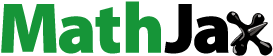
Abstract
We propose a novel electrode design for co-planar single chamber solid oxide fuel cells. The concentric electrodes as well as the controlled gas flow in perpendicular to the center of the electrode enable continuous laminar flow from the cathode to the anode, which leads optimal residence time of the gas near electrodes and in turn allows high-performance stable operation. The steady state gas flow without turbulence determines not only the local thermodynamic equilibrium, but also the kinetics of the electrochemical reactions. The cell with the concentric electrodes shows the maximum open circuit voltage (OCV) of 0.70 V, and the maximum power density is improved by 154%. In contrast, the conventional cell with linear-shaped electrodes exhibits the maximum OCV of 0.55 V. Impedance spectroscopy reveals that the polarization resistance is reduced by altering the electrode design from the linear-shape to the concentric design.
1 Introduction
Single chamber solid oxide fuel cells (SC-SOFCs) can propose an alternative way to overcome the hurdle associated with low ionic conductivity of solid electrolytes and to eliminate the required gas-tight sealing between two electrodes [Citation1–Citation3]. SC-SOFCs are operated with a mixed gas of fuel and oxygen, which allows for only one gas chamber and simplified system configurations. In particular, thin electrolyte films are unnecessary in co-planar type SC-SOFCs in which anode and cathode are located on the same side of the electrolyte substrate, so that narrowing the inter-electrode gap could shorten the conduction path of the oxygen ions instead of thinning down the electrolyte thickness [Citation4–Citation6]. Given these considerations, a mechanically stable power generation system could be implemented by using a thick electrolyte substrate [Citation7,Citation8]. Since the first demonstration of co-planar SC-SOFCs by Hibino et al., the interdigitated array of electrodes, in which comb-like electrodes are positioned at narrow inter-electrode distance to minimize the conduction path and to maximize the electrode area at the same time, has been developed to improve the cell performance [Citation4,Citation5]. Co-planar SC-SOFCs with the interdigitated design showed the performance of ∼100 mW cm−2 at 800 °C using methane and air mixture gas, while even the cell containing a single pair of electrodes exhibited the performance of 245 mW cm−2 at 600 °C under butane and air mixture gas [Citation8–Citation10].
Fig. 1 (a) Schematic diagrams of the measurement system for a single chamber SOFC. (b) Schematic diagram of the gas flow pattern for the concentric and linear electrode cells.
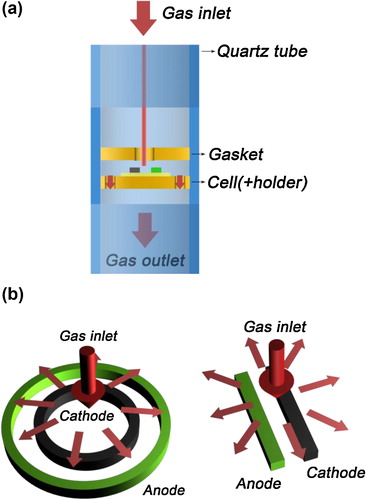
Fig. 2 (a) Optical photograph of the screen-printed cells. SEM image of the screen-printed cell: (b) anode and (c) cathode.
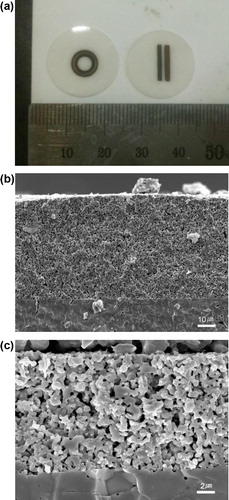
Fig. 5 Nyquist plot of the impedance spectra of the screen-printed cells in air and CH4–air mixture.
