Abstract
Tungsten oxide (WO3)-containing glasses of WO3–ZnO–B2O3 were prepared using a conventional melt quenching method, and α-ZnWO4 nanocrystals were synthesized through the crystallization of glasses. A glass with the composition of 20WO3–50ZnO–30B2O3 showed the bulk crystallization of α-ZnWO4 nanocrystals with a diameter of ∼10 nm. Broad and asymmetric emission peaks were observed at the wavelength of λ ∼ 475 nm, i.e., blue emissions, in the photoluminescence spectra for the samples with α-ZnWO4 nanocrystals. From the degradation of the intensity of optical absorption under ultraviolet light (λ = 254 nm) irradiations for the solution consisting of crystallized particles with α-ZnWO4 nanocrystals and methylene blue, it was clarified that α-ZnWO4 nanocrystals formed have photocatalytic activities. The formation of α-ZnWO4 nanocrystals is discussed from the viewpoint of the glass-forming tendency.
1 Introduction
Tungstate compounds have received much attention because of their unique functions, e.g., ZnWO4 for scintillator [Citation1] and photocatalyst [Citation2], Sc2(WO4)3 showing negative thermal expansion [Citation3], Eu3+-doped (La,Gd)2(WO4)3 for phosphors [Citation4], and KYb(WO4)2 for solid state laser host [Citation5]. It is of importance to control the morphology, size, and quality of tungstate crystals for practical applications. On the other hand, glasses containing tungsten oxide (WO3) such as WO3–TeO2 and K2O–WO3–TeO2 have been synthesized, and their structure and properties have also received much attention [Citation6–Citation13]. For instance, the electronic polarizability of oxide ions (i.e., optical basicity) in WO3 is very high [Citation14,Citation15], and thus, WO3-based glasses show large third-order optical nonlinearities [Citation10,Citation16].
It is known that the crystallization of glass is an effective method for the fabrication of transparent materials with desired shapes and also nanostructures [Citation17–Citation19], and the crystallization technique has been applied to WO3-based glasses in order to fabricate glass-ceramics (crystallized glasses) consisting of functional WO3-based compounds [Citation20–Citation23]. For instance, Maczka et al. [Citation20] reported that a glass in the Cs2O–MgO–WO3–P2O5 system exhibits a prominent nanocrystallization during thermal treatments, providing Cs(Mg0.25W1.75)O6 nanocrystals with the size of 4–10 nm. Aleksandrov et al. [Citation21] synthesized glass–crystal composite materials with the LaBWO6 crystalline phase through the crystallization of 25La2O3–50WO3–25B2O3 glass. It is of interest to synthesize nano-scaled tungstate compounds through the crystallization of glasses and to clarify physical and chemical properties for practical device applications.
In this study, we focus our attention on the synthesis and optical properties of ZnWO4 nanocrystals through the crystallization of glasses. Unfortunately, for ZnWO4, it is impossible to prepare bulky glasses (not very thin or small flakes) with the same composition as this crystal, i.e., a glass of 50ZnO–50WO3. In order to design the crystallization of ZnWO4, therefore, glass-forming oxides such as SiO2 and B2O3 must be added. In the present study, we use the ternary system of WO3–ZnO–B2O3, although its glass-forming region is narrow as reported by Imaoka [Citation24]. ZnWO4 crystals have been synthesized by using various techniques such as solid state reaction and mechanochemical methods [Citation1,Citation2,Citation25–Citation27]. However, there has been no report on the crystallization of ZnWO4 in glasses. Some electrical, thermal, and optical properties of WO3–ZnO–B2O3 glasses have been reported so far [Citation6,Citation9], but any crystallization behavior has not been reported. Recently, Aleksandrov et al. [Citation28] examined the structure and crystallization behavior of MoO3–ZnO–B2O3 glasses and found that some glasses show the bulk crystallization of α-ZnMoO4 nanocrystals with a diameter of ∼5 nm and Eu3+-doped α-ZnMoO4 nanocrystals show enhanced photoluminescence emissions. Considering the chemical similarity of tungsten (W) and molybdenum (Mo) elements, the crystallization of ZnWO4 nanocrystals would be expected in WO3–ZnO–B2O3 glasses. As one of the optical properties of ZnWO4 nanocrystals synthesized in this study, photocatalytic activities for the decomposition of methylene blue under ultraviolet (UV) light were examined. There have been some reports on the photocatalytic activities of ZnWO4 powders [Citation2,Citation29–Citation31]. For instance, Huang and Zhu [Citation2] reported that ZnWO4 powders prepared by calcining co-precipitated precursors exhibit excellent photocatalytic activities. Garadkar et al. [Citation29] synthesized ZnWO4 nanoparticles (∼10 nm) by using a simple microwave assisted technique and found that they exhibit highly enhanced photocatalytic activity toward rhodamine B and methylene blue samples.
