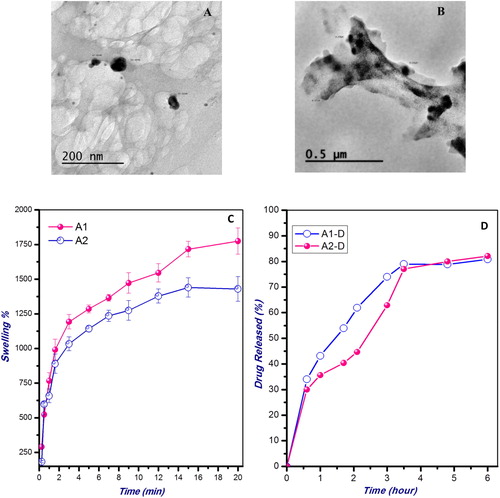
Table 1 The average particle size of the prepared void and PE-loaded CS nanoparticles of different compositions and their entrapment efficiencies.
Swelling ability of the prepared NPs is one of the key characteristics that determine their appropriateness for various biomedical applications. The swelling behavior of the void CSNPs obtained at different STPP concentrations (A1 and A2) was studied as shown in C. The data reveals that increasing the STPP content in the NPs tends to reduce the swelling values attained at equilibrium.
The cumulative release pattern of the loaded PE from the CSNPs of different crosslinking extents in PBS (pH 7.4) at 37 °C is illustrated in D. As shown in the figure, the percent of PE released increased gradually with time until attaining almost constant value after about 3–4 h. The data also demonstrates a relatively higher percentage of the drug released in the case of the less crosslinked formulation (A1) as compared to the more crosslinked nanoparticles (A2).
Antitumor response
The antitumor effect of chitosan nanoparticle encapsulating polyphenon-E (CSNPs-PE) against EAC tumor implanted in mice was demonstrated by assessing tumor weights (A), and tumor volume (B) 30 days after EAC cell inoculation. The EAC-bearing mice demonstrated a significant increase in tumor weight and volume. Compared to the tumor-bearing mice, void CS-NPs, free PE and CSNPs-PE treatment resulted in significant reductions in tumor weights by 53%, 60% and 72% respectively. Similar trend was demonstrated in the tumor volume which significantly reduced along the experimental period when CSNPs-PE has been given orally. In all cases CSNPs-PE produced a pronounced inhibitory effect on tumor weight and volume than Free PE.
Fig. 3 Effect of polyphenon-E (PE) encapsulated into chitosan nanoparticles (CSNPs-PE) on (A) body weight changes and (B) tumor volume. EAC cells (2x106 cells/mouse) were injected subcutaneously to induce solid tumor in Swiss albino mice. CSNPs-PE was orally administered daily for 30 days. Values are mean ± SEM of three determinations. *P < .05 vs control group and ■P < .05 vs PE-treated group.
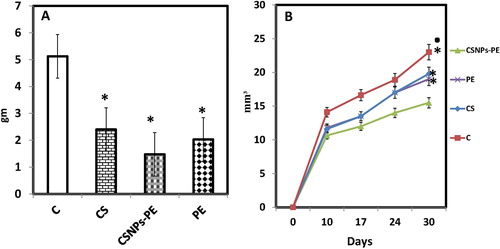
To investigate if the reduction in tumor growth induced by CSNPs-PE administration is due to inhibition of tumor cell proliferation, we analyzed the cell cycle using flow cytometer. The percentage of cells in S-phase was decreased (P < .05) from approximately 25.09 ± 1.76 % in tumor bearing control mice to 4.13% ± 0.21% and 9.90% ± 1.4 treated with CSNPs-PE and PE respectively (). The cell cycle analysis also revealed cycle arrest at G0/G1 in tumors obtained from mice treated with CSNPs-PE and PE respectively.
Fig. 4 Effect of polyphenon-E (PE) encapsulated into chitosan nanoparticles (CSNPs-PE) on cell cycle phases. The cell cycle distribution of EAC nuclear DNA was determined by flow cytometer using propidium iodide staining in EAC bearing-mice in different animal groups. The DNA histogram shows the distribution and the percentage of cells in phases of the cell cycle. Histogram display of DNA content (x-axis, PI-fluorescence) versus counts (y-axis) has been shown. Values are expressed as percentage of the cell population in the G0/G1, S and G2/M phases of cell cycle. The CSNPs-PE caused cell cycle arrest at G0/G1 phase with decreased cell population in S phase.
