Abstract
Bisphenol A (BPA) is of global concern because of its disruption of endocrine systems and ubiquity in aquatic environment. In this study, BPA antibody was successfully immobilised on novel mesoporous silica (MPS) carriers that display unique properties such as high surface area, highly uniform pore distribution and high adsorption capacity. Mobil Crystalline Material MCM-41 (2.7 nm), Santa Barbara Amorphous SBA-15-1 (12.3 nm) and SBA-15-2 (24.0 nm) materials were used as supports for these antibodies. On these carriers, the BPA antibody immobilisation reached 40 μg mg−1. For each MPS, 15 ng of BPA antigen was adsorbed on 1 mg of MPS–antibody composite, which resulted in an antibody activity of 30%. The highest recovery rate of BPA antigen was observed for 80% acetonitrile in 10 mM phosphate buffer (pH 7). After six repeated runs, BPA antibodies immobilised on SBA-15-1 and SBA-15-2 retained about 30% of their initial activity. In contrast, these antibodies showed 13% lower residual activity on MCM-41 than on SBA-15-1 and SBA-15-2. This result indicated that entire antibody molecules were adsorbed inside SBA-15-1 and SBA-15-2 pores, stabilising their structural conformation.
1 Introduction
Mesoporous silica (MPS) has attracted considerable research interest since Mobil first proposed its use in 1992 [Citation1]. In addition to being easily functionalisable, this material has exhibited stability for synthesis, high and specific surface areas and pore volumes [Citation2,Citation3], well-defined channels, hydrothermal stability [Citation4], adjustable pore sizes [Citation5] and narrow size distribution [Citation6]. Therefore, MPS can find applications in adsorption materials [Citation7–Citation9], catalyst carriers [Citation10–Citation12] and separation sensors [Citation13,Citation14]. In addition, its implementation in biological applications, such as drug delivery [Citation15], transfection and regenerative medicine [Citation16], is expected.
The immobilisation of enzymes, antibodies and other proteins on solid supports that can improve the biological stability and activity under extreme conditions has proven quite useful for catalysis, sensing and separation applications [Citation17–Citation19]. The creation of MPS-based high-performance biosensors and protein separation systems hinges on a better understanding of the factors that influence the pore size and morphology in these materials. Nakanishi et al. [Citation20] have previously described the relationship between protein A molecules and MPS pore sizes, as well as the reusability of antibodies immobilised on MPS. They observed that the MPS-immobilised protein A showed higher binding activity to the antigen (IgG) on MPSs with a smaller pore size (2.7 nm). Interestingly, 8-anilino-1-naphthalenesulphonic acid (ANS) analysis indicated that conformational changes during the immobilisation process did not impact IgG binding activity. These findings suggested that the optimisation of MPS pore size was extremely important for protein A immobilisation [Citation21–Citation23].
Often present in environmental water, bisphenol A [2,2-bis(4-hydroxyphenyl)propane, BPA] has now drawn attention because of its harmful effects on the endocrine systems of humans and wild animals [Citation24–Citation27]. Widely used for the production of epoxy resins and polycarbonate plastics [Citation28], this endocrine disrupter is currently found in baby bottles, plastic drinking bottles, dental seals, injection syringes, canned beverage drinks and metal food containers. Several methods based on high-performance liquid chromatography (HPLC) and gas chromatography/mass spectrometry (GC/MS) [Citation29–Citation31] have been developed for the characterisation of BPA. However, these methods are required to elaborate pretreatments [Citation32,Citation33]. Trace amounts of BPA have also shown estrogenic activity even at concentrations as low as 0.001 ng mL−1 [Citation34–Citation36]. In this ultra-low concentration range, many factors, such as contamination from glasswares, containers and injection syringes, interfere with BPA characterisation. Furthermore, purified water contains certain BPA levels as a result of leaching from resin parts in the water purification system. Therefore, effective methods to concentrate and to detect low BPA concentrations need to be developed. Because of their highly uniform channels, large surface area and narrow pore-size distribution, MPS supports are expected to be ideal sensing materials for enhancing the stability and sensitivity of BPA detection.
To the best of our knowledge, few comparative studies have addressed the adsorption of BPA antibody onto MPS, and its application to microanalysis. In this study, various MPSs were used as BPA antibody carriers and their activity was evaluated. Organic solvent-tolerance and recyclability of the MPS immobilised antibody were investigated because pore size plays a pivotal role in determining the immobilisation state of antibodies which is related to the stability of antibodies.
