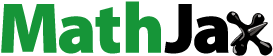
Abstract
In this study the effect of inclusion of nano-metakaolin (NMK) to ordinary Portland cement (OPC) on the hydration characteristics and microstructure of hardened OPC–NMK pastes was studied. The OPC–NMK blends were prepared by the partial substitution of OPC by NMK (4, 6, 10 and 15 weight %). The fresh pastes were made using an initial water/solid (W/S) ratio of 0.27 by weight and then hydrated for various time intervals. At the end of each hydration time, the hardened blended cement pastes were tested for compressive strength, free lime content, combined water content, X-ray diffraction (XRD) analysis, differential scanning calorimetry (DSC) and scanning electron microscopy (SEM). The compressive strength results revealed that the inclusion of nano-metakaolin into OPC improved the mechanical properties of NMK–OPC pastes during almost all ages of hydration, especially with the paste containing 10 wt% NMK. The compressive strength values obtained for OPC paste blended with 4% silica fume (SF) and 6% NMK are comparable to those of the neat OPC paste. The DSC thermograms and XRD diffractograms obtained for some selected hardened pastes indicated the formation of amorphous calcium silicate hydrates, calcium sulfoaluminate hydrates, calcium aluminate hydrate and calcium hydroxide. SEM micrographs showed the formation of a dense microstructure for the hardened OPC–NMK and OPC–NMK-SF pastes as compared to the neat OPC paste after 90 days of hydration.
Introduction
Studies have been made to enhance the use of cementitious materials such as pozzolans as a partial substituent of Portland cement. Utilization of these materials for such purposes not only eliminates the problems of Portland cement but also results in high efficiency cement production [Citation1,Citation2]. Pozzolana was defined as siliceous/aluminous materials, which reacts chemically with calcium hydroxide (CH) in the presence of water to form compounds that possess cementitious properties. Fly ash, rice husk ash, silica fume and slag in addition to the calcined clays in the form of metakaolin are examples of pozzolanic substances [Citation3,Citation4].
The thermal activation process or de-hydroxylation of kaolin at a specific temperature, leads to the breakdown or partial breakdown of the crystal lattice structure, forming a phase transition (metakaolin) which has highly disordered and amorphous characters with pozzolanicity. The exposure duration to temperature above the de-hydroxylation temperature promotes recrystallization to form mullite and hence loss in pozzolanicity [Citation5,Citation6]. A small part of AlO6 octahedra is maintained, while the rest is transformed into much more reactive tetra- and penta-coordinated units [Citation7]. When nano-metakaolin particles are used as cementitious materials, various improvements can be attained, thereby leading to improved permeability and strength. The nano-metakaolin particles act as nuclei of hydration, possess pozzolanic activity, and can fill the voids in the cement matrix. Also the large surface area of nano-particles and their abundance due to their small size, can facilitate the chemical reactions necessary to produce a dense structure with more calcium silicate hydrates (CSH) and less calcium hydroxide contents [Citation8–Citation[9]Citation[10]Citation11].
The use of MK as a partial cement replacement material up to 10–15% in mortar and concrete leads to improvement in the pore structure and high resistance to the transportation of water, the aggressive action of organic acids and diffusion of harmful ions which lead to degradation of the matrix [Citation12Citation[13]Citation[14]Citation15]. The effects of high temperatures up to 800 °C on the mechanical properties and microstructure of OPC containing nano-metakaolin (NMK) were studied. NMK was prepared by thermal activation of nano-kaolin clay at 750 °C for 2 h. It was found that after an initial increase in compressive strength at 250 °C for the mortar specimens, the strength decreased considerably at higher temperatures [Citation16]. The mechanical and durability properties of high performance metakaolin (MK) and silica fume (SF) concretes and their microstructure characteristics were studied [Citation14].
The object of this study is to investigate the hydration characteristics of hardened OPC pastes blended with different amounts of NMK and NMK + SF. This was done via determination of compressive strength, chemically combined water and free lime contents at different ages of hydration. In addition, phase composition was examined using XRD and DSC.