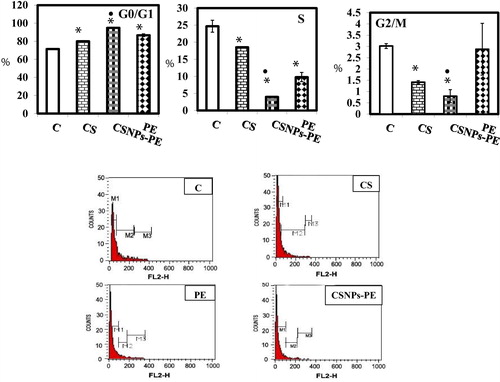
The proliferating activity of the tumor was studied by evaluating the related cell cycle antigen expression, the proliferating cell nuclear antigen (PCNA) using immunohistochemical approach on formalin-fixed paraffin embedded tumor (). Encapsulation of PE in CS-NPs produced a significantly higher inhibition in the PCNA expression by 56% than control tumor. In addition, the void CSNPs and free PE treated group showed remarkable reduction in the PCNA expression by 33% and 40% respectively compared to the tumor-bearing control group (A). The flow cytometer analysis of p53 levels in tumor tissue of Ehrlich carcinoma bearing mice under different conditions is shown in B. Associated with increased apoptosis, p53 significantly elevated in tumor tissue from mice treated with CSNPS-PE two fold compared with void CSNPs and free PE-treated EAC-bearing mice (B).
Fig. 5 Effect of polyphenon-E (PE) encapsulated into chitosan nanoparticles (CSNPs-PE) on expression of PCNA (A) and p53 (B) in tumor tissues of mice. Tumor sections were stained using specific antibodies. Counterstaining was performed with hematoxylin. Photomicrographs (magnification, ×20) show representative pictures from two independent samples (A). P53 was estimated using flow cytometer (B). *P < .05 vs control group and ■P < .05 vs PE-treated group. CSNPs-PE downregulated PCNA and upregulated p53 in tumor tissue.
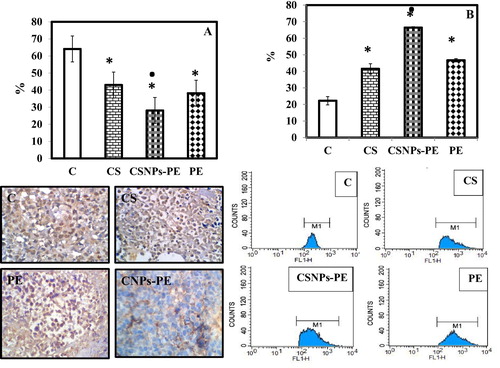
shows the immunohistochemical detection of VEGF and CD31 in different tumor bearing mice. After 30 days, the VEGF in CSNPs-PE-treated group diminished significantly by 34% compared with the tumor-bearing control group. Oral administration of void CSNPs or free PE reduced the VEGF by 15% and 27% respectively when compared with the control group (A). Meanwhile, the immunohistochemical expression of the endothelial markers, CD31 in EAC as possible evidence of vasculogenic mimicry was investigated (B). Immunostaining with antibodies against CD31 revealed that control bearing tumor mice showed significantly higher immunostained tumoral cells than other treated groups. The CSNPs-PE-treated groups showed markedly lower immunostained tumoral cells than CSNPs and PE-treated animals (B). These data suggest that PE inhibited the neoangiogenesis in tumor tissues and the CSNPs-PE is more efficient than PE alone.
Fig. 6 Effect of polyphenon-E (PE) encapsulated into chitosan nanoparticles (CSNPs-PE) on expression of angiogenic indicators VEFG and CD31 in tumor tissues of mice. Tumor sections were stained using specific antibodies for each parameter. Counterstaining was performed with hematoxylin. Photomicrographs (magnification, ×20) show representative pictures from two independent samples. *P < .05 vs control group and ■P < .05 vs PE-treated group. CSNPs-PE downregulated expressions of VEGF and CD31 in tumor sections indicating inhibition of angiogenesis.
