Abstract
Vanadium is a contaminant of crude oil that released into the atmosphere through burning of fossil fuels. The mechanism by which it exerts toxic influences had not been fully elucidated in African giant rat (AGR). This study investigates the mechanisms of sodium metavanadate (SMV) induced oxidative stress in AGR. A total of 24 adult male AGR weighing 600–850 g were used. Animals were randomly divided into six groups. Groups 1, 3 and 5 served as control while groups 2, 4 and 6 were treated with intraperitoneal 3 mg/kg body weight of SMV for 3, 7 and 14 days, respectively. Serum, brain, liver, testes, kidneys, spleen and lungs were harvested for biochemical assays. SMV induced significant increase in malondialdehyde, hydrogen peroxide, sulfhydryl (total thiol) and protein carbonyl levels but decreased non-protein thiol levels in tissues accessed. A significant decrease was observed in glutathione-S-transferase (GST), superoxide dismutase (SOD), reduced glutathione (GSH) and glutathione peroxidase (GPx) levels in SMV treated rats compared to controls. Serum myeloperoxidase, xanthine oxidase and Advanced Oxidative Protein Products (AOPP) were markedly increased while nitrous oxide levels were significantly decreased in all treated groups. SMV exposure to AGR induced oxidative stress through generation of reactive oxygen species (ROS) and depletion of the antioxidant defence system. These conditions could become severe with prolonged exposure.
1 Introduction
The human population has been on a steady increase in third world countries [Citation1], resulting in destructive human activities such as increased exploitation of minerals leading to pollution of confined environments [Citation1,Citation2].
Air pollution is a complex mixture of gases, particulate matter, endotoxins, organic compounds and metals [Citation3] and can result from combustion of fossil fuel [Citation1] as seen in the Nigeria Niger Delta [Citation4]. Increased industrial use of metals such as vanadium results in environmental pollution with chronic toxicity effects [Citation5]. As a result, the risks of occupational and environmental exposure of vanadium to humans are of great concern [Citation5] especially in Nigeria with recent increase in exploitation of minerals [Citation4].
Vanadium, a metal in the first transition series, is essential for mammals [Citation6,Citation7]. Vanadium was named in honor of Vanadis, the goddess of fertility and beauty due to the variety of colours generated by the metal in solution [Citation8], but this compound is also known to be highly toxic [Citation4,Citation7], hence, the paradox between its beauty and death [Citation9].
Over the last five decades, vanadium salts have been used medicinally as antidiabetics, antiseptics, blood tonic as well as antituberculotic agents [Citation9,Citation10]. However, acute exposure to this metal has been reported to cause gastrointestinal disturbances, headache, diarrhea, skin and eye irritations, respiratory tract inflammation [Citation7,Citation10], cardiovascular diseases [Citation11], depletion of lymphoid cells, hepatic and testicular degeneration [Citation7].
As a catalytic metal, vanadium salts induce reactive oxygen species formation [Citation12,Citation13], through generation of free radicals and depletion of antioxidants, including glutathione [Citation14–Citation16]. Schoneich et al. [Citation17] showed that oxidation of several biological related thiols by vanadium generates corresponding thiyl radicals which have the ability to peroxidize lipids [Citation18].
It implies that the ability of vanadium to generate free radicals like hydroxyl radicals in the Fenton-like reaction [Citation19,Citation20] and to decrease concentration of biologically important cellular compounds causes weakness of the antioxidative system rendering cells more vulnerable to oxidative damage [Citation6], which if prolonged could lead to the accumulation of lipid peroxides and tissue injury.
Since many wildlife rodents are exposed to this chemical in oil producing areas of most poor resource setting, emphasis had been on introducing the African giant rats as an experimental animal for toxicological studies [Citation21].
African giant rat (AGR) (Cricetomys gambianus, Waterhouse, 1840) is usually found in Central and West African countries, including Nigeria and is known as a pouched rodent, belonging to the family Muridae, and makes up a group of the order Rodentia [Citation22]. The AGR has been used to detect land mines in Mozambique [Citation23] and diagnosis of tuberculosis in Europe [Citation24]. Usende et al. [Citation21] emphasized the use of this rodent as an experimental model for ecotoxicological research due to its nocturnal and ubiquitous nature, this rodent can assist in knowing the level of animal and human exposure to environmental pollutants.
Therefore, the aim of this study was to evaluate the oxidative stress response and antioxidants profile of AGR following intraperitoneal SMV exposure.
2 Materials and methods
2.1 Chemicals
Sodium metavanadate (vanadium (IV)), potassium hydroxide, reduced glutathione (GSH), trichloroacetic acid, sodium hydroxide, 1, 2 dichloro-4-nitrobenzene (CDNB), thiobarbituric acid (TBA), xylenol orange, sodium hydroxide and hydrogen peroxide (H2O2), N-(1-naphthyl) ethylenediamine dihydrochloride, cyclophosphamide, 5, 5-Dithiobis-(2-nitrobenzoic acid) (DTNB) were purchased from Sigma (St Louis, MO, USA). All other chemicals were of analytical grade and were obtained from British Drug Houses (Poole, Dorset, UK).