Experimental
Ordinary Portland cement (OPC) used in this investigation was obtained from South Valley Cement Company, Egypt, with Blaine surface area of 2945 cm2 g−1. Its chemical oxide composition is given in . Nano-kaolin was supplied from Middle East Mineralogical (MEMCO), Egypt. The nano-kaolin was fired at 750 °C for 3 h to obtain nano-metakaolin (NMK) with a Blaine surface area of 1.8 × 104 cm2 g−1. Its chemical composition before and after burning is given in . The microstructure of nano-metakaolin (NMK) was studied by means of scanning electron microscopy (SEM) and transmission electron microscopy (TEM) in an earlier publication [Citation17]. Condensed silica fume (SF), which is a by-product of silicon or ferrosilicon alloy industries, was obtained from ferro-silicon company, Kom-Ombo, Egypt. Silica fume particles are spherical and have an average diameter of about 0.1 μm. It consists of 99% amorphous silica with a specific surface area of 20 × 104 cm2 g−1. These characteristics account for the substantial pozzolanic activity of silica fume in terms of both its capacity of binding lime and rate of hydration reaction.
Table 1 Chemical oxide composition of OPC (mass %).
Table 2 Major oxide composition of nano-kaolin (mass %) before and after burning.
Different blended cement pastes were prepared using W/S ratio of 0.27; only 0.1 wt% superplasticizer was added in the case of blended cements containing 10% and 15% NMK (mixes M3 and M4) and 6% NMK + 4% SF (mix MS) to maintain the same W/S ratio. Each paste was prepared by mixing the dry mixture with the required amount of water for about 3 min. After complete mixing, the resultant paste was molded into cubic specimens by using 1 inch cubic molds. Then the specimens were cured with their molds at 100% relative humidity for 24 h. The cubic specimens were removed from their molds and then cured under water at room temperature for different time intervals of 3, 7, 14, 28 and 90 days. illustrates the sample designation and their compositions.
Table 3 Compositions of different mixes and their designation.
At each time interval, three cubes of each hardened cement paste were subjected to compressive strength test and the average value was recorded. This was accomplished using a Ton-industrie machine (West Germany) for maximum load of 60 tons. The resulting crushed specimens of the hardened cement pastes were ground and the hydration reaction was stopped [Citation18]. The samples were then dried at 80 °C for 3 h in CO2-free atmosphere.
Kinetics of hydration reaction were studied by the determination of chemically combined water and free lime contents at different ages of hydration using the ground dried samples. The chemically combined water content, (Wn,%) , was determined by the ignition loss test at 1000 °C for 1 h. Duplicate measurements were carried out for each sample and the mean value was recorded.where Wo = dried sample mass and Wi = ignited sample mass.
The free lime content, CaO(%), was determined by using the glycerol/ethanol extraction method and the mean value of the two independent determinations was recorded [Citation19].
Phase composition of the formed hydrates was investigated for some selected hydrated samples using X-ray diffraction analysis (XRD) and differential scanning calorimetry (DSC). XRD analysis was performed using cobalt target (λ = 0.17889 nm), and nickel filter under working conditions of 40 kV and 40 mA. DSC technique runs were conducted using a Shimadzu DSC-50 thermal analyzer at a heating rate of 20 °C min−1. The sample chamber was purged with nitrogen at a flow rate of 30 cm3 min−1.
Results and discussion
Compressive strength
The results of compressive strength values obtained for the hardened pastes made of neat OPC (Mo) and OPC blended with different amounts of NMK and SF (mixes M1–M4 and MS) are shown in . Evidently, the values of compressive strength of the neat OPC paste (Mo) increase continuously with increasing age of hydration up to the final age of hydration (90 days). This increase is mainly attributed to the hydration of OPC with the formation of hydration products which have high hydraulic properties (mainly as CSH) which act as strong binding centers. For OPC pastes blended with different amounts of NMK and NMK + SF, the same trend was observed. For the pastes made of mixes M1, M2 and M3, the compressive strength values were found to be higher than those obtained for the neat OPC paste (Mo) during almost all ages of hydration. This increase in the strength is mainly attributed to the pozzolanic reaction of the free calcium hydroxide (CH), liberated from Portland cement hydration, with nano-metakaolin to form excessive additional amounts of hydration products, mainly as CSH gel as well as crystalline CSH hydrates; these hydrates serve as micro-fills which lead to a reduction in the total porosity leading to an increase in the total contents of binding centers in the specimens; consequently, an increase in the compressive strength values was observed [Citation20,Citation21].