The enhanced antibody stability and activity upon immobilisation on optimal MPS carriers will be advantageous in a wide range of industrial scale applications such as microanalysis systems and biosensors.
2 Experimental
2.1 Materials
All the materials were of analytical grade and were used as received from the manufacturer. The triblock poly(ethylene oxide)–poly(propylene oxide)–poly(ethylene oxide) copolymer (Pluronic P123, Mw = 5800, EO20PO70EO20) was purchased from Sigma–Aldrich (St. Louis, MO). Cetyltrimethylammonium bromide (CTAB, [CH3(CH2)15N(CH3)3]Br) and 1,3,5-trimethylbenzene (TMB) were procured from Wako Pure Chemical Industries (Tokyo, Japan). Tetraethoxysilane (TEOS) was obtained from Shin-Etsu Chemical Co. (Tokyo, Japan). The protein-free blocking buffer (No. 37570) was acquired from Thermo Fisher Scientific Inc. (Waltham, MA). Bisphenol A was obtained from Kanto Chemical Co. (Tokyo, Japan). Bisphenol A polyclonal antibody was gifted by Japan EnviroChemicals (Osaka, Japan). The Bio-Rad Protein Assay Kit (500-0006) was purchased from Bio-Rad Laboratories (Hercules, CA).
2.2 Synthesis of mesoporous silica materials
2.2.1 Synthesis of Mobil Crystalline Material MCM-41
MCM-41 was synthesised using CTAC as an organic template. CTAB (2.6 g) was added to an aqueous HCl solution (pH 0.5, 30 mL) and stirred for 2 h at room temperature. TEOS (3.5 g) was added to the solution and the mixture was stirred for 5 h. Ammonia (3.0 g, 28 wt%, 14.7 M) was added at one time. After standing for 24 h, the white precipitate was filtered, successively washed with deionised water (50 mL) and ethanol (50 mL) and dried overnight at room temperature. Finally, the sample was calcined at 500 °C in air for 6 h (heating rate: 1 °C min−1) to remove the organic template.
2.2.2 Synthesis of Santa Barbara Amorphous SBA-15-1
SBA-15-1 was synthesised using P123 as an organic template [Citation37,Citation38]. P123 (1 g) was dissolved in 1.07 M hydrogen chloride (HCl, 35 mL) and the solution was stirred for 2 h at room temperature to give SBA-15-1. n-Decane (7.6 g) was added to the solution and the mixture was stirred for 2 h at room temperature. After addition of NH4F (11.5 mg) and TEOS (2.13 g), the solution was stirred for 1 h at room temperature and kept at 40 °C for 20 h using a temperature controlled bath. The solid product was collected by filtration, washed and dried overnight at room temperature. Organic template removals by calcination were performed through the same procedure as for MCM-41.
2.2.3 Synthesis of SBA-15-2
P123 (1 g) was dissolved in 1.07 M HCl (35 mL) and the solution was stirred for 2 h at room temperature. The solution was rapidly mixed with TMB (5 g) to expand pore sizes and the mixture was stirred for 3 d at 25 °C [Citation39]. NH4F (11.5 mg) and TEOS (2.1 g) were successively added to the mixture which was stirred for 1 h at room temperature. The resulting solution was further stirred at 40 °C in a temperature controlled bath for 24 h before being transferred to an autoclave and heated at 100 °C for 48 h. The solid product was collected by filtration, washed and dried at 70 °C for 5 h. P123 and TMB were removed by calcination using a procedure similar to that used for MCM-41.
2.3 Material characterisation
The specific surface area and pore volume of materials were measured by gas adsorption following the Brunauer–Emmett–Teller (BET) method using a Tristar3000 micrometrics analyser (Shimazu Co., Kyoto, Japan). Small-angle X-ray diffraction (XRD) spectra were recorded using a Rigaku RINT-TTR diffractometer (CuKα, 50 kV, 300 mA) and wide-angle XRD spectra were measured on a Rigaku RINT2000/PC (FeKα, 40 kV, 30 mA). Sample morphologies were examined by field emission scanning electron microscopy (FE-SEM; S-4300, Hitachi Co., Tokyo, Japan) with an accelerating voltage of 10.0 kV. Before the FE-SEM observation, the samples were coated with platinum using a Hitachi E-1020 ion sputtering system (Hitachi High-Technologies Co., Tokyo, Japan). Transmission electron microscopy (TEM) images were acquired using JEOL JEM 2010 electron microscope (JEOL Co., Tokyo, Japan) operating at 200 kV.