2 Experimental
Compositions (mol%) examined in this study are 10WO3–50ZnO–40B2O3, 20WO3–50ZnO–30B2O3, 30WO3–50ZnO–20B2O3, 30WO3–40ZnO–30B2O3, and 30WO3–30ZnO–40B2O3. Commercial powders of reagent grade WO3, ZnO, and B2O3 were used as starting materials and were melted in a platinum crucible at 1250 °C for 20 min in an electric furnace. The melts were poured onto an iron plate and pressed to a thickness of ∼1.5 mm by another iron plate. The glass transition, Tg, and crystallization peak, Tp, temperatures were determined using differential thermal analysis (DTA) (Rigaku, TG8120) at a heating rate of 10 K/min. Glass samples were heat-treated at different temperatures in an electric furnace in air, and the crystalline phase present in the crystallized samples was identified by X-ray diffraction (XRD) analysis (CuKα radiation). Morphologies of crystals in the crystallized samples were examined from high resolution transmission electron microscope (HRTEM, JEM-2100F) observations.
Photoluminescence (PL) emission and excitation (PLE) spectra were measured at room temperature with a spectrofluorophotometer (Shimadzu RF-5300PC). Photocatalytic activities of heat-treated samples were evaluated by the degradation of methylene blue (MB) under UV light (wavelength: λ = 254 nm). First, heat-treated samples were pulverized by using an agate mortar, and powders (100 mg) were mixed with a methylene blue solution (concentration: 5 ppm, volume: 100 mL) in a beaker. UV light (UV lamp; Toshiba, GL15-F) was irradiated onto the mixtures, and optical absorption spectra of solutions were measured by using a spectrophotometer (Shimadzu UV-3150). The total irradiation time of UV light was 180 min, and solutions were stirred by using a magnetic stirrer during UV light irradiations.
3 Results and discussion
3.1 Formation of α-ZnWO4 nanocrystals
The melt-quenched samples with the compositions of 30WO3–50ZnO–20B2O3, 30WO3–40ZnO–30B2O3, and 30WO3–30ZnO–40B2O3 include some amounts of crystals. On the other hand, the melt-quenched samples of 10WO3–50ZnO–40B2O3 and 20WO3–50ZnO–30B2O3 have a good optical transparency. In the XRD patterns for these two samples, only broad halo peaks being typical for glassy materials were observed. These results about the glass-forming tendency in the ternary WO3–ZnO–B2O3 system is well consistent with that reported by Imaoka [Citation24]. It is, therefore, confirmed from the present study that the glass-forming tendency in the samples with WO3 contents of more than 30 mol% is weak. Considering the purpose of this study, it is favorable to use glasses with WO3 and ZnO contents as large as possible. We, therefore, determined to use 20WO3–50ZnO–30B2O3 glass (designated here as 20WZB glass) and to examine its crystallization behavior.
shows the DTA pattern for the bulk 20WZB glass. An endothermic dip corresponding to the glass transition and three exothermic peaks corresponding to the crystallization are observed clearly, providing the values of Tg = 490 °C, Tp1 = 667 °C, Tp2 = 748 °C, and Tp3 = 811 °C. The optical photograph for the 20WZB glass is included in , indicating colorless in the visible light region. This suggests that the valence of W ions in the 20WZB glass is mainly W6+. Even if W5+ ions providing a color of brown are present in the 20WZB glass, their amount would be very small. From the optical absorption spectrum, it was estimated that the 20WZB glass has an optical absorption edge of the wavelength of λ = 354 nm (i.e., 3.50 eV).