Fig. 1 Compressive strength values versus age of hydration for the hardened neat OPC paste and the different blended cement pastes.
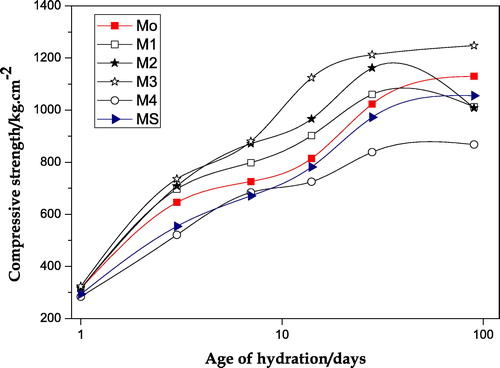
However, for the pastes containing 4% and 6% NMK (mixes M1 & M2) a slight decrease in compressive strength was observed at the later age of hydration (90 days).This decrease may be attributed to a sort of stabilization, via crystallization, of the formed hydrates at later age of hydration.
The paste made of Mix M3 (90% OPC + 10% NMK) possesses the highest strength values at all hydration ages as compared to the other pastes containing 0%, 4%, 6% and 15% NMK. The notable increase in the values of compressive strength for the OPC pastes containing different amounts of NMK may be attributed to the following mechanism: when water is added to each OPC–NMK mixture, free calcium hydroxide liberates as a result of initial hydration of OPC fraction, the silica and alumina of NMK go into solution quickly and react to form secondary hydration products (mainly as calcium silicate hydrate) together with formation of hexagonal calcium aluminate hydrate (mainly as C4AH13) which are precipitated as soon as saturation is approached [Citation22]. For hardened paste made of mix M4 (85% OPC + 15% NMK) the results of compressive strength indicate relatively low strength values than those obtained for all of the tested mixes, . The slight decrease in the strength values of the hardened pastes made of mixes having higher percentages of NMK (such as 15% NMK) may be attributed to the presence of residual amounts of NMK which act as a filler in the hardened OPC–NMK pastes [Citation12,Citation17]. For the hardened OPC paste containing 6% NMK and 4% SF (mix MS), the values of compressive strength are comparable with those of neat OPC paste.
Chemically combined water and free lime contents
The results of chemically combined water content for all pastes investigated, neat OPC, OPC–NMK and OPC–NMK–SF, are given in . As shown in the figure the values of combined water content increases with increasing age of hydration for all of the hardened cement pastes made of all mixes up to the final age of hydration (90 days). This increase can be attributed to the progress of hydration reaction. Also the results indicate a fast hydration reaction takes place from the time of mixing up to one day of hydration. After that, the initially formed hydration products shield the cement grains leading to a slower rate of hydration reaction (7–14 days); this is followed by a gradual increase in the rate of hydration up to 90 days. Evidently, the values of combined water content obtained for most of blended cement pastes are high compared with those of neat OPC paste (Mo), and the highest for M4 and MS mixes up to 28 days of hydration.
Fig. 2 Chemically combined water content versus age of hydration for the hardened neat OPC paste and the different blended cement pastes.
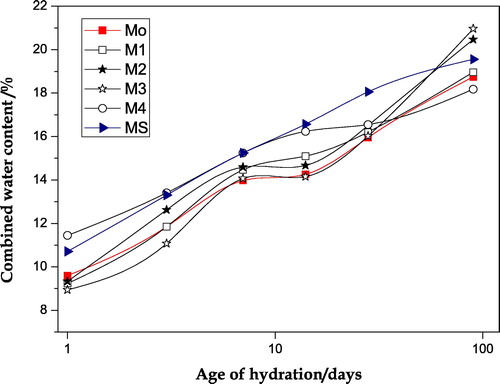
The values of the free lime content obtained for the various investigated cement pastes (Mo, mixes M1–M4 and MS) are shown in .The free lime content increases continuously with increasing age of hydration up to the final ages of hydration for the neat OPC paste (Mo), indicating the continuous liberation of free portlandite (CH) from the hydration of OPC. On the other hand the observed free lime contents for all OPC–NMK and OPC–NMK–SF blended pastes show a slight increase up to 28 days followed by a marked decrease after 90 days. Evidently, the values of free lime content observed for these blended cement pastes represent a net result of two opposite processes; first an increase in the amount of free lime which liberated from hydration of Portland cement and second the consumption of these liberated lime via the pozzolanic reaction with NMK (mixes M1–M4) or NMK and SF (mix MS). As a result of these two processes the values of free lime contents obtained for all admixed OPC pastes are lower than those of the neat OPC paste especially at later ages of hydration (90 days). Also, the increase in the percentage of pozzolanic material replacement (NMK & SF) in OPC pastes is accompanied by a marked decrease in the values of free lime content [Citation17].