2.2 Animal protocol and experimental design
A total of 24 young adult male African giant rats weighing 600–850 g were obtained from local market in Ibadan, Nigeria and used for the research. The animals were kept singly in metal cages in a well-ventilated animal house under controlled light cycle (12 h light/12 h dark), fed with standard commercial pelletized rat feed, fresh groundnut and yam with water ad libitum and were habituated for 14 days before the start of experiment. This choice of fresh groundnut and yam was to mimic the natural nuts and tuber choice of this rodent in the wild. All of the animals received humane care according to the criteria outlined in the Guide for the Care and the Use of Laboratory Animals prepared by the National Academy of Science and published by the National Institute of Health. The ethics regulations were followed in accordance with national and institutional guidelines for the protection of the animals’ welfare during experiments [Citation25].
2.3 Administration of sodium metavanadate (SMV) as vanadium (IV)
All animals were randomly divided into six groups of four animals each as follows:
Group I: Control. African giant rats received intraperitoneal injection of sterile water for 3 days.
Group II: African giant rats treated with intraperitoneal 3 mg/kg body weight of SMV for 3 days.
Group III: Control. African giant rats received intraperitoneal injection of sterile water for 7 days.
Group IV: African giant rats treated with intraperitoneal 3 mg/kg body weight of SMV for 7 days.
Group V: Control. African giant rats received intraperitoneal injection of sterile water for 14 days.
Group VI: African giant rats treated with intraperitoneal 3 mg/kg body weight of SMV for 14 days.
The dose of 3 mg/kg body weight of SMV used was as previously used by Todorich et al. [Citation26] and Usende et al. [Citation4]. The administration of SMV was started with each AGR housed in its cage alone and continued daily over the period of experiment.
2.4 Serum collection
Twenty-four hours after the last treatment, five millilitres (5 mL) of blood were drawn from the retro-orbital venous plexus of each animal into plain tubes before they were sacrificed by quick cervical dislocation. Thereafter, blood was centrifuged at 4000 rpm for 10 min. The clear serum gotten was separated with Pasteur pipettes into another plain tube and stored at −20 °C until the time of biochemical analysis.
2.5 Preparation of microsomal fractions
The liver, kidneys, half hemisphere of brain, testes, lungs, and spleen were carefully dissected out and a portion harvested, weighed and rinsed in 1.15% KCl and thereafter homogenized in aqueous potassium phosphate buffer (0.1 M, pH 7.4). Homogenates were centrifuged at 10,000 g for 20 min to obtain the supernatant post-mitochondrial fraction [Citation27]. These were stored at −20 °C until the time of use for biochemical assays.
2.6 Biochemical assays
The post-mitochondrial fractions from the various organs were used for protein concentration as was described by the Biuret method of Gornal et al. [Citation28]. Nitrite (NO) content was determined according to the method of Olaleye et al. [Citation29]. Hydrogen peroxide (H2O2) generation was assessed by the method of Wolff [Citation30]. Malondialdehyde (MDA) concentration as an index of lipid peroxidation was quantified according to the method described by Farombi et al. [Citation31]. Reduced glutathione (GSH) concentration was determined using the method of Jollow et al. [Citation32]. Superoxide dismutase (SOD) assay was carried out by the method of Misra and Fridovich [Citation33] with slight modification by Oyagbemi et al. [Citation27]. Glutathione peroxidase (GPx) activity was measured by the method of Rotruck et al. [Citation34]. Glutathione-S‑transferase (GST) was estimated by the method of Habig et al. [Citation35] using 1‑chloro‑2,4‑dinitrobenzene as substrate. The activity of Xanthine oxidase (XO) was determined according to methods of Akaike et al. [Citation36]. Myeloperoxidase (MPO) activity was determined by the method of Xia and Zweier [Citation37]. The thiol (Non-protein thiol (NPT)) and sulfhydryl (total thiol (PT)) content was estimated by the method of Ellman [Citation38]. Advanced oxidation protein products (AOPP) level was determined according to the method of Kayali et al. [Citation39]. Protein carbonyl (PCO) was measured using the method of Reznick and Packer [Citation40].
2.7 Statistical analysis
The three SMV treated and their control groups were compared using ANOVA test. To determine continuous exposure effects from the different time points, statistics were performed using Graph Pad Prism version 5. All data generated were evaluated for statistical significance using one-way ANOVA with Tukey multiple post-test for comparison.