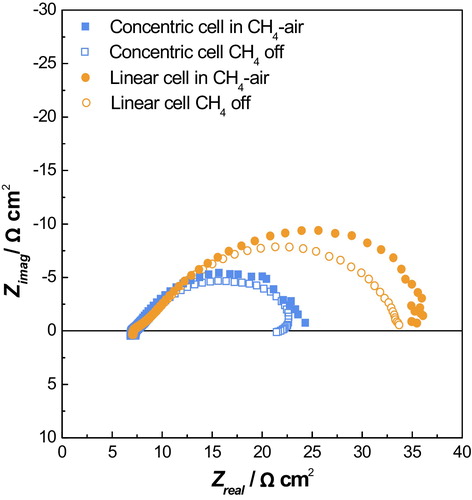
Despite the successful demonstrations of co-planar SC-SOFCs with various designs of electrodes or stacking of the unit cells, their fundamental operational principles such as reaction kinetics, local equilibrium of oxygen chemical potential, and reaction selectivity of the electrodes are still not clearly elucidated [Citation11–Citation18]. During the last decade, there have been significant efforts to understand the influence of flow geometry on the performance of co-planar SC-SOFCs with a single pair of linear-shaped electrodes. Jacques-Bedard et al. demonstrated that the inlet gas flow directed to the anode side decreased significantly the open circuit voltage (OCV) and power density of the cells, in which methane and oxygen were consumed rapidly due to the catalytic activity of nickel toward methane partial oxidation, resulting in deficient oxygen condition near cathode [Citation19]. Buergler et al. and Ahn et al. revealed the influence of electrode geometries on the cell performance by varying the electrode configurations [Citation14,Citation20]. They indicated that the turbulent flow may be induced between the electrodes so that the open circuit voltage (OCV) fluctuates. Jasinski also claimed that a laminar flow field is necessary for optimal operation of the co-planar SC-SOFCs and the inter-electrode gap under the 50 μm could cause the turbulent gas flow, leading to low OCV and degraded performance [Citation21]. Riess introduced the concept of residence time to explain the importance of steady state flow conditions near the electrodes. Only the short residence time around 10 ms could guarantee the selectivity of the cathode for high performance because the perovskite cathode materials retained the inherent catalytic activity toward the oxidation of hydrocarbon fuel [Citation22].
In this study, we propose novel concentric electrodes in which the circular shaped inner cathode is surrounded by a ring-shaped outer anode. When supplied gas flows perpendicular to the cell with the concentric electrode array: (1) reactive mixed gas of methane and oxygen could meet the overall active area of electrodes without partial exposure; (2) the gas flow direction could be manipulated from the one electrode to another so that the reaction kinetics of electrodes at all array positions are identical; (3) if the anode is placed near the gas outlet, the influence of methane oxidation induced volume expansion can be minimized. Consequently, the strategy of perpendicular and radial flow by concentric electrode array would reduce the prolonged residence time near electrode, which had been considered to give rise to the perturbed catalytic selectivity. We compare the concentric electrode type cell with the conventional linear-shaped electrodes in terms of I–V characteristics and electrochemical impedance spectroscopy.
2 Experimental procedure
Electrolyte pellets were fabricated via uni-axial pressing with YSZ powder (TZ-8Y, Tosoh Co.) and sintered at 1500 °C. After sintering, YSZ pellet exhibited the diameter of 20 mm and subsequently the sintered pellet was polished to eliminate the surface defects, resulting in the final thickness of 0.5 mm. The paste of NiO-YSZ anode was prepared with commercial NiO-YSZ composite powder (Fuel Cell Materials, NiO:YSZ = 60:40 wt%), ethyl cellulose (Sigma Aldrich Co.), and α-terpinol (Sigma Aldrich Co.). The screen-printed NiO-YSZ anode was sintered at 1250 °C, resulting in the electrode with the thickness of 60 μm. LSM-YSZ (La0.8Sr0.2MnO3, Rhodia Co., LSM:YSZ = 70:30 wt%) cathode was deposited in the same way, followed by sintering at 1000 °C, which resulted in 13 μm-thick cathode. The cells with both linear-shaped and concentric electrodes were fabricated at an inter-electrode distance of 1 mm. Microstructures of the sintered cell were observed by scanning electron microscopy (SEM, JEOL, JSM-7001F).