Fig. 1 DTA curve of bulk 20WO3–50ZnO–30B2O3 glass. Heating rate was 10 K/min. Tg and Tp are the glass transition and crystallization peak temperatures, respectively. The optical photograph for the bulk glass is also included.
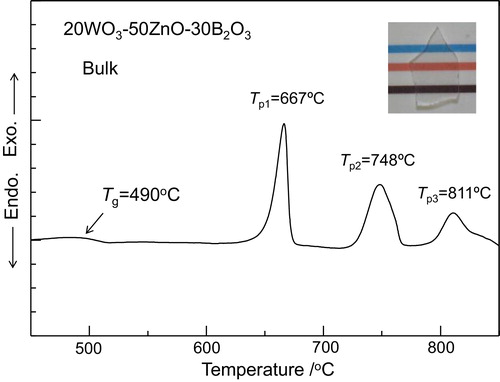
shows the XRD patterns for the samples obtained by different heat treatment temperatures in the 20WZB glass. The XRD peaks observed for the sample heat-treated at 680 °C for 3 h are assigned to α-ZnWO4 crystals (ICDD: No. 01-075-8594). For the samples heat-treated at 760 and 815 °C, the formation of Zn4O(BO2)6 crystals is observed besides α-ZnWO4. The results shown in indicate that the initial crystalline phase appearing in the crystallization of 20WZB glass is α-ZnWO4. In order to examine the crystallization behavior of α-ZnWO4 crystals, the 20WZB glass was heat-treated at temperatures lower than the first crystallization peak temperature of Tp1 = 667 °C, and the XRD patterns for the samples obtained are shown in . It is seen that the samples heat-treated at 570 and 610 °C for 3 h show XRD peaks assigned to α-ZnWO4 crystals. It should be pointed out that XRD peaks are very broad, suggesting that the size of α-ZnWO4 crystals might be small. The average particle size of α-ZnWO4 crystals formed in the heat-treated (610 °C, 3 h) sample was estimated from the peak width of XRD patterns using the Scherrer's equation, in which the (1 1 1) plane was used, and the value of ∼13 nm was obtained. A TEM photograph for the heat-treated (610 °C, 3 h) sample is shown in . The sample for TEM observations was prepared by grinding plate-shaped crystallized glass, and the edge of grain particles was observed. It is seen that crystals having a diameter of around 10 nm are present, being consistent with the average size (∼13 nm) of α-ZnWO4 crystals estimated from the Scherrer's equation. The XRD and TEM results, therefore, demonstrate that α-ZnWO4 nanocrystals are formed initially in the crystallization of 20WZB glass. The optical absorption spectrum for the transparent heat-treated (570 °C, 3 h) sample with α-ZnWO4 nanocrystals was measured, and an optical absorption edge of λ = 350 nm (i.e., 3.54 eV) was obtained. This value is almost the same as that (λ = 354 nm) for the base 20WZB glass. Garadkar et al. [Citation29] reported the optical band gap (Eg) of Eg = 3.4 eV for ZnWO4 nanoparticles (∼10 nm). Huang and Zhu [Citation2] reported the value of Eg = 3.02 eV for ZnWO4 powders with a diameter of 200–500 nm. The sample obtained in this study is composed of the glassy phase and α-ZnWO4 nanocrystals, and further studies would be necessary to determine the optical band gap of α-ZnWO4 nanocrystals themselves. At this moment, the amount of α-ZnWO4 nanocrystals has not been determined. However, considering the chemical composition of 20WO3–50ZnO–30B2O3 glass, after the formation of α-ZnWO4 nanocrystals with the ratio of ZnO/WO3 = 1, the chemical composition of the residual glassy phase would be close to 30ZnO–30B2O3 with the ratio of ZnO/B2O3 = 1. This would be a reason for the formation of Zn4O(BO2)6 crystals with the ratio of ZnO/B2O3 = 0.67 as the second crystalline phase.