3 Results
3.1 Brain
The brain supernatant obtained from AGRs groups which received SMV intraperitoneally for 3, 7 and 14 days showed statistically significant increased oxidative stress markers including MDA, PCO, H2O2 and PT (–, levels). However, a statistical significant decrease was observed in same brain tissue in NPT ( levels). When comparison was done between SMV treated groups, a slight decrease was observed in MDA levels at day 7 compared to day 3. However, at day 14, there was an increase above what was observed at days 3 and 7. For H2O2, a time dependent increase was observed but a decrease at day 14 of PT and PCO levels compared to days 3 and 7 was recorded. For the brain antioxidant markers, our results showed a decrease in GSH (), SOD, GPx and GST levels which was time dependent for GPx and (). However, we observed slight increased GSH at day 14 and GST at days 7 and 14 when the SMV treated groups were compared.
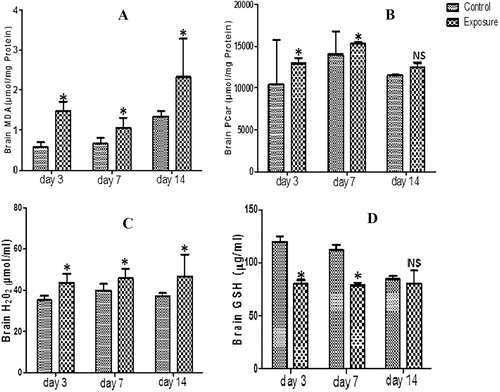
Table 1 Brain anti-oxidant enzyme profile, total thiol (PT) and non-protein thiol (NPT) following vanadium exposure to African giant rat at three different time points.
3.2 Kidney
Our results showed no significant difference in renal GST activity of SMV treated AGR groups compared to their controls. There was a significant decrease in renal SOD, GPx () and GSH () activities observed in SMV treated AGRs compared to their controls, except on day 14 when a significant increase was seen in renal GSH of treated group compared to control. On the other hand, renal NPT decreased significantly while renal PT, MDA, PCO, and H2O2 levels increased significantly in SMV treated AGR groups compared to controls (, –). These findings were time dependent.
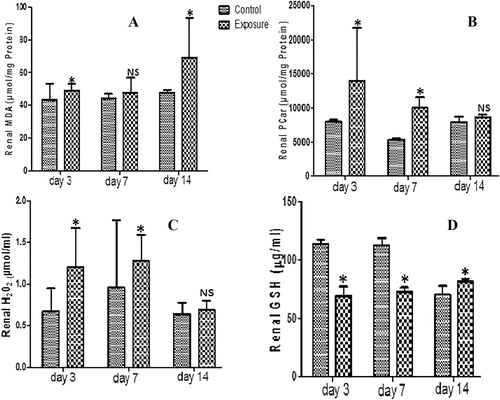
Table 2 Renal anti-oxidant enzyme profile, total thiol (PT) and non-protein thiol (NPT) following vanadium exposure to African giant rat at three different time points.
3.3 Testis
For the testis, our results indicated a decrease in GPx, SOD, GST and GSH antioxidant levels which was time dependent (; ) and was significant only for treated days 3 and 7 for GSH. For the oxidative stress markers, there was increase MDA, PCO, H2O2 and PT generation levels but a decrease NPT levels (–; ). These were statistically significant and time dependent.
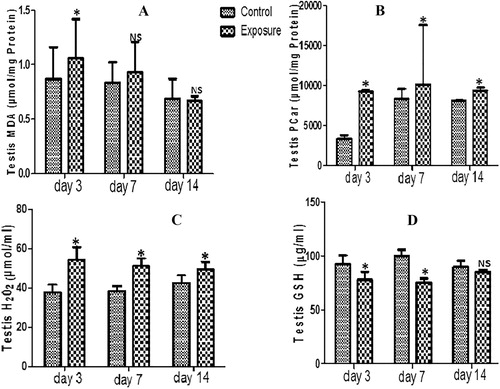
Table 3 Testis anti-oxidant enzyme profile, total thiol (PT) and non-protein thiol (NPT) following vanadium exposure to African giant rat at three different time points.
3.4 Liver
Statistically significant decrease was seen in liver GST level of SMV treated AGR groups (). A statistical significant increase was however seen in MDA, PCO, PT and H2O2 generation levels in same liver tissue compared to their controls (–; ). Furthermore, decreased SOD, GPx and GSH antioxidant levels (; ) and NPT oxidative stress markers () was seen in SMV treated AGR groups compared to their control. Comparing the SMV treated groups alone, a decrease in MDA was observed at day 7 compared to day 3 but with continuous treatment to day 14 an increase above what was observed at day 3 and day 7 was seen (). NPT levels on the other hand increased at day 7 compare to day 3 and day 14 (). A time dependent decrease was seen in PCO, PT, and H2O2 ( and ; ). For the antioxidant enzymes, an increase was observed in day 7 treated AGR compared to day 3 which drop at day 14. The same pattern was seen in SOD.