Au wire and paste (8884-G, ESL Co.) were used as a current collector. Au was selected because of the absence of catalytic activity toward partial oxidation of methane and oxygen reduction reaction. In contrast, platinum is undesirable since it is an excellent catalyst for the oxygen reduction reaction. Before the electrochemical measurement, the as-prepared cells were treated in 4% H2–Ar balanced gas to reduce the NiO anode into metallic nickel. All electrochemical characterizations were performed under the gas flow of CH4 80, O2 40 and N2 160 sccm (standard cubic centimeter per minute) at 800 °C. A k-type thermocouple was placed 5 mm above the cell to monitor the actual temperature near the cell. The actual reaction chamber was confined by the two gaskets which had the diameter of 45 mm; the upper gasket had a single inlet hole at the center, while the lower gasket retained a groove for positioning the cell and 8 holes with 2 mm diameter at the edge for emitting the outlet gas. The current interruption method was used to characterize the I–V characteristics of each cell with a potentiostat (1287A, Solartron). The electrochemical impedance spectroscopy was also measured to determine the polarization resistance of the cells using potentiostat and frequency analyser (1252, Solartron) in the frequency range of 100 kHz–0.1 Hz with AC amplitude of 20 mV.
3 Results and discussion
a schematically depicts the measurement system configuration for the SC-SOFCs in which the feed gas mixture is focused to the center of the cell in a perpendicular direction using gasket and guide tube. When the steady state condition is set in the tubular chamber, the supplied gas generates radial flow along the gasket. In this case, the inner cathode in the concentric electrode cell receives the incoming gas firstly and the outer anode faces the radially distributed gas afterward as shown in b. In contrast, the gas flow is focused to the center of the cell where both the linear-shaped cathode and the anode receive the radially spread gas mixture simultaneously. Total gas flow was set to 280 sccm (standard cubic centimeter per minute) and the actual area of the gas chamber was 15.9 cm2, which was confined by the gap of two gaskets. Under this circumstance, the velocity of gas flow was 0.29 cm s−1. At the anode side, the concentric electrode array will have the constant residence time of 83 ms, while that at the linear electrode will be in the range of 345–488 ms. The volume expansion of gas during the fuel cell reactions was neglected to estimate the residence time.
Electrochemical cells were fabricated to confirm the benefits of concentric electrodes via conventional screen-printing process. The screen-printed concentric and linear electrode cells were configured to have the same total electrode area and inter-electrode distance to eliminate other variables except for the electrode shape. shows the as-fabricated cells and their microstructures. The total electrode area was 10 mm2 and the average inter-electrode distance was 1 mm. In a single-chamber operation, the thickness of the nickel-based anode is a critical factor to determine the OCV value [Citation11,Citation19]. Nickel is vulnerable to oxidation under the fuel and air mixture, which can poison the catalytic ability. Thick electrode with at least over 50 μm is required to reduce the local oxygen partial pressure near electrolyte where the electrochemical oxidation of fuel occurs actively. Both the screen-printed concentric and linear electrode cells have sufficient thicknesses of 57 and 61 μm, respectively.
The effect of the flow structures was firstly observed by OCV measurements as shown in . Both concentric and linear cells exhibited the OCV oscillation as a function of time, which was induced by the cyclic oxidation and reduction of nickel in the presence of oxygen [Citation23,Citation24]. The concentric cell exhibited the higher average OCV of 0.67 V than 0.42 V for the linear cell. The amplitude of oscillation was 0.20 V in the concentric cell, while that of the linear cell was 0.45 V. More stable and higher OCV of the concentric cell represents higher oxygen partial pressure difference between electrodes. This observation implies that the concentric electrode enables the laminar flow from cathode to anode so that the desirable residence time for the catalytic selectivity would be set in the concentric cell.