Phase composition and microstructure
X-ray diffraction (XRD) analysis
X-ray diffractograms obtained for the hardened cement pastes made of neat OPC (mix Mo) and OPC containing 10% NMK (mix M3) after 1, 28 and 90 days of hydration are shown in and , respectively. As shown in the main hydration products obtained from hydration of neat OPC paste are microcrystalline calcium silicate hydrates (CSH) and portlandite (CH); the peak characteristics for unhydrated parts of C3S and β-C2S grains could also be distinguished as well as quartz (Q) and calcium carbonate. Obviously, the results of show that the intensities of the peaks characterizing C3S and β-C2S decrease with increasing age of hydration; meanwhile, the intensities of the peaks characterizing the hydration products, mainly as calcium silicate hydrates (CSH), increase with increasing age of hydration up to the final age of hydration (90 days) as the result of the progress of hydration reaction. However, the intensities of the peak characteristic for the free calcium hydroxide showed a slight decrease with increasing age of hydration. The decrease in the intensities of the peaks characterizing CH is attributed to the decrease in the degree of crystallinity of the formed CH and not to a decrease in its amount, since the results of free lime obtained by solvent extraction measurement (represent both amorphous and crystalline parts) indicate a continuous increase in its amount with increasing age of hydration up to 90 days. X-ray diffractograms obtained for the hardened OPC–NMK paste of mix M3 display nearly the same hydrated phases distinguished as in the case of the neat OPC (Mo), but with higher amorphous nature (). As shown in , the intensities of the peaks characteristic for CH increase gradually during the early ages of hydration for the OPC–NMK paste containing 10% NMK up to 28 days; this increase is attributed to the amount of CH liberated from OPC hydration exceeds that are consumed by the pozzolanic reaction with NMK. On the other hand, there is a corresponding decrease in the peak intensities characteristics for the anhydrous calcium silicate compounds during the early age of hydration as a result of the progress of hydration reaction. At later age of hydration (90 days), the peaks of the unhydrous cement compounds are almost vanished and the intensities of the peaks characterizing CH decrease. At later age of hydration, the amount of CH consumed in the pozzolanic reaction with NMK exceeds the amount liberated during OPC hydration and so there will be a net decrease in the amount of CH leading to a decrease in the intensities of the peaks characterizing CH after 90 days of hydration.
Differential scanning calorimetry (DSC)
The DSC thermograms obtained for the specimens made of neat OPC and OPC containing 10% NMK hydrated for 1, 28 and 90 days of hydration are shown in and , respectively. The DSC thermograms of the neat OPC paste (Mo) indicate three endothermic peaks at 90–110 °, 490–510 ° and 718–750 °C, . The first endotherm located at 90–110 °C is mainly due to the removal of residual free water as well as dehydration of the sulfoaluminate hydrates and the amorphous part of calcium silicate hydrates (CSH). The enthalpies of this endotherm are 10.32, 9.24, and 8.35 J/g after 1, 28 and 90 days of hydration, respectively. This slight decrease in the value of the enthalpy of this endotherm with increasing age of hydration is explained in terms of the decrease in free water content and not the decrease in CSH content; since the compressive strength values for these hardened pastes increase with increasing the age of hydration, which mean an increase in the amount of the formed hydrates (mainly as amorphous CSH). The second peak located at 490–510 °C, which represents the major weight loss, is mainly related to the decomposition of portlandite (CH) [Citation23]. The intensity of this endotherm increases with increasing age of hydration; this increase is attributed to the increase in the amount of formed free lime. The enthalpy of this endotherm increases from 21.32 J/g to 31.93 J/g with increasing age of hydration from 1 to 28 days, respectively; these values indicate an increase in the amount of portlandite. After 90 days of hydration the enthalpy of this endotherm decreases to 26.41 J/g., this decrease is explained in terms of decrease in the degree of crystallinity of the formed portlandite as mentioned previously from the results of X-ray analysis. Finally the third endotherm located at 718–750 °C is due to the decomposition of calcium carbonate. The enthalpy of this endotherm gives nearly the same value (3.20 J/g) after 1 and 28 days and becomes zero after 90 days, these values account for the degree of carbonation of the hardened specimens [Citation24,Citation25].