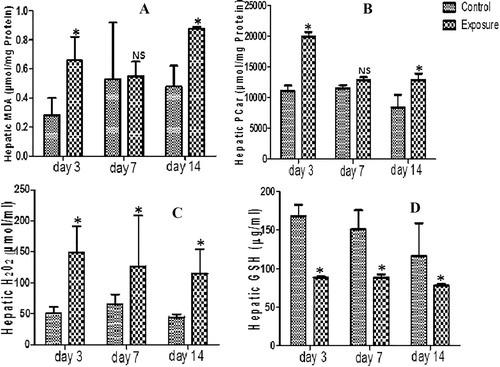
Table 4 Hepatic anti-oxidant enzyme profile, total thiol (PT) and non-protein thiol (NPT) following vanadium exposure to African giant rat at three different time points.
3.5 Spleen
Spleen supernatant of SMV treated AGRs had increased levels of MDA, PT, PCO and H2O2 generation that were not statistically significant compared to their controls (–; ). The increase seen in MDA and PCO and the decrease seen in NPT ( and ; ) were time dependent. However, we observed a decreased H2O2 and PT at day 7 compared to day 3 which later increased at day 14 with the PT higher than what was observed at day 3. The results also indicated a decrease in SOD, GPx, GST and GSH antioxidant levels (; ). This was significant and time dependent. When the antioxidant levels in the treated group were compared, a time dependent decrease of SOD and GPx was observed except in GST level where an increase at day 14 compared to day 3 and day 7 was seen (). For GSH, an increase was observed when day 7 was compared to day 3, but a decrease when was seen day 14 was compared to day 3 and day 7 ().
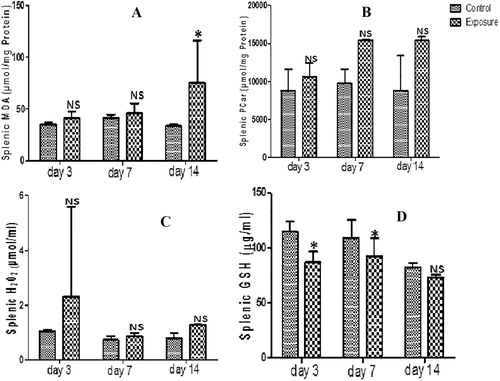
Table 5 Splenic anti-oxidant enzyme profile, total thiol (PT) and non-protein thiol (NPT) following vanadium exposure to African giant rat at three different time points.
3.6 Lungs
Only PCO and H2O2 generation levels showed significant increase in lungs of SMV treated AGR groups compared to their controls ( and ). Although an increase in MDA, PT and a decrease in NPT were observed, these were not significant (; ). Similarly, a non significant decrease in GST, SOD, GPx and GSH antioxidant levels was also seen in SMV treated AGRs compared to their controls (; ). For MDA, the increase observed in treated groups was time dependent but for H2O2 a decrease at day 7 and day 14 was seen compared to day 3. When PT generation in treated groups was compared, an increase at day 7 compared to day 3 was noticed which later decrease at day 14. The same pattern was seen in PCO. The decrease observed in NPT and antioxidant enzyme level was time dependent.
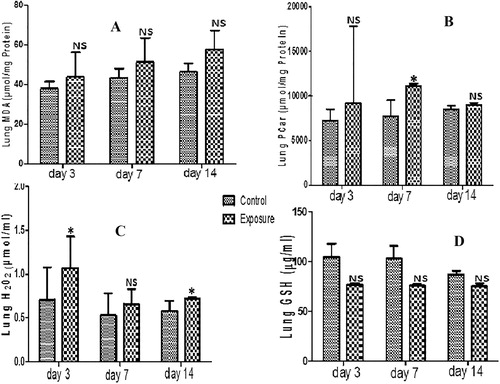
Table 6 Lung anti-oxidant enzyme profile, total thiol (PT) and non-protein thiol (NPT) following vanadium exposure to African giant rat at three different time points.
3.7 Serum
Serum markers of oxidative stress indicated a decrease in NO but increase in MPO, AOPP and XO level which was statistically significant and time dependent (). When the treated groups where compared for MPO, a significant increase in day 7 compared to day 3 was observed, however, at day 14 a decrease compared to day 7 was seen, no difference was seen when day 3 was compared to day 14. For AOPP, a decrease was observed when day 3 was compared to day 7, but at day 14 an increase was seen although not as high as day 3. For XO, there was decrease at day 7 compared to day 3 but at day 14 an increase was observed compared to day 7 which was not as high as day 3 ().
Table 7 Serum markers of oxidative stress following vanadium exposure.