Fig. 2 XRD powder patterns for the samples heat-treated at 680, 760, and 815 °C for 3 h in 20WO3–50ZnO–30B2O3 glass.
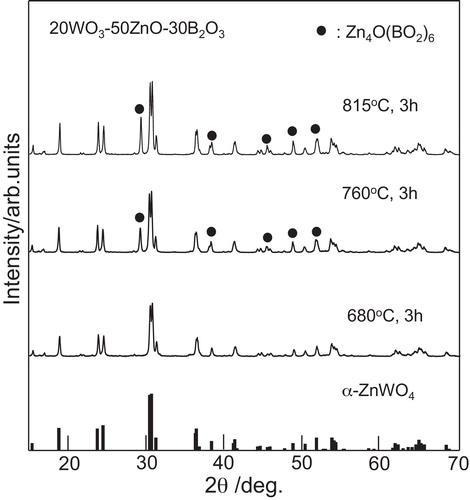
Fig. 3 XRD powder patterns for the samples heat-treated at 530, 570, and 610 °C for 3 h in 20WO3–50ZnO–30B2O3 glass. The optical photographs for the heat-treated samples are also included.
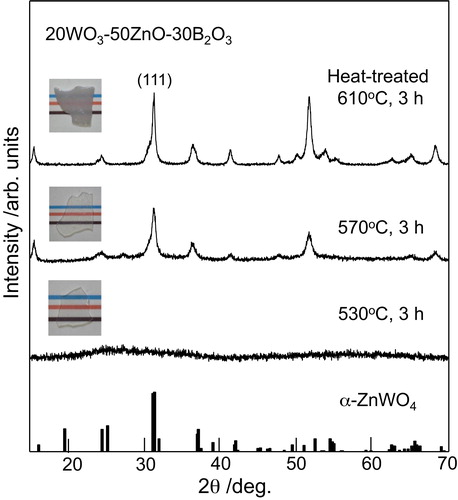
The glass of 20WO3–50ZnO–30B2O3 includes a relatively small amount (i.e., 30 mol%) of B2O3 which is a glass former. The stability of borate structures depends on the delocalization of formal charges of BnOm anions as a result of their polymerization [Citation32]. Considering the amount of B2O3 content, it is expected that the degree of the polymerization of B–O–B bonds would be small (i.e., short). Furthermore, it is recognized that the polymerized borates are often less stable for high-valence and small-sized cations [Citation32,Citation33]. Cations of higher valence such as WO3, MoO3, and Nb2O5 can form rather rigid coordination polyhedra with oxygen atoms. In the binary systems such as WO3–B2O3, MoO3–B2O3, and Nb2O5–B2O3, it is expected that the glass formation would be extremely difficult. Indeed, any wide glass-forming region has not been reported in these binary borate systems [Citation28]. On the other hand, the glass formation has been confirmed in the binary system of ZnO–B2O3 [Citation28], i.e., in the compositions of (43.9–63.6)ZnO–(56.1–36.4)B2O3 [Citation28], consequently providing the glass formation in the ternary system of WO3–ZnO–B2O3. This information on the glass-forming tendency suggests that it might be difficult to create rigid network structure with strong chemical bonds such as W–O–B in the ternary WO3–ZnO–B2O3 system. Furthermore, it is considered that the formation of compounds containing both WO3 and ZnO such as α-ZnWO4, but containing no boron atoms, would be enhanced in WO3–ZnO–B2O3 glasses. The above structural concept in glasses is an important clue for the design and control of crystallization in borate glasses. Indeed, various functional nanocrystals have been formed easily through the crystallization of borate glasses, e.g., α-ZnMoO4 nanocrystals in MoO3–ZnO–B2O3 glasses [Citation28], ferroelectric SrxBa1−xNb2O6 nanocrystals in SrO–BaO–Nb2O5–B2O3 glasses [Citation34], ferroelectric Ba1−xRE2x/3Nb2O6 nanocrystals (RE: Sm, Eu, Gd, Dy, Er) in RE2O3–BaO–Nb2O5–B2O3 glasses [Citation17], and CaF2 nanocrystals in CaF2–NaF–CaO–Al2O3–B2O3–SiO2 glasses [Citation35].