2.4 BPA antibody adsorption on MPS
MPS material (10 mg) was added to a solution of BPA antibody (500 μg) in 10 mM phosphate buffer (1 mL, pH 7). This mixture was kept at 4 °C for 12 h to allow the BPA antibody to adsorb on the MPS surface and centrifuged at 3000 rpm for 5 min. A Bio-Rad Protein Assay reagent (200 μL) was mixed with the supernatant (10 μL) for 20 min at room temperature to determine the protein concentration, as recommended by the supplier. The amount of adsorbed antibody was measured by a spectrophotometric method using bovine serum albumin as the standard.
2.5 BPA antibody–antigen reaction on MPS
MPS (10 mg) was added to a solution of BPA antibody (500 μg) in 10 mM phosphate buffer (1 mL, pH 7). The mixture was kept at 4 °C for 12 h for BPA antibody adsorption. After solvent removal, BPA (1 mL, 500 ng mL−1), used as an antigen, was added to the solid and kept at 37 °C for 3 h (). The reaction mixture was centrifuged and the unbound antigen present in the supernatant was isolated and analysed by high-performance liquid chromatography (HPLC). HPLC was performed using a Shimadzu LC 10-AD instrument equipped with an ultraviolet detector set at 210 nm and a Wakosil-II 5C18HG column (Wako Pure Chemicals, Osaka, Japan) eluted with an acetonitrile–water mobile phase (8.0/2.0; v/v) at a flow rate of 0.15 mL min−1. The retention time of BPA was 16.7 min. Each reported value was the mean of at least three experiments.
2.6 BPA recovery
To evaluate the solvent effects on BPA recovery rates, the MPS–BPA antibody–BPA composite was kept in 70%, 80%, 90% or 100% acetonitrile solution (1 mL) in 10 mM phosphate buffer (pH 7) at room temperature for 3 h to cleave the antigen–antibody interactions. The recovered BPA was measured through the procedure described in Section 2.5.
2.7 Recyclability of MPS immobilised BPA antibody
The reusability of immobilised antibodies is an important consideration for industrial applications. To examine the residual activity of BPA antibody, the procedures outlined in Section 2.5 were repeated up to three times using recovered MPS–BPA antibody composites.
2.8 BPA concentration
To enrich the BPA sample, a 50 ng mL−1 BPA solution was first prepared. This BPA solution (1 mL) was mixed with the MPS–BPA antibody composite and maintained at 37 °C for 3 h. This process was repeated four times to increase the BPA concentration. Next an 80% acetonitrile solution (1 mL) in 10 mM phosphate buffer (pH 7) was added to the MPS–BPA antibody–BPA composites to release BPA. The recovered BPA was measured through the same procedure as mentioned in Section 2.5.
3 Results and discussion
3.1 Characterisation of MPS
MPS morphologies and structures were characterised through SEM, TEM and nitrogen (N2) adsorption/desorption analyses. Shape is known to play an important role in the behaviour of MPS beads as protein carriers [Citation40]. SEM images showed that MCM and SBA materials formed aggregated polyhedral particles with average particle sizes of 50 × 200 nm (MCM-41, a), 400 × 200 nm (SBA-15-1, c) and 1000 × 800 nm (SBA-15-2, e). TEM analysis showed that the MPS material displayed a high degree of structural order (). Surface area and average pore diameter are known to impact the immobilisation and activity of supported antibodies. Therefore, N2 adsorption/desorption measurements (a) were performed for each MPS and the Barrett–Joyner–Hallenda model (b) was applied to the adsorption isotherm for MCM-41, SBA-15-1, and SBA-15-2. This approach typically underestimates the surface area, pore volume and diameter of mesopores but is widely used. All of the isotherms were type IV curves. The isotherm of MCM-41-1 indicated a reversible adsorption–desorption process with a characteristic nitrogen condensation evaporation step at a relative pressure of ca. 0.3, and the volume adsorbed was 1.5 cm3 (STP) g−1. Meanwhile, the isotherms of SBA-15-1 and SBA-15-2 exhibited the same step at relative pressures of ca. 0.75 and 0.84, with adsorbed volumes of 2.0 and 2.7 cm3 (STP) g−1, respectively. b shows the pore size distribution curves of synthesised MCM-41, SBA-15-1, and SBA-15-2. The curves of MCM-41, SBA-15-1, and SBA-15-2 displayed sharp peaks at 2.7, 12.3, and 24.0 nm, respectively. Surface areas and pore volumes were deduced using the BET model (). All the samples exhibited relatively high surface areas (502–962 m2 g−1) and large pore volumes (1.5–2.3 cm3 g−1). Moreover, pore sizes ranged from 2.7 to 24.0 nm, indicating that the materials consisted of mesopores. All the samples were synthesised according to the methods in our previous reports [Citation41] and XRD patterns of the products showed similar profiles. The wide-angle XRD patterns of MCM-41 displayed three reflection peaks corresponding to (1 0 0), (1 1 0) and (2 0 0) planes, confirming the presence of ordered hexagonal mesoporous structures [Citation41,Citation42]. Small-angle XRD patterns showed three peaks corresponding to the (1 0 0), (1 1 0) and (2 0 0) reflections of two-dimensional hexagonal mesostructures for SBA-15-1 [Citation43–Citation45] but a broad peak for SBA-15-2. This suggests that the addition of TMB to P123 formed a mesocellular silica foam [Citation46].