4 Discussion
In the present study, we assessed the antioxidant defence system and markers of oxidative stress in the brain, testes, liver, kidney, spleen, lungs and serum of AGRs exposed to SMV at three different time points. Our results showed that SMV induced ROS and free radical generations as well as depletion of glutathione similar to reports of Younce et al. [Citation13] and Zaporowska [Citation15] in Wister rats.
GSH and GSH related thiols are known to participate in many important biological reactions including protection of cell membranes against oxidative damage [Citation6,Citation41]. It has been established that vanadium generates free radicals and decreases concentration of important biological cellular compounds causing weakness of antioxidant system rendering cells more vulnerable to oxidative damage [Citation6]. Also, GPx and GST are key enzymes that take part in maintaining glutathione homeostasis [Citation27,Citation42]. Together with GSH, these enzymes work to decompose H2O2 and other organic hydroperoxides [Citation43].
In our study, vanadium induced significant changes in the levels of markers of oxidative stress. Specifically, there was increase in MDA, H2O2 and PCO levels following exposure to SMV in all the tissues accessed and this was time dependent. H2O2 has been reported to produce cytotoxic effects to endothelial cells of different organs [Citation27,Citation44]. Our report therefore points to cytotoxicity on endothelial cells of all the different tissues we accessed. Prolonged exposure may lead to accumulation of lipid peroxides causing damage to these tissues. Similar findings have been reported by Scibior et al. [Citation6]. It has been reported also that the presence of endogenous GSH and antioxidant enzymes like GPx and GST eliminates H2O2 and other toxic substances [Citation45]. Interestingly, we reported that these enzymes were depleted with exposure to SMV and this depletion was aggravated with prolong exposure. With depletion of these enzymes, H2O2 elimination was hindered leading to more generation of ROS. Depletion of GSH with SMV exposure is a clear indication of oxidative stress and toxicity. Our report showed a decrease in GST levels in brain, testis and spleen indicative of compromised defensive mechanism.
The time dependent increase in MDA levels in all the tissues assayed will lead to an enhance lipid peroxidation and was probably due to production of superoxide, peroxide and hydroxyl radicals [Citation27,Citation46]. Also, lipid peroxidation is a critical important reaction in toxicological process [Citation6] and it has been reported that lipid hydroperoxides can react with redox metals like V5+ and V4+ producing mutagenic and carcinogenic malondialdehyde, 4-hydroxynonenal and other exocyclic DNA adducts [Citation6,Citation20]. Our report together with that of Scibior et al. [Citation6] confirmed vanadium induced oxidative stress in exposed tissues. Zaporowska and Scibior [Citation47] recorded that chronic vanadium intoxication can lead to damage of liver and kidney. Our finding report is consistent with their work in all tissues. However, our report emphasized the fact that AGR reacted drastically to acute exposure, tried to stabilize at day 7 but continuous exposure aggravates the damage done.
The brain, testis, liver, kidney, spleen and lungs GSH concentration reduced significantly with vanadium exposure and it was time dependent indicating oxidative stress and toxicity associated with vanadium. Increasing the time of exposure also increased the level of toxicity.
Again, we showed a depletion of cellular non-protein thiol pool in all the tissues of AGR accessed following SMV exposure. Similar findings have been reported by Shi et al. [Citation18] after using electron spin resonance (ESR) and ESR spin tapping methodology. Our work for the first time, reported an in vivo study. It has also been reported that GSH-related thiols are known to function directly or indirectly in many important biological reactions including the protection of cell membranes against oxidative damage [Citation18]. The mechanism is by reduction of cellular H2O2 levels via glutathione peroxidase [Citation18], an important detoxification pathway. Since our report showed increase in H2O2 production; when vanadium reacts with H2O2, OH radical was generated through Fenton-like mechanism playing an important role in vanadium toxicity [Citation18], specifically vanadium IV. Therefore, the depletion we reported of tissues non-protein thiol pool by SMV will lead to reduced protection against H2O2, lipid peroxides and other toxic species which accumulate and render the tissues (cells) more vulnerable to oxidative damage [Citation18] and systemic oxidative stress [Citation48]. We however, reported an increase in sulfhydryl (total thiol) level in all tissues accessed and this is indicative of enhanced protein oxidation in these tissues and might be associated with systemic oxidative stress [Citation48].
There was a significant decrease in activities of brain, testis, spleen, kidney, liver and lungs SOD. The decrease in SOD reported may suggest that these organs have no protective and or adaptive mechanism to SMV induced oxidative damage.
Our study also showed increased PC levels in all the tissues accessed. Protein carbonyl assays generally measure any carbonyl in a protein regardless of the source [Citation27,Citation49] and elevation of this marker is an indication of increased oxidation of protein due to oxidative stress [Citation50,Citation51].