3.2 Photoluminescence of α-ZnWO4 nanocrystals
The excitation spectrum of the heat-treated (664 °C, 3 h) sample containing α-ZnWO4 nanocrystals is shown in (a), in which the emission of λ = 482 nm was monitored. A broad excitation emission peaked at λ ∼ 270 nm is observed. A PL spectrum at the excitation of λ = 267 nm for the sample is shown in (b). A broad and asymmetric emission peaked at λ ∼ 475 nm is observed, providing a blue color ((c)). A blue emission in α-ZnWO4 has been considered to be related to WO6 polyhedra [Citation36–Citation38]. A molecular orbital model for the octahedral (WO6)6− oxyanion complex (Oh point symmetry) shows that the highest occupied state with a t1g symmetry is associated with the 2p orbital of oxygen and the excited state consists of an electron at the W 5d orbital with a t2g symmetry [Citation38]. That is, the excitation and emission spectra in α-ZnWO4 are related to an electronic transition between the O(2p) and W(5d) states in the WO6 group. Zinc tungstate α-ZnWO4 has the wolframite crystal structure, in which the WO6 octahedral complex has an asymmetric shape with short, medium, and long W–O bond distances [Citation38]. The deformation of WO6 octahedra in α-ZnWO4 would be related to the appearance of the broad and asymmetric emission and PL spectra shown in . It is, therefore, concluded that α-ZnWO4 nanocrystals formed through the crystallization of 20WO3–50ZnO–30B2O3 glass have the electronic state being similar to α-ZnWO4 crystals synthesized by other preparation techniques.
Fig. 5 Excitation spectrum (a) monitored by the emission of λ = 482 nm and photoluminescence spectrum (b) at the excitation of λ = 267 nm for the heat-treated (664 °C, 3 h) sample containing α-ZnWO4 nanocrystals in 20WO3–50ZnO–30B2O3 glass. The optical photograph (c) for the heat-treated sample with a blue photoluminescence is also included.
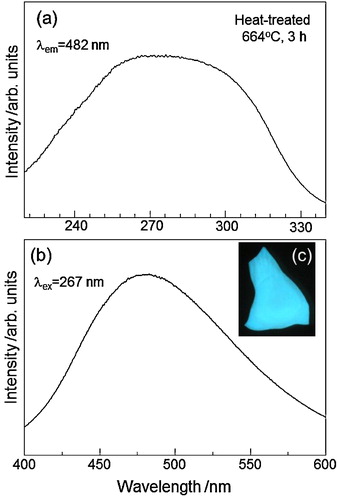
3.3 Photocatalytic properties of α-ZnWO4 nanocrystals
For the photocatalytic activity of α-ZnWO4 nanocrystals, the crystallized samples obtained by a heat treatment at 610 °C for 3 h were used. The samples were pulverized by a agate mortar, and the SEM photograph for the particles obtained is shown in (a), indicating the particle size of 1–10 μm and sharp surfaces. UV light (λ = 254 nm) was irradiated onto the solution consisting of these particles and methylene blue. However, any degradation of methylene blue, i.e., the decrease in the intensity of optical absorptions, was not observed. That is, any photocatalytic activity was not confirmed in the particles of the crystallized sample with α-ZnWO4 nanocrystals. In the crystallized samples themselves, it is considered that the area of α-ZnWO4 nanocrystals exposing at the surface would be small, because many α-ZnWO4 nanocrystals are surrounded by the residual glassy phase and also are present in the interior of the particles.
Fig. 6 SEM photographs for the pulverized particles (a) for the heat-treated (610 °C, 3 h) sample and for particles (b) obtained by etching in 1 N HNO3 solution for the heat-treated sample in 20WO3–50ZnO–30B2O3 glass.