Table 1 Structural properties of mesoporous silicates prepared in this manuscript.
3.2 Immobilisation study
3.2.1 BPA antibody adsorption on MPS and BPA binding activity of the MPS-immobilised BPA antibody
Antibodies molecules are Y-shaped and consist of four polypeptide chains: two identical heavy chains and two identical light chains connected by disulphide bonds. The under part of the Y is called fragment crystallisable (Fc fragment), which has a molecular size of 3–5 nm. The upper part of the Y is called fragment antigen binding (Fab fragment), which has a molecular size of 9–11 nm and recognises specific foreign objects [Citation41,Citation47]. The relative adsorbed rate of BPA antibody was determined for MCM-41 (2.7 nm), SBA-15-1 (12.3 nm) and SBA-15-2 (24.0 nm) using the Bradford assay (, solid bar). In this assay, MPS (10 mg) was added to a solution of BPA antibody (500 μg mL−1, 1 mL). The absorbance of BPA antibody (50 μg) was assumed to be the adsorption rate of the antibody 100%. We analysed the amount of BPA antibody in the supernatant and decided the relative adsorption rate. All MPSs adsorbed over 80% (40 μg mg−1) of the BPA antibody. In the case of the MCM-41 (2.7 nm), the Fc fragment which has a molecular size of 3–5 nm, fit into the pore. On the contrary, the Fab fragment which has a molecular size of 9–11 nm absorbed inside the SBA-15-1 (12.3 nm) and SBA-15-2 (24.0 nm); therefore, the adsorption amount of antibodies was a similar to three MPS.
Fig. 4 Relative BPA antibody adsorption on MPS and BPA binding activity of MPS-immobilised BPA antibody.
Relative adsorption rate (%) = 100 × (absorbance of BPA antibody − absorbance of unbound antibody in the supernatant)/absorbance of BPA antibody.
Relative binding rate (%) = 100 × (absorbance of unbound antigen in the supernatant on the MCM-41-BPA antigen system − absorbance of unbound antigen in the supernatant on the MPS–BPA antibody–BPA antigen system)/absorbance of unbound antigen in the supernatant on the MCM-41–BPA antigen system.
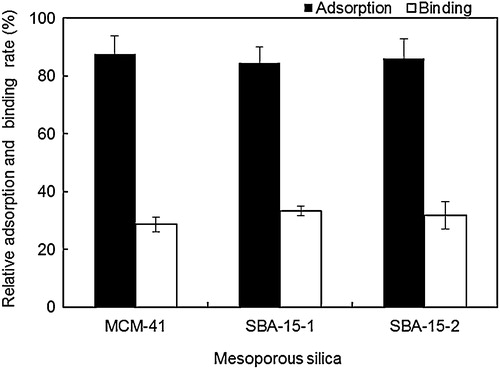
Subsequently, the relative binding ratio between the MPS-immobilised antibody and BPA was analysed (, open bar). In this assay, MPS–BPA antibody composite (10 mg) was added to a solution of BPA (500 ng mL−1, 1 mL). The HPLC peak height of BPA (50 ng mL−1) was set as 100% binding activity. We analysed the amount of BPA in the supernatant by HPLC and decided the relative binding rate. BPA amounts of 14, 17 and 16 ng adsorbed on 1 mg of MCM-41, SBA-15-1 and SBA-15-2-based MPS–antibody composites, respectively. Therefore, the immobilised antibody exhibited BPA binding activities of 28%, 33% and 32% on MCM-41, SBA-15-1 and SBA-15-2, respectively. These results indicate that MPS pore size and morphology did not significantly affect the BPA antibody adsorption and binding activity of the resulting MPS–antibody composites.