In the serum, we showed that SMV exposure induced increased AOPP, MPO and XO but decreased NO level. Together with PCO, elevated levels of AOPP in tissues serve as a prognostic factor for severe cardiovascular disease and progression of renal damage [Citation27,Citation52,Citation53]. Again, the increased serum activity of XO has been associated with oxidative stress through generation of ROS [Citation54] and is also an indirect measurement of serum uric acid level [Citation27]. Meng et al. [Citation55] and Ohashi et al. [Citation56] recently suggested a link between hyperuricaemia and renal failure which is a possibility following SMV exposure.
Serum MPO plays an important role in inflammation and oxidative stress at cellular level by generation of reactive molecules capable of oxidizing lipids and protein of low-density lipoprotein [Citation41,Citation57] and its recognition is a marker of heart failure and cardiac damage [Citation58]. Therefore, elevated levels of this marker as reported in this study may point to the possible effects of SMV in heart failure and cardiac damage.
It has been reported that NO produced from the vascular endothelium helps to maintain a continuous vasodilator tone and this is essential for the regulation of blood flow, blood pressure, platelet aggregation and vasodilation [Citation27,Citation49]. We also observed a reduction of NO in the serum of AGR groups exposed to SMV. The mechanism could be associated with the reduction in the activity of nitric oxide synthases or as a result of depletion of NO by superoxide anion radical [Citation27,Citation49] which could precipitate hypertension and endothelial dysfunction [Citation59].
5 Conclusions
In conclusion, we showed that SMV exposure to AGR induced oxidative stress through generation of reactive oxygen species, depletion of important endogenous and other enzymes and could lead to brain, gonads, lung, splenic, hepatic, cardiac and renal damage; hyperuricaemia as well as precipitation of hypertension. Although, AGR treated groups showed a little adaptation after acute exposure, these conditions became severe with prolonged exposure. There is a need to investigate organ morphological alterations induced in this animal following exposure to vanadium
Competing interests
None.
Acknowledgement
The authors acknowledge Drs. Obasa, AA, Afolabi JM and Ogunpolu BS for technical assistance.
Notes
Peer review under responsibility of Faculty of Veterinary Medicine, Cairo University.
References
- O.O.IgadoJ.O.OlopadeS.K.OnwukaA.C.ChukwudiO.A.DaramolaU.E.AjufoEvidence of Environmental Pollution in caprine brains obtained from a Relatively Unindustrialized Area in NigeriaAfr J Biomed Res112008305309
- J.O.OlopadeS.K.OnwukaD.AdejumoA.A.LadokunAnalysis of some industrial metals and ions in the cerebral cortex of goats in NigeriaNig Vet J2620055155
- L.Calderon-GarciduenasR.R.MaronpotR.Torres-JardonC.Henriquez-RoldanR.SchoonhovenH.Acuna-AyalaDNA damage in nasal and brain tissues of canines exposed to air pollution is associated with evidence of chronic brain inflammation and neurodegenerationToxicol Pathol312003524538
- I.L.UsendeD.F.LeitnerE.NeelyJ.R.ConnorJ.O.OlopadeThe deterioration seen in myelin related morpho-physiology in vanadium exposed rats is partially protected by concurrent iron deficiencyNiger J Physiol Sci3120161122
- J.L.DomingoDevelopmental Toxicity of Metal Chelating AgentsReprod Toxicol Rev121998499500
- A.ScibiorH.ZaporowskaA.BanachCombined effect of vanadium (V) and Chromium (III) on lipid peroxidation in liver and kidney of ratsChem Biol Interact1592006213222
- J.O.OlopadeJ.R.ConnorVanadium and neurotoxicity: a reviewCurr Top Toxicol720113339
- A.ShaverJ.B.NgD.A.HallB.I.PosnerThe chemistry of peroxovanadium compounds relevant to insulin mimesisMol Cell Biochem1531995515
- A.MorinvilleD.MaysingerA.ShaverFrom Vanadis to Atropos: vanadium compounds as pharmacological tools in cell death signallingTrends Pharmacol Sci191998452460
- S.ShrivastavaA.JadoS.ShuklaEffect of Tiron and its combination with nutritional supplements against Vanadium intoxication in female albino ratsJ Toxicol Sci322007185192
- S.S.SoaresF.HenaoM.AurelianoC.Gutiérrez-MerinoVanadate induces necrotic cell death in neonatal rat cardiomyocytes through mitochondrial membrane depolarizationChem Res Toxicol212005607618