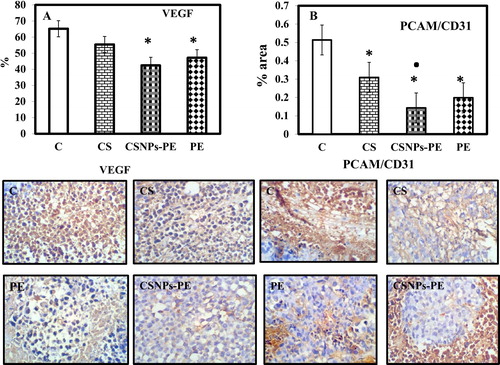
Annexin/PI-labeled fluorescent technique was used to determine if the growth-inhibitory effects of CSNPs-PE correlated with enhanced induction of apoptosis. The data showed increased numbers of apoptotic cells and significantly (P > .001) lower numbers of live cells obtained from mice treated with CSNPs-PE compared with the control tumor bearing mice ( and ). CSNPs-PE also produced a significant increase in the necrotic cell compared with the control tumor mice. The staining with annexin-V of tumor cell obtained from void CSNPs and free PE-treated mice showed increased apoptosis by 9% and 22% compared to control tumor. The data also show that the apoptotic cells in case of CSNPs-PE-treated mice is significantly (P > .001) higher than free PE-treated rats. This effect is reflected on the percentage of live cells that is significantly lower in CSNPs-PE than free PE and CS treated rats. These findings indicate that the effect is more prominent with CSNPS-PE than free PE indicating the advantage of the catechin encapsulation in CS nanoparticles.
Fig. 7 Flow cytometry data showing examples of the data generated by FACS. Dual parameter dot plot of FITC-fluorescence (x-axis) versus PI-fluorescence (y-axis) has been shown in logarithmic fluorescence intensity. The events shown in the lower left quadrant represent viable cells, and the upper left quadrant is indicative of necrotic cells that have taken up the PI. The percentage of early apoptotic cells that are Annexin V positive only is represented in the lower right quadrant, and events depicting late apoptotic cells that are Annexin V/ PI positive are shown in the upper right quadrant. CSNPs-PE produced remarkable late apoptosis and necrosis. Values are mean ± SEM of three determinations. *P < .05 vs control group and ■P < .05 vs PE-treated group.
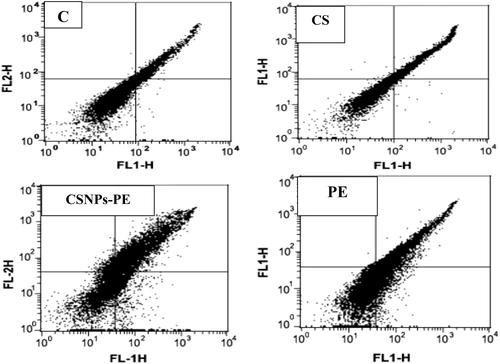
Table 2 Effect of polyphenon-E (PE) encapsulated into chitosan nanoparticles (CSNPs-PE) on EAC apoptosis and necrosis using flow cytomety. In a double label system, unfixed EACs from different animal groups of tumor-bearing mice were labeled with PI and Annexin V Fluos and then fixed and analyzed on a Flow cytometer.
The proteins expression involved in apoptosis were evaluated and displayed in (A) and a representation of the flow cytometer charts is indicated in (B). The expression of Bax was increased by CSNPs-PE oral treatment. Concurrently, the expression of Bcl-2 was decreased by CSNPs-PE (). The data also showed the advantage of encapsulated PE compared with native PE and CSNPs. There was a significant increase in activation of the caspases 3, 8 and 9 respectively in the animals treated with CSNPs-PE as compared to free PE and void CSNPs (). The changes indicated that the encapsulated form of PE is more effective than the native PE. A significant increase in the expression of CD95 demonstrated in the EAC cell obtained from tumor-bearing mice treated with CSNPs-PE compared with void CSNPs and natural PE ().
Fig. 8 (A) Percent expression of Bcl-2, Bax, CD95, and caspases 3, 8, and 9 of EAC cells from EAC-bearing animals of all groups measured by flow cytometry using specific antibody. Values are mean ± SEM of three determinations. *P < .05 vs control group and ■P < .05 vs PE-treated group. The CSNP-PE oral treatment for 30 days showed increased expressions of Bax, CD95, and caspases-3, 8, and 9 and downregulated Bcl-2 expression in tumor. (B) Flow cytometry data showing examples of the data generated by FACS.