3.2.2 Effects of acetonitrile concentration on BPA recovery
Organic solvents strongly affect the BPA recovery rates of the BPA–MPS system. Therefore, the MPS–BPA antibody–BPA composites were maintained in 70%, 80%, 90% and 100% acetonitrile solutions in 10 mM phosphate buffer (pH 7). As shown in , the 80% acetonitrile solution provided relative BPA recovery rates of almost 100% for all the MPSs. Relative recovery rates were high only for MCM-41 (90%) and SBA-15-2 (100%) with 70% acetonitrile. In contrast, for 90% and 100% acetonitrile solutions, these relative recovery rates decreased to 70–90%. Relative recovery rates were found to strongly depend on the acetonitrile concentration. The Fab fragment in the BPA antibody displayed a greatly reduced activity in 90% and 100% acetonitrile solutions compared to 70% and 80% solutions because its structure changed at high acetonitrile concentrations. Reactions were therefore performed under optimum conditions (80% acetonitrile) in the following recyclability and BPA enrichment experiments.
3.2.3 Effects of reuse on BPA antibody activity
The residual activity of the MPS-immobilised BPA antibody was examined after multiple antigen binding–recovery cycles. shows the residual activities of MPS–BPA antibody composites for BPA after three recycles relative to the original activity before reuse (taken as 100%). The residual activities after three recycles for SBA-15-1 and SBA-15-2 amounted to 32% and 30%, respectively. BPA antibody immobilised on MCM-41 displayed a lower residual activity (13%) after three recycles. It is understood that the immobilised antibodies in the extremely large pores did not get easily deactivated by the acetonitrile treatment because entire molecules of antibodies absorbed inside the pores; therefore, the structural conformation of the antibody was greatly stabilised.
3.2.4 Effect of MPS on the enrichment of BPA traces
To concentrate BPA, the antigen–antibody reaction was repeated four times using 50 ng mL−1 BPA for a total adsorption of 200 ng on the MPS–BPA antibody composites. Relative recovery rates for SBA-15-1 and SBA-15-2 were equal to 82.4% (165 ng) and 79.3% (159 ng), respectively (). On the other hand, the rate was markedly reduced for MCM-41 (42.3%). These results suggest that multiple soakings, separations and washing steps involved in recycling easily strip the BPA antibody from MCM-41 pores, because only the Fc fragment of the antibody adsorbed on the support. BPA antibody presented relatively weaker interaction forces with MCM-41 compared with SBA-15-1 and SBA-15-2 consisting of larger pores, which resulted in a reduced relative recovery rate for MCM-41.
4 Conclusions
Three types of MPS – MCM-41 (2.7 nm), SBA-15-1 (12.3 nm) and SBA-15-2 (24.0 nm) – were used for BPA binding experiments. Investigations of BPA antibody immobilisation and antibody–antigen reaction showed that all MPSs adsorbed almost the same amount of BPA antibody (approximately 80%) and exhibited similar binding rates (approximately 30%). BPA recovery rates from 70%, 90% and 100% acetonitrile solutions were significantly reduced, but were the highest when 80% acetonitrile was used. After three binding–recovery cycles, the immobilised BPA antibody retained over 30% of its initial activity on SBA-15-1 and SBA-15-2. This result indicated that entire antibody molecules were adsorbed inside the pores, greatly stabilising their conformational orientation.
These findings open new and interesting prospects for ordered mesoporous materials, and provide important information on their ability to detect and recover ultra-low concentration compounds in microanalysis systems.
Notes
Peer review under responsibility of The Ceramic Society of Japan and the Korean Ceramic Society.
References
- C.T.KresgeM.E.LeonowiczW.J.RothJ.C.VartuliJ.S.BeckNature3591992710712
- S.TakahashiY.IkkaiK.SakamotoC.R.AbreuK.AramakiJ. Colloid Interface Sci.33520097076
- K.NakagawaH.MatsuyamaT.MakiM.TeramotoN.KubotaSep. Purif. Technol.442005145151
- B.TatineniS.ManickamM.HideyukiAnal. Bioanal. Chem.3842006488494
- M.HartmannA.VinuLangmuir18200280108016
- D.Perez-QuintanillaI.