- M.YounesO.StrubeltVanadium-induced toxicity towards isolated perfused rat liver: the role of lipid peroxidationToxicology6619916374
- M.YounesE.KayserO.StrubeltEffect of antioxidant on vanadate-induced toxicity towards isolated perfused rat liversToxicology701991141149
- M.ElfantC.L.KeenSodium vanadate toxicity in adult and developing rats. Role of peroxidative damageBiol Trace Elem Res141987193208
- H.ZaporowskaEffects of vanadium on L-ascorbic acid concentration in rat tissuesGen Pharmacol251994467470
- A.ŚcibiorD.GołębiowskaA.AdamczykJ.KurusM.StaniszewskaI.SadokEvaluation of lipid peroxidation and antioxidant defense mechanisms in the bone of rats in conditions of separate and combined administration of vanadium (V) and magnesium (Mg)Chem Biol Interact2842018112125
- C.SchoneichU.DillingerF.von BruchhausenK.D.AsmusOxidation of polyunsaturated fatty acids and lipids through thiyl and sulfonyl radicals: reaction kinetics, and influence of oxygen and structure of thiyl radicalsArch Biochem Biophys2921992456467
- X.ShiX.SunN.S.DalalReaction of vanadium (V) with thiol generates vanadium (IV) and thiyl radicalsFEBS2711990185188
- S.S.LeonardG.K.HarrisX.ShiMetal-induced oxidative stress and signal transductionFree Radic Boil Med37200419211942
- M.ValkoH.MorrisM.T.CroninMetals, toxicity and oxidative stressCurr Med Chem12200511611208
- I.L.UsendeB.O.EmikpeJ.O.OlopadeHeavy metal pollutants in selected organs of African giant rats from three agro-ecological zones of Nigeria: evidence for their role as an environmental specimen bankESPR2420172257022578
- C.S.IbeB.I.OnyeansusiJ.O.HamboluFunctional morphology of the brain of the African giant pouched rat (Cricetomys gambianus; Waterhouse, 1840)Onderstepoort J Vet Res81201417
- Mott M. Bees. Giant African rats used to sniff landmines. <http://news.nationalgeographic.com>; 2004 [accessed 13.02.15].
- Maggie L. Giant rats to sniff out tuberculosis. <http://www.newscientist.com>; 2003 [accessed 16.02.15].
- PHS (Public Health Service)Public health service policy on humane are and the use of laboratory animals2015US Department of Health and Humane ServicesWashington (DC) pp. 99–158
- B.TodorichJ.O.OlopadeN.SurguladzeX.ZhangE.NeelyJ.ConnorThe mechanism of vanadium-mediated developmental hypomyelination is related to destruction of oligodendrocyte progenitors through a relationship with ferritin and ironNeurotox Res192011361373
- A.A.OyagbemiT.O.OmobowaleE.R.AsenugaA.O.AdejumobiT.O.AjibadeT.M.IgeSodium fluoride induces hypertension and cardiac complications through generation of reactive oxygen species and activation of nuclear factor Kappa BetaEnviron Toxicol32201610891101
- A.G.GornalJ.C.BardawillM.M.DavidDetermination of serum proteins by means of Biuret reactionJ Biol Chem1771949751766
- S.B.OlaleyeO.A.AdaramoyeP.P.ErigbaliO.S.AdeniyiLead exposure increases oxidative stress in the gastric mucosa of HCl/ethanol-exposed ratsWorld J Gastroenterol13200751215126
- S.P.WolffFerrous ion oxidation in the presence of ferric ion indicator xylenol orange for measurement of hydroperoxidesMethods Enzymol2331994182189
- E.O.FarombiJ.G.TahntengA.O.AgboolaJ.O.NwankoG.O.EmeroleChemoprevention of 2 acetylaminofluorene-induced hepatotoxicity and lipid peroxidation in rats by kolaviron a- Garcina kola seed extractFood Chem Toxicol382000353541
- D.J.JollowJ.R.MitchellN.ZampaglioneJ.R.GilletteBromobenzene induced liver necrosis: protective role of glutathione and evidence for 3, 4 bromobenzene oxide as the hepatotoxic metabolitePharmacol111974151169
- H.P.MisraI.FridovichThe role of superoxide anion in the autooxidation of epinephrine and a simple assay for superoxide dismutaseJ Biol Chem247197231703175
- J.T.RotruckA.L.PopeH.E.GantherA.B.SwansonD.G.HafemanW.G.HoekstraSelenium: biochemical role as a component of glutathione peroxidaseScience1791973588590
- W.H.HabigM.J.PabstW.B.JacobyGlutathione-S-transferase activity: the enzymic step in mercapturic acid formationJ Biol Chem2491974130139
- T.AkaikeM.AndoT.OdaT.DoiS.IjiriS.ArakiDependence on O2- generation by xanthine oxidase of pathogenesis of influenza virus infection in miceJ Clin Invest851990739745
- Y.XiaJ.L.ZweierMeasurement of myeloperoxidase in leukocyte-containing tissuesAnal Biochem24519979396