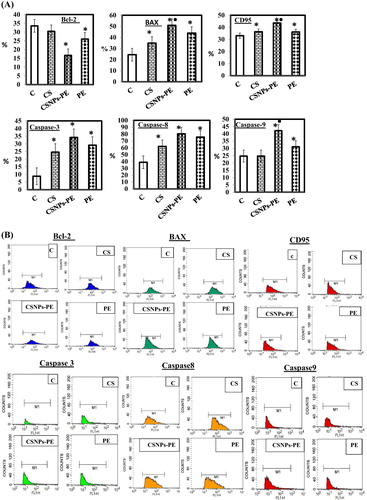
Discussion
Tea catechins have remarkable antitumor impact, however they demonstrated a limited pharmacological application in clinical settings due to their poor in vivo stability, limited bioavailability, degradation in the gastrointestinal canal and inadequate intestinal absorption [Citation7]. CS nanoformulations for drug delivery showed to enhance bioavailability and increased the absorption of tea polyphenols [Citation26,Citation27] . CSNPs were prepared by interaction of amino group of CS with phosphate group of STPP in aqueous medium. The synthesized CSNPs provided a formulation for green tea catechins to be embedded in the polymeric network [Citation11], and therefore green tea catechins can be protected from degradation in the harsh milieu of the stomach and the gastrointestinal tract [Citation28]. This was supported by a steady sustained release of the PE and in agreement with the swelling pattern of the particles and drug release (). The concentrations of CS and STPP are important to define the size of NPs. After several trials, we used this particular preparation to synthesize spherical NPs with 53–69 nm and with 83% entrapment efficiency. Reports have shown that using NPs made from CS can improve biocompatibility and decrease possible toxicity [Citation29]. This is consistent with reported work that CSNPs enhanced the plasma exposure of EGCG in mice through an enhancement in intestinal stability [Citation7]. This lends credence to the newly developed CSNPS-PE that can enhance and extend the intestinal absorption of PE. In addition, CSNPs can adhere to the mucosa of the intestinal membrane and open up the tight junction between epithelial cells [Citation30].
The main objective of the present nanoformulation CSNPs-PE was to enhance the antitumor effect of green tea catechin in Ehrlich tumor in vivo and to evaluate the effect of CSNPs-PE versus free PE on tumor growth and proliferation. The present data demonstrated that CSNPs-PE remarkably reduced the tumor growth in terms of tumor volume and weight compared with tumor implanted mice and mice who received free PE and void CS. The oncoprotein p53 that is essential for the inhibition of cell proliferation via impeding cell cycle progression and induction of apoptosis [Citation31] was significantly increased in Ehrlich tumor after oral treatment with CSNPs-PE compared with free PE and void CS.
The cell cycle progression was analyzed to investigate if the reduction in tumor growth induced by CSNP-PE administration is due to inhibition of tumor cell proliferation. The percentage of cells in S-phase was significantly decreased in tumor obtained from CSNPs-PE than PE treated and control mice indicating the effectiveness of nanoformulation. The cell cycle analysis also revealed cycle arrest at G0/G1 in tumor obtained from mice treated with CSNPs-PE compared with other treated groups. These data also demonstrated that PE in nanoformulation is more effective than free PE. Furthermore, The CSNPs-PE group tumors, relative to control tumors, had significantly decreased PCNA expression that match to the reduced cell population in S-phase results suggesting a decrease in the proliferation of tumor cells by CSNPs-PE. This effect is remarkably evident in CSNPs-PE treated mice compared with free PE indicating the advantage of encapsulation PE in chitosan nanoparticles. These data also support the reduced tumor growth observed in this study.