Fig. 7 DSC thermograms of hardened OPC paste containing 10% NMK (mix M3) at different ages of hydration.
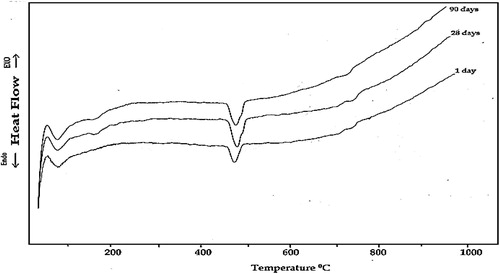
The thermograms obtained for the OPC–NMK blended cement pastes containing 10% NMK (mix M3) indicate the same three endothermic peaks obtained for the neat OPC paste (Mo) as shown in . The first peak located at 90–105 °C is mainly due to the removal of residual free water as well as dehydration of sulfoaluminate hydrates and the amorphous part of calcium silicate hydrates (CSH). The values of enthalpy of this endotherm are 10.86, 10.68 and 11.08 J/g after 1, 28 and 90 days of hydration, respectively. These values are explained in terms of increasing amounts of the formed hydrates (mainly as tobermorite like CSH) but with high amorphous character, since the compressive strength data showed a continuous increase with increasing age of hydration (up to 90 days).The second peak is located at 490–510 °C, which represents the major weight loss in the decomposition of portlandite (CH). The enthalpy of this endotherm decreases from 12.39 to 8.24 and 6.28 J/g with increasing age of hydration from 1 to 28 and 90 days, respectively. This is due to the consumption of free CH by the pozzolanic reaction with NMK. The third endotherm located at 718–750 °C is due to the decomposition of calcium carbonate. The enthalpy of this endotherm is 1.48, 2.29 and 0.62 J/g after 1, 28 and 90 days, respectively; these values account for the degree of carbonation of the hardened specimens.
Conclusions
On the basis of the results obtained in this investigation, the following conclusions could be derived:
1. | The hardened pastes made of OPC–NMK blends containing 4%, 6% and 10% NMK showed higher compressive strength values as compared to those of the neat OPC paste. The optimum substitution of OPC by NMK is 10%. |
2. | The values of combined water content obtained for all OPC–NMK blended cement pastes are higher than those of the neat OPC paste. |
3. | The values of the free lime content of all OPC–NMK blended cement pastes are lower than those of the neat OPC paste especially at later ages of hydration. |
4. | XRD diffractograms and DSC thermograms obtained for all the investigated cement pastes indicate the formation of nearly amorphous calcium silicate hydrates, calcium sulfoaluminate hydrates, calcium aluminate hydrates, calcium hydroxide and calcium carbonate. |
Notes
Peer review under responsibility of Housing and Building National Research Center.
References
- J. Davidovits, M. Moukwa, S.L. Sarkar, K. Luke, Geopolymer cement to minimize carbon-dioxide greenhouse warming, ceramic transaction cement-based material: present, future, environmental Aspect, Westerville: The American Ceramic Society, 1993 37 165–181.