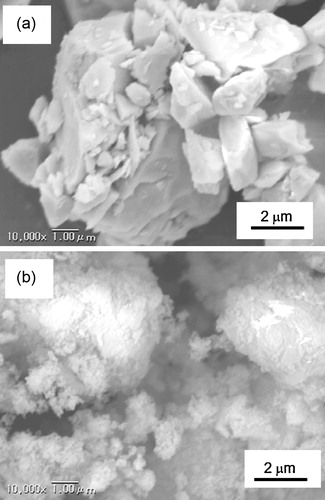
In order to increase the exposure area of α-ZnWO4 nanocrystals at the surface (i.e., the elimination of the glassy phase), the pulverized particles were etched by using a 1 N HNO3 solution, and the SEM photograph for the particles obtained is shown in (b). It is seen that the surface state of the etched particles ((b)) changes largely in comparison with that of non-etched particles ((a)). The appearance of the rough surface shown in (b) indicates that the glass of 20WO3–50ZnO–30B2O3 is etched effectively by a 1 N HNO3 solution. UV light (λ = 254 nm) was irradiated onto the solution of these etched particles and methylene blue, and the results on the optical absorption spectra in the visible light region at room temperature are shown in . It is seen that the intensity of optical absorption due to the methylene blue decreases with increasing UV light irradiation time. In particular, for the irradiation time of 180 min, it is considered that the methylene blue present in the solution is almost decomposed. The results shown in , therefore, indicate that α-ZnWO4 nanocrystals formed through the crystallization of 20WO3–50ZnO–30B2O3 glass have photocatalytic activities. Similar experiments were carried out for TiO2 particles (Nippon Aerosil Co. P25, particle size: ∼21 nm) in order to confirm the validity of our experiments applied in this study, and it was found that the methylene blue present in the solution was almost decomposed in the UV (λ = 254 nm) irradiation time of 90 min. Garadkar et al. [Citation29] examined the photocatalytic activity of ZnWO4 nanoparticles (∼10 nm) synthesized by a simple microwave assisted technique for the degradation of methylene blue in a solution (100 mL, 1.0 × 10−5 mol/L) under UV light (λ = 254 nm) and found that the UV light irradiation time of 60 min is enough for the decomposition of methylene blue. The photocatalytic activity of α-ZnWO4 nanocrystals formed through the crystallization of glasses in this study is, therefore, not so high compared with the data reported so far [Citation29]. In this study, the glassy phase that remained in the crystallized glasses were etched by using a 1 N HNO3 solution, and α-ZnWO4 nanocrystals were taken. It is required to clarify the surface state of α-ZnWO4 nanocrystals taken by the etching process. Furthermore, it is of interest to study photocatalytic properties and behaviors including intermediate products for our samples in more detail. It is also strongly desired to pattern α-ZnWO4 nanocrystals more densely only at the surface of bulk WO3–ZnO–B2O3 glasses for practical applications, for instance, by using laser-induced crystallization techniques [Citation39].
4 Conclusions
The WO3-containing glasses based on the WO3–ZnO–B2O3 system were prepared using a conventional melt quenching method in order to design crystallized glasses with α-ZnWO4 nanocrystals. It was difficult to prepare glasses with a large amount (∼30 mol%) of WO3, but the melt-quenched sample with the composition of 20WO3–50ZnO–30B2O3 showed an optically transparent glass (20WZB glass). The 20WZB glass showed the bulk crystallization of α-ZnWO4 nanocrystals with a diameter of ∼10 nm. Broad and asymmetric emissions peaking at the wavelength of ∼475 nm, i.e., blue emissions, in the photoluminescence spectrum were observed in the samples with α-ZnWO4 nanocrystals. From the degradation of the absorption of methylene blue color under UV light irradiations, it was clarified that α-ZnWO4 nanocrystals formed have photocatalytic activities.
Acknowledgements
This study was supported by the Grant-in-Aid for Scientific Research from the Ministry of Education, Science, Sport, Culture, and Technology, Japan (No. 23246114), and by Program for High Reliable Materials Design and Manufacturing in Nagaoka University of Technology.
Notes
Peer review under responsibility of The Ceramic Society of Japan and the Korean Ceramic Society.