The prime pathway followed by cancer cells is to suppress apoptosis and p53 function; therefore appropriate anticancer treatments would stimulate apoptotic mechanisms and regain p53 activity to inhibit cancer proliferation. Apoptosis consists of mitochondrial and transmembrane death receptors pathways which comprise of the activation the effector caspases via the initiator caspases in cell death [Citation32]. Consistent with increased expression of p53 in Ehrlich tumor of mice treated with CSNPS-PE, there was greater activation of CD96, caspases 3, 8 and 9 with up-regulation of Bax and down-regulation of Bcl-2 compared to free PE and void CSNPs. Changes in the Bax to Bcl-2 ratio resulted in the destabilization of the mitochondrial membrane and liberation of the proteolytic caspases and cleavage of PARP [Citation33] that lead to cell death [Citation3].
These results confirm previous study that PE increased lung cancer cell death by inhibition of Bcl-2 related apoptosis through reducing mitochondrial membrane potential and enhancing PARP cleavage [Citation34]. Furthermore, PE induced growth inhibited in colon cancer cells [Citation35]. The later authors demonstrated similar potencies of PE and EGCG and preferred PE for easier preparation and synergistic effect of mixed catechins. In a recent report, PE caused severe and prolonged endoplasmic reticulum stress in prostate cancer cells leading cell death through a caspase-independent pathway of necrotosis [Citation15].
It is well known that p53 functions to stimulate protective mechanisms against tumor growth by regulating the expression of both Bax and Bcl-2 [Citation36,Citation37] . It also acts as a tumor suppressor through its capacity to induce cell cycle arrest and apoptosis in response to a variety of chemotherapeutic drugs [Citation38]. These results confirm other studies in which induction of apoptosis by tea polyphenols mediated in mouse skin tumors [Citation39] and human fibrosarcoma HT-1080 cells through intrinsic cell death pathways in mouse skin tumors [Citation39] and human fibrosarcoma HT-1080 cells [Citation40]. Similarly, Epigallocatechin-3-gallate induces apoptosis in estrogen receptor-negative human breast carcinoma cells via modulation in protein expression of p53, Bax and caspase 3 activation [Citation41]. Thus, CSNPs-PE played an important role to influence p53 to transduced signals into tumor-suppressive apoptotic and growth arresting responses. In addition, oral treatment with CSNPs-PE can induce apoptotic changes including activation of Bax, caspases 3, 8, and 9, and p53 and abrogated the activation of Bcl-2 in Ehrlich tumor ().
Fig. 9 Molecular mechanism of cancer cell death induced by CSNPs-PE in tumor tissue. CSNPs-PE upregulated proapoptotic proteins BAX, and p53, activate caspases-3, 8 and 9, and CD95, with concomitant decrease in the expression of Bcl-2 and inhibition of VEGF and CD31 expressions in tumor tissue. These changes lead to apoptosis and inhibition of tumor growth.
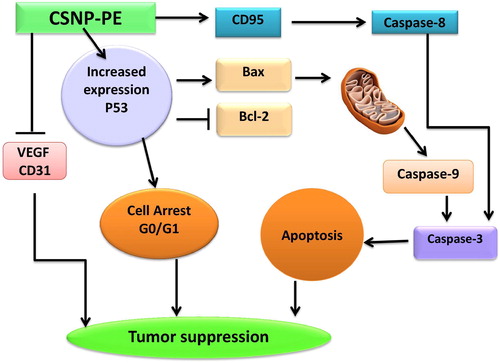
Angiogenesis is largely essential for tumor development and expansion of tumor mass because neovascularization permits rapid tumor growth by providing an exchange of nutrients, oxygen, and paracrine stimuli to the tumor [Citation42]. Therefore, in this study, we used immunohistochemical staining for CD31 and VEGF, as markers of angiogenesis, to determine the effect of free PE and encapsulated nanoform on Ehrlich tumor angiogenesis in mice. CSNPs-PE-treated tumor had reduced angiogenesis than the free PE tumor as compared with control tumor. These results also suggest that a pronounced decrease in tumor angiogenesis is associated with a decrease in tumor size of Ehrlich tumor in the female mice treated with CSNPs-PE compared to those in the control mice. There are several mechanisms described for the antiangiogenic effect of tea catechins. This includes a decrease tumor cell VEGF synthesis, a decrease in its binding to VEGF receptors [Citation43], and inhibition of VEGF receptor phosphorylation [Citation44,Citation45] . Thus, CSNPs-PE exhibited anti-angiogenic effect by inhibiting VEGF and CD31 to prevent the communication between the tumor and its microenvironment and ultimately impede tumor growth and expansion ().