- G.L.EllmanTissue sulfhydryl groupsArch Biochem Biophys8219597077
- R.KayaliU.CakatayT.AkcayT.AltugEffect of alpha-lipoic acid supplementation on markers of protein oxidation in post-mitotic tissues of ageing ratCell Biochem Funct2420067985
- A.Z.ReznickL.PackerOxidative damage to proteins: spectrophotometric method for carbonyl assayMethods Enzymol2331994357363
- A.S.AkinleyeO.EbunoluwaA.A.OyagbemiO.T.OmobowaleGastrointestinal protective efficacy of Kolaviron (a bi-flavonoid from Garcinia kola) following a single administration of sodium arsenite in rats: biochemical and histopathological studiesPharmacognosy Res72015268276
- A.A.OyagbemiT.O.OmobowaleA.S.AkinrindeA.B.SabaB.S.OgunpoluO.DaramolaLack of reversal of oxidative damage in Renal tissues of lead acetate treated ratsEnviron Toxicol30201512351243
- S.ZhouZ.WenA.LiangS.ZhangExperimental research on therapeutic efficacy of tradition nal Chinese medicine Shengjing capsule extracts in treating spermatogenesis impairment induced by oxidative stressMed Sci Monit2220165056
- T.O.OmobowaleA.A.OyagbemiA.S.AkinrindeA.B.SabaO.T.DaramolaB.S.OgunpoluFailure of recovery from lead induced hepatoxicity and disruption of erythrocyte antioxidant defence system in Wistar ratsEnviron Toxicol Pharmacol37201412021211
- P.K.SharmaR.BhardwajB.S.DwarakanathR.VarshneyMetabolic oxidative stress induced by a combination of 2-DG and 6-AN enhances radiation damage selectively in malignant cells via non-coordinated expression of antioxidant enzymesCancer Lett2952010154166
- M.A.Abdel-WahhabS.H.Abdel-AzimA.A.El-NekeetyInulacrithmoides extract protects against ochratoxin A-induced oxidative stress, clastogenic and mutagenic alterations in male ratsToxicon522008566573
- H.ZaporowskaA.ScibiorLipid peroxidation in liver and kidneys of rats intoxicated with vanadiumActa Polon Toxicol519973540
- J.WuM.A.SalehA.KiraboH.A.ItaniK.R.MontanielL.XiaoImmune activation caused by vascular oxidation promotes fibrosis and hypertensionJ Clin Invest12620165067
- T.O.OmobowaleA.A.OyagbemiO.A.OyewunmiO.A.AdejumobiChemopreventive effect of methanolic extract of Azadirachta indica on experimental Trypanosoma brucei induced oxidative stress in dogsPharmacognosy Res72015249259
- L.YaidikarS.ThakurArjunolic acid, a pentacyclic triterpenoidal saponin of Terminalia arjuna bark protects neurons from oxidative stress associated damage in focal cerebral ischemia and reperfusionPharmacol Rep672015890895
- J.ZhouG.XuZ.BaiK.LiJ.YanF.LiSelenite exacerbates hepatic insulin resistance in mouse model of type 2 diabetes through oxidative stress- mediated JNK pathwayToxicol Appl Pharmacol2892015409418
- A.CumaogluC.CevikL.RackovaN.AriC.KarasuEffects of antioxidant stobadine on protein carbonylation, advanced oxidation protein products and reductive capacity of liver in streptozotocin-diabetic rats: role of oxidative/nitrosative stressBiofact302007171178
- C.IacobiniS.MeniniC.RicciA.ScipioniV.SansoniG.MazzitelliAdvanced lipid oxidation end-products mediate lipid- induced glomerular injury: role of receptor-mediated mechanismsJ Pathol2182009360369
- E.BiberogluK.BiberogluA.KirbasK.DaglarM.GencA.AvciCirculating and myometrial markers of oxidative stress in pregnant women with fetal growth restrictionJ Obstet Gynaecol Res4220162935
- Z.MengY.YanZ.TangC.GuoN.LiW.HuangAnti-hyperuricemic and nephroprotective effects of rhein in hyperuricemic micePlanta812015279285
- N.OhashiS.IshigakiS.IsobeN.TsujiT.IwakuraM.OnoHyperuricaemia is associated with renal damage independently of hypertension and intrarenal renin-angiotensin system activation, as well as their circadian rhythmsNephrol (Carlton)202015814819
- K.H.ZangZ.RaoG.Q.ZhangH.Y.QinAnticolitis activity of chinese herbal formula Yupingfeng powder via regulating colonic enterochromaffin cells and serotoninIndian J Pharmacol472015632637
- D.J.KennedyK.ShresthaB.SheeheyX.S.LiA.GuggilamY.WuElevated plasma marinobufagenin, an endogenous cardiotonic steroid, is associated with right ventricular dysfunction and nitrative stress in heart failureCirc Heart Fail8201510681076
- K.ChalupskyD.KracunI.KanchevK.BertramA.GorlachFolic acid promotes recycling of tetrahydrobiopterin and protects against hypoxia-induced pulmonary hypertension by recoupling endothelial nitric oxide synthaseAntioxid Redox Signal23201510761091