References
- P.F.SchofieldK.S.KnightG.GresseyJ. Mater. Sci.31199628732877
- G.HuangY.ZhuMater. Sci. Eng. B1392007201208
- J.S.O.EvansT.A.MaryA.W.SleightJ. Solid State Chem.1371998148160
- C.A.KodairaH.F.BritoM.C.F.C.FelintoJ. Solid State Chem.1712003401407
- P.KloppU.GriebnerV.PetrovX.MateosM.A.BursukovaM.C.PujolR.SoleJ.GavaldaM.AguiloF.GuellJ.MassonsT.KirikivF.DiazAppl. Phys. B742002185189
- F.P.KoffybergF.A.BenkoJ. Non-Cryst. Solids401980717
- J.MaaßH.AhrensP.FröbelK.BärnerE.R.GiessingerR.BraunsteinSolid State Commun.871993567572
- T.KosugeY.BeninoV.DimitrovR.SatoT.KomatsuJ. Non-Cryst. Solids2421998154164
- J.R.KimG.K.ChoiD.K.YimJ.S.ParkK.S.HongJ. Electroceram.1720066569
- G.PoirierM.NalinY.MessaddeqS.J.L.RibeiroSolid State Ionics1782007871875
- Y.ChenQ.NieT.XuS.DaiX.WangX.ShenJ. Non-Cryst. Solids354200834683472
- T.ScheikeH.SegawaS.InoueY.WadaOpt. Mater.34201214881492
- K.YoshimotoA.MasunoH.IonueY.WatanabeJ. Am. Ceram. Soc.95201235013504
- V.DimitrovT.KomatsuJ. Solid State Chem.1632002100112
- V.DimitrovT.KomatsuJ. Solid State Chem.1782005831846
- V.DimitrovT.KomatsuJ. Non-Cryst. Solids2491999160179
- H.IdaK.ShinozakiT.HonmaK.Oh-ishiT.KomatsuJ. Solid State Chem.1962012384390
- K.ShinozakiT.HonmaT.KomatsuJ. Appl. Phys.1122012 093506/1–093506/7
- T.KomatsuT.HonmaInt. J. Appl. Glass Sci.42013125135
- M.MaczkaL.KepinskiJ.HanuzaS.KojimaJ. Non-Cryst. Solids353200746814690
- L.AleksandrovT.KomatsuR.IordanovaY.DimitrievOpt. Mater.342011201206
- Y.WangT.HonmaT.KomatsuOpt. Mater.3520139981003
- Y.TakiK.ShinozakiT.HonmaT.KomatsuL.AleksandrovR.InordanovaJ. Non-Cryst. Solids38120131722
- M.ImaokaJ. Ceram. Soc. Jpn.691961282306
- G.B.KumarK.SivaiahS.BuddhuduCeram. Int.362010199202
- M.ManchevaR.IordanovaY.DimitrievJ. Alloys Compd.50920111520
- M.MaiC.FeldmannJ. Mater. Sci.47201214271435
- L.AleksandrovT.KomatsuR.IordanovaY.DimitrievOpt. Mater.332011839845
- K.M.GaradkarL.A.GhuleK.B.SapnarS.D.DholeMater. Res. Bull.48201311051109
- W.WangJ.ShenN.LiM.YeMater. Lett.1062013284286
- T.D.SavicI.L.ValidzicT.B.NovakovicZ.M.VukovicM.I.ComorJ. Cluster Sci.242013679688
- N.I.LeonyukJ. Cryst. Growth1741997301307
- H.FloodT.FörlandActa Chem. Scand.11947592604
- T.YamazawaT.HonmaH.SuematsuT.KomatsuJ. Am. Ceram. Soc.92200929242930
- K.ShinozakiA.NojiT.HonmaT.KomatsuJ. Fluorine Chem.14520138187
- M.BonanniL.SpanhelM.LerchE.FügleinG.MüllerChem. Mater.101998304310
- Z.LouJ.HaoM.CociveraJ. Lumin.992002349354
- V.B.MikhailikH.KrausG.MillerM.S.MykhaylykD.WahlJ. Appl. Phys.972005 083523/1–083523/8
- T.KomatsuT.HonmaJ. Asian Ceram. Soc.12013916