- N.J.SaikiaaP.SenguptaaP.K.GogoibP.C.BorthakuraCementitious properties of metakaolin–normal Portland cement mixture in the presence of petroleum effluent treatment plant sludgeCem. Concr. Res.32200217171724
- B.B.SabirS.WildJ.BaiMetakaolin and calcined clay as pozzolans for concreteCem. Concr. Compos.232001441454
- M.SinghM.GargReactive pozzolana from Indian clays—their use in cement mortarsCem. Concr. Res.36200619031907
- R.A.SayanamA.K.KalsotraS.K.MehtaR.S.SingG.MandalStudies on thermal transformations and pozzolanic activities of clay from Jammu region (India)J. Therm. Anal. Calorim.3519899106
- G.Badogiannis KakaliG.DimopoulouE.ChaniotakisS.TsivilisMetakaolin as supplementary cementitious materials, optimization of kaolin to metakaolin conversionJ. Therm. Anal. Calorim.812005457462
- S.A.Abo-El-EneinM.HeikalM.S.AminH.H.NegmReactivity of dealuminated kaolin and burnt kaolin using cement kiln dust or hydrated lime as activatorsConstr. Build. Mater.47201314511460
- M.S.MorsyH.AglanDevelopment and characterization of nano-structuredperlite- cementitious surface compoundsJ. Mater. Sci.422007196202
- J.ShihT.ChangT.HsiaoEffect of nanosilica on characterization of Portland cement compositeMater. Sci. Eng. A4242006266274
- S.S.SheblL.AllieM.S.MorsyH.A.AglanMechanical behavior of activated nano silicate filled cement bindersJ. Mater. Sci.44200916001606
- X. He, X. Shi, Chloride permeability and microstructure of Portland cement mortars incorporating nanomaterials, In: Transportation Research Record: J. Transp. Res. Board, No. 2070, Transportation Research Board of the National Academies, Washington, DC, 2008 13–21.
- J.PeraS.RolsM.ChabannetJ.AmbroiseInfluence of the cement type on the resistance of concrete to an agricultural environmentAm. Concr. Soc.1998419430
- S. Martin, Metakaolin and its contribution to the acid resistance of concrete, In: international symposium on concrete for a sustainable Agriculture, Stavanger, Norway, 1997, 21–29.
- C.S.PoonS.C.KouL.LamCompressive strength, chloride diffusivity and pore structure of high performance metakaolin and silica fume concreteConstr. Build. Mater.202006858865
- Z.LiZ.DingProperty improvement of Portland cement by incorporating with metakaolin and slagCem. Concr. Res.332003579584
- M.S.MorsyY.A.Al-SalloumH.AbbasS.H.AlsayedBehavior of blended cement mortars containing nano-metakaolin at elevated temperaturesConstr. Build. Mater.352012900905
- S.A. Abo-El-Enein, M.S. Amin, F.I. El-Hosiny, S. Hanafi, T.M. ElSokkary, M.M. Hazem, Pozzolanic and hydraulic activity of nano-metakaolin, HBRC, Fifth International Conference on nano-technology in construction, 2013.
- H.El-DiamononyA.S.KhalilStudies on artificial pozzolanic cement: physico-chemical propertiesZem-Kalk-Gips3461981312314
- S.A.Abo-El-EneinM.DiamonS.OhsawaR.KondoHydration of low porosity slag lime pastesCem. Concr. Res.421974299312
- S.A.Abo-El-EneinR.AbbasY.E.EzzatPropiedades y duyabilidad de los cemento sadicionados con metacaolin properties and durability of metakaolin blended cementsMater. Construction6029920102135
- M.S.MansourE.KadriS.KenaiM.GhriciR.BennaceurInfluence of calcined kaolin on mortar propertiesConstr. Build. Mater.25201122752282
- M.S.AminS.A.Abo-El-EneinAbdelRahmanK.A.Aand AlfalousArtificial pozzolanic cement pastes containing burnt clay with and without silica fumeJ. Therm. Anal. Calorim.107201211051115
- S.M.A.El-GamalF.S.HashemM.S.AminThermal resistance of hardened cement pastes containing vermiculite and expanded vermiculiteJ. Therm. Anal. Calorim.1092012217226
- M.S.MorsyA.F.GalalS.A.Abo-El-EneinEffect of temperature on phase composition and microstructure of artificial pozzolana-cement pastes containing burnt Kaolinite ClayCem. Concr. Res.288199811571163
- A.HidalgoJ.L.GarciaM.C.AlonsoL.FernandezC.AndradeMicrostructure development in mixes of calcium aluminate cement with silica fume or fly ashJ. Therm. Anal. Calorim.962009335345