The major disadvantage associated with inability of many natural compounds in clinical setting is their limited bioavailability [Citation2]. The present study overcome this limitation and demonstrated remarkable anticancer effect of PE encapsulated in CSNPs than free PE. In more recent study, EGCG encapsulated in CSNPs showed significant longer half-life than free EGCG indicating that nanoformulation increased stability and bioavailability leading to better clinical results [Citation3]. The advantage of high surfaces area to volume ratio and ultra-small size of NPs suggests an increased in their conjugation with tumor cells which characterized by bigger size leading to more medical efficacy [Citation46]. In addition, the biodegradability and biocompatibility of CS eliminates possibility of any systemic toxicity [Citation1,Citation47] . It is interesting that void CS showed antitumor effect nevertheless, it is less than encapsulated PE. Chitosan is highly biodegradable and used as immunoadjuvant in cancer therapy [Citation48] to enhance the photodynamic therapy of tumors [Citation49]. This observation in agreement with pervious study using CS encapsulated tea polyphenols on HepG2 cells [Citation50] offering additional advantage to medical utility of CS.
In conclusion, encapsulation of PE in chitosan nanoparticles provide good platform for cancer chemotherapy and possess strong rational for further trials with different cancers and raise exiting application of different polyphenols for nano-chemotherapy/prevention.
References
- M.PrabaharanChitosan-based nanoparticles for tumor-targeted drug deliveryInt J Biol Macromol72201513131322
- I.A.SiddiquiV.M.AdhamiD.J.BharaliB.B.HafeezM.AsimS.I.Khwajaet al.Introducing nanochemoprevention as a novel approach for cancer control: proof of principle with green tea polyphenol epigallocatechin-3-gallateCancer Res69200917121716
- I.A.SiddiquiD.J.BharaliM.NihalV.M.AdhamiN.KhanJ.C.Chamcheuet al.Excellent anti-proliferative and pro-apoptotic effects of (−)-epigallocatechin-3-gallate encapsulated in chitosan nanoparticles on human melanoma cell growth both in vitro and in vivoNanomedicine10201416191626
- M.PrabaharanReview paper: chitosan derivatives as promising materials for controlled drug deliveryJ Biomater Appl232008536
- M.A.KhanM.ZafaryabS.H.MehdiI.AhmadM.M.RizviCharacterization and anti-proliferative activity of curcumin loaded chitosan nanoparticles in cervical cancerInt J Biol Macromol932016242253
- V.Zamora-MoraM.Fernandez-GutierrezA.Gonzalez-GomezB.SanzJ.S.RomanG.F.Goyaet al.Chitosan nanoparticles for combined drug delivery and magnetic hyperthermia: from preparation to in vitro studiesCarbohydr Polym1572017361370
- A.DubeJ.A.NicolazzoI.LarsonChitosan nanoparticles enhance the plasma exposure of (−)-epigallocatechin gallate in mice through an enhancement in intestinal stabilityEur J Pharm Sci442011422426
- M.HassanH.WatariA.AbuAlmaatyY.OhbaN.SakuragiApoptosis and molecular targeting therapy in cancerBiomed Res Int201415084
- V.C.GeorgeG.DellaireH.P.RupasinghePlant flavonoids in cancer chemoprevention: role in genome stabilityJ Nutr Biochem452016114
- E.LecumberriY.M.DupertuisR.MiralbellC.PichardGreen tea polyphenol epigallocatechin-3-gallate (EGCG) as adjuvant in cancer therapyClin Nutr322013894903
- N.KhanD.J.BharaliV.M.AdhamiI.A.SiddiquiH.CuiS.M.Shabanaet al.Oral administration of naturally occurring chitosan-based nanoformulated green tea polyphenol EGCG effectively inhibits prostate cancer cell growth in a xenograft modelCarcinogenesis352014415423