presents the I–V characteristics of the concentric and linear cells. The concentric electrode cell exhibited 1.54 times higher power density and larger OCV than those in the linear electrode cell. The maximum power density and OCV for the concentric cell were 6.23 mW cm−2 and 0.71 V, while 4.03 mW cm−2 and 0.59 V for the linear cell. OCV was slightly lower than theoretical value due to non-ideal selectivity of the catalysts and small fluctuation of OCV. Ideal OCV can be achieved only if the selectivity of electrodes would be ideal on each electrode [Citation22]. As expected, the screen-printed cells show poor performance because the ohmic resistance was very large due to long conduction path between the electrodes, which was 1000 times longer than that of anode supported cells that involve 10 μm-thick electrolytes. If the inter-electrode distance narrows to 100 μm, the maximum power density could be increased to a few hundred milliwatts per square centimeter. The ohmic losses of the concentric and linear cells, which were represented by the slope of I–V curves, were almost similar. This similarity confirmed the identical length of ionic conduction path which was determined by the inter-electrode distance.
To elucidate the influence of electrode design on the electrode reaction kinetics, electrochemical impedance spectroscopy (EIS) was conducted as shown in . EIS spectra were measured under the mixture of CH4 and O2 and subsequently impedance responses were observed immediately at the temporal pause of CH4 supply. During the operation of SC-SOFCs, H2 and CO can be generated via partial oxidation of CH4 at anode surfaces as given in the following equation.(1)
(1) Subsequently, thus generated H2 and CO are oxidized electrochemically leading to faradaic currents throughout the cell. Simultaneously, electrochemical oxygen reduction reactions take place at cathode surfaces replenishing oxygen ions. When CH4 and O2 are supplied to the cell under OCV conditions, chemical reactions, such as partial oxidation of CH4 and dissociative adsorption of gas molecules would take place dominantly, which could induce the noise of the impedance response. Impedance responses after interruption of CH4 flow, no chemical reactions can occur so that electrochemical reduction of oxygen into oxygen ions which, in turn, will oxidize the adsorbed species on the anode surfaces. In this regard, impedance responses owing to the faradaic currents can be separated by elimination of chemical reforming reactions in the absence of CH4 supply as shown in .
High frequency intercepts of impedance spectra among all measured conditions exhibited the similar value of 7.2 Ω cm2, which confirmed the similar ohmic resistances between the concentric and linear cell. On the other hand, the concentric cell exhibited the much lower polarization resistance of 17.44 Ω cm2 under CH4 and O2 mixture and 15.20 Ω cm2 after the interruption of CH4. The linear cell showed the polarization resistance of 29.01 Ω cm2 under CH4 and O2, whereas 26.49 Ω cm2 when no supply of CH4. As expected, the interruption of CH4 flow stabilized the spectra and lowered the polarization resistance due to the elimination of perturbation by chemical reactions. Much lower polarization resistance of the concentric cell represented better electrochemical reaction kinetics than that of the linear cell. The concentric electrodes could allow for the radial flow from the center of the cell to the outside, which induced the laminar gas flow from cathode to anode. This laminar flow could prevent the turbulence of inlet and outlet gas flow so that the reactions at the electrode surfaces were activated. On the other hand, the local inlet gas composition at the electrode surfaces could be randomized when the electrodes were arrayed linearly. Because the exothermic partial oxidation of CH4 accompanied the volume expansion, turbulent flow could be developed in the linear cell, which, in turn, hindered the electrochemical reactions.
Higher OCV of the concentric cell than that of the linear cell can be conceived with the gas flow characteristics confirmed by the EIS analyses. The kinetics and equilibrium state of SC-SOFCs could not be clarified because the electrodes are in the same gas condition with the SC-SOFCs configuration. Only the different catalytic selectivity of the electrodes toward partial oxidation of methane induces local gradient of oxygen partial pressure near electrodes. Therefore, the gas composition near electrodes under steady state condition determines not only kinetics of the electrochemical reactions at the electrodes but also the equilibrium state, which would optimize the residence time near electrodes. In other words, the optimized reaction kinetics are closely related to the local oxygen chemical potential so that the lower polarization resistance of the concentric cell gives rise to the higher OCV as well.