- N.KhanH.MukhtarDietary agents for prevention and treatment of lung cancerCancer Lett3592015155164
- N.YoshiokaY.HiasaM.ChoY.KitahoriK.HiraoN.Konishiet al.Effect of polyphenon-60 on the development of renal cell tumors in rats treated with N-ethyl-N hydroxyethylnitrosamineCancer Lett13619997982
- A.SznarkowskaA.KosteckaK.MellerK.P.BielawskiInhibition of cancer antioxidant defense by natural compoundsOncotarget820171599616016
- F.RizziV.NaponelliA.SilvaA.ModernelliI.RamazzinaM.Bonaciniet al.Polyphenon E(R), a standardized green tea extract, induces endoplasmic reticulum stress, leading to death of immortalized PNT1a cells by anoikis and tumorigenic PC3 by necroptosisCarcinogenesis352014828839
- L.N.FixM.ShahT.EfferthM.A.FarwellB.ZhangMicroRNA expression profile of MCF-7 human breast cancer cells and the effect of green tea polyphenon-60Cancer Genomics Proteomics72010261277
- B.J.PhilipsC.H.CoyleS.N.MorrisroeM.B.ChancellorN.YoshimuraInduction of apoptosis in human bladder cancer cells by green tea catechinsBiomed Res302009207215
- S.J.KimE.AmankwahS.ConnorsH.Y.ParkM.RinconH.Cornnellet al.Safety and chemopreventive effect of Polyphenon E in preventing early and metastatic progression of prostate cancer in TRAMP miceCancer Prev Res (Phila)72014435444
- M.OzaslanI.D.KaragozI.H.KilicM.E.GuldurEhrlich ascites carcinomaAfr J Biotech10201123752378
- S.VimalS.Abdul MajeedG.TajuK.S.NambiN.Sundar RajN.Madanet al.Chitosan tripolyphosphate (CS/TPP) nanoparticles: preparation, characterization and application for gene delivery in shrimpActa Trop1282013486493
- M.M.TomaykoC.P.ReynoldsDetermination of subcutaneous tumor size in athymic (nude) miceCancer Chemother Pharmacol241989148154
- J.F.EnsleyZ.MaciorowskiH.PietraszkiewiczG.KlemicM.KuKurugaS.Saparetoet al.Solid tumor preparation for flow cytometry using a standard murine modelCytometry81987479487
- R.S.RayB.GhoshA.RanaM.ChatterjeeSuppression of cell proliferation, induction of apoptosis and cell cycle arrest: chemopreventive activity of vanadium in vivo and in vitroInt J Cancer12020071323
- W.S.JuanH.W.LinY.H.ChenH.Y.ChenY.C.HungS.H.Taiet al.Optimal Percoll concentration facilitates flow cytometric analysis for annexin V/propidium iodine-stained ischemic brain tissuesCytometry A812012400408
- S.SinghS.PandeyA.N.BhattR.ChaudharyV.BhuriaN.Kalraet al.Chronic dietary administration of the glycolytic inhibitor 2-deoxy-D-glucose (2-DG) inhibits the growth of implanted Ehrlich’s Ascites tumor in micePLoS ONE102015e0132089
- J.LiangF.LiY.FangW.YangX.AnL.Zhaoet al.Synthesis, characterization and cytotoxicity studies of chitosan-coated tea polyphenols nanoparticlesColloids Surf B Biointerfaces822011297301
- S.KumarR.MeenaP.RajamaniFabrication of BSA-green tea polyphenols-chitosan nanoparticles and their role in radioprotection: a molecular and biochemical approachJ Agric Food Chem64201660246034
- H.l.ZhangSh.WuY.TaoLq.ZangZq.SuPreparation and characterization of water-soluble chitosan nanoparticles as protein delivery systemJ Nanomater20102010-1
- Y.ZhangN.KohlerM.ZhangSurface modification of superparamagnetic magnetite nanoparticles and their intracellular uptakeBiomaterials23200215531561
- Y.PanY.J.LiH.Y.ZhaoJ.M.ZhengH.XuG.Weiet al.Bioadhesive polysaccharide in protein delivery system: chitosan nanoparticles improve the intestinal absorption of insulin in vivoInt J Pharm2492002139147
- H.BassionyS.SabetT.A.Salah El-DinM.M.MohamedA.A.El-Ghoret al.Magnetite nanoparticles inhibit tumor growth and upregulate the expression of p53/p16 in Ehrlich solid carcinoma bearing micePloS One92014e111960
- A.BozecA.RuffionM.DecaussinJ.AndreM.DevonecM.Benahmedet al.Activation of caspases-3, -6, and -9 during finasteride treatment of benign prostatic hyperplasiaJ Clin Endocrinol Metab9020051725
- E.C.StuartM.J.ScandlynR.J.RosengrenRole of epigallocatechin gallate (EGCG) in the treatment of breast and prostate cancerLife Sci79200623292336
- T.BorgovanJ.P.BellistriK.N.SlackL.KopelovichM.DesaiA.K.JoeInhibition of BCL2 expression and activity increases H460 sensitivity to the growth inhibitory effects of polyphenonE J Exp Ther Oncol82009129144
- M.ShimizuA.DeguchiJ.T.LimH.MoriwakiL.KopelovichI.B.Weinstein(−)-Epigallocatechin gallate and polyphenon E inhibit growth and activation of the epidermal growth factor receptor and human epidermal growth factor receptor-2 signaling pathways in human colon cancer cellsClin Cancer Res11200527352746
- T.MiyashitaS.KrajewskiM.KrajewskaH.G.WangH.K.LinD.A.Liebermannet al.Tumor suppressor p53 is a regulator of bcl-2 and bax gene expression in vitro and in vivoOncogene9199417991805
- W.Y.FuJ.P.ChenX.M.WangL.H.XuAltered expression of p53, Bcl-2 and Bax induced by microcystin-LR in vivo and in vitroToxicon462005171177
- G.I.EvanK.H.VousdenProliferation, cell cycle and apoptosis in cancerNature41168352001342348
- P.RoyN.NigamJ.GeorgeS.SrivastavaY.ShuklaInduction of apoptosis by tea polyphenols mediated through mitochondrial cell death pathway in mouse skin tumorsCancer Biol Ther8200912811287
- M.H.LeeD.W.HanS.H.HyonJ.C.ParkApoptosis of human fibrosarcoma HT-1080 cells by epigallocatechin-3-O-gallate via induction of p53 and caspases as well as suppression of Bcl-2 and phosphorylated nuclear factor-kappaBApoptosis1620117585
- A.M.RoyM.S.BaligaS.K.KatiyarEpigallocatechin-3-gallate induces apoptosis in estrogen receptor-negative human breast carcinoma cells via modulation in protein expression of p53 and Bax and caspase-3 activationMol Cancer Ther4120058190
- J.W.GuK.L.MakeyK.B.TuckerE.ChincharX.MaoI.Peiet al.EGCG, a major green tea catechin suppresses breast tumor angiogenesis and growth via inhibiting the activation of HIF-1alpha and NFkappaB, and VEGF expressionVasc cell520139
- T.KondoT.OhtaK.IguraY.HaraK.KajiTea catechins inhibit angiogenesis in vitro, measured by human endothelial cell growth, migration and tube formation, through inhibition of VEGF receptor bindingCancer Lett1802002139144
- S.LamyD.GingrasR.BeliveauGreen tea catechins inhibit vascular endothelial growth factor receptor phosphorylationCancer Res6222002381385
- Y.K.LeeN.D.BoneA.K.StregeT.D.ShanafeltD.F.JelinekN.E.KayVEGF receptor phosphorylation status and apoptosis is modulated by a green tea component, epigallocatechin-3-gallate (EGCG), in B-cell chronic lymphocytic leukemiaBlood1042004788794
- L.BaloghS.S.NigavekarB.M.NairW.LesniakC.ZhangL.Y.Sunget al.Significant effect of size on the in vivo biodistribution of gold composite nanodevices in mouse tumor modelsNanomedicine32007281296
- L.ZhangF.X.GuJ.M.ChanA.Z.WangR.S.LangerO.C.FarokhzadNanoparticles in medicine: therapeutic applications and developmentsClin Pharmacol Ther832008761769
- Y.KatoH.OnishiY.MachidaApplication of chitin and chitosan derivatives in the pharmaceutical fieldCurr Pharm Biotechnol42003303309
- W.R.ChenM.KorbelikK.E.BartelsH.LiuJ.SunR.E.NordquistEnhancement of laser cancer treatment by a chitosan-derived immunoadjuvantPhotochem Photobiol812005190195
- J.LiangF.LiY.FangW.YangX.AnL.Zhaoet al.Cytotoxicity and apoptotic effects of tea polyphenol-loaded chitosan nanoparticles on human hepatoma HepG2 cellsMater Sci Eng C Mater Biol Appl362014713