4 Conclusions
The effect of the concentric electrodes for high performance coplanar SC-SOFCs was investigated with respect to the conventional linear-shaped electrode cell. The concentric electrode design induced the radial gas flow from the cathode to the anode, so that laminar flow could be developed through the cell leading to the desired residence time of gaseous reactants. To confirm the idea of the concentric shape, both concentric- and linear-shaped electrode cells were characterized by the electrochemical measurement. The concentric cells exhibited higher OCV of 0.71 V and the maximum power density of 6.23 mW cm−2, while those of the linear cell were 0.59 V and 4.03 mW cm−2 at 800 °C. Lower polarization resistance associated with the concentric cell accounted for better kinetics of electrode reactions than those of the linear cell. Laminar flow brought by the concentric electrode design allowed for the activated electrode reactions, which induced higher difference in the oxygen chemical potentials between the electrodes, leading to higher OCV of the cell.
Acknowledgements
This work was supported by the National Research Foundation of Korea (NRF) grant funded by the Korea government (MEST) (No. 2012R1A3A2026417) and the Basic Research Laboratory (BRL) Program (No. 2011-8-2048). It was also partially supported the third Stage of Brain Korea 21 Plus Project.
Notes
Peer review under responsibility of The Ceramic Society of Japan and the Korean Ceramic Society.
References
- T.HibinoH.IwaharaChem. Lett.7199311311134
- T.HibinoA.HashimotoT.InoueJ.TokunoS.YoshidaM.SanoScience288200020312033
- M.KuhnT.W.NappornEnergies22009332337
- T.HibinoK.UshikiT.SatoY.KuwaharaSolid State Ionics81199513
- T.HibinoK.UshikiY.KuwaharaSolid State Ionics9119966974
- J.FleigH.L.TullerJ.MaierSolid State Ionics1742004261270
- T.HibinoH.TsunekawaS.TanimotoM.SanoJ. Electrochem. Soc.147200013381343
- T.HibinoA.HashimotoM.SuzukiM.YanoS.YoshidaM.SanoJ. Electrochem. Soc.1492002A195A200
- S.-J.AhnJ.-H.LeeJ.KimJ.MoonElectrochem. Solid State Lett.92006A228A231
- S.-J.AhnY.-B.KimJ.MoonJ.-H.LeeJ.KimJ. Electroceram.172006689693
- T.W.NappornX.Jacques-BedardF.MorinM.MeunierJ. Electrochem. Soc.1512004A2088A2094
- T.W.NappornF.MorinM.MeunierElectrochem. Solid State Lett.72004A60A62
- Y.-B.KimS.-J.AhnJ.MoonJ.KimH.-W.LeeJ. Electroceram.172006683687
- S.-J.AhnY.-B.KimJ.MoonJ.-H.LeeJ.KimJ. Power Sources1712007511516
- M.KuhnT.W.NappornM.MeunierS.VengallatoreD.TherriaultJ. Power Sources1942009941949
- M.KuhnT.W.NappornM.MeunierD.TherriaultSolid State Ionics1812010332337
- B.WeiZ.LüX.HuangM.LiuD.JiaW.SuElectrochem. Commun.112009347350
- Z.WangZ.LuB.WeiX.HuangK.ChenM.LiuW.PanW.SuElectrochem. Solid State Lett.132010B14B16
- X.Jacques-BedardT.W.NappornR.RobergeM.MeunierJ. Electrochem. Soc.1542007B305B309
- B.E.BuerglerM.OchsnerS.VuilleminL.J.GaucklerJ. Power Sources1712007310320
- P.JasinskiMicroelectron. Int.2520084248
- I.RiessJ. Power Sources1752008325337
- D.LeeD.KimJ.KimJ.MoonSci. Rep.420143937
- X.ZhangC.S.-M.LeeD.O.HaywardD.M.P.MingosCatal. Today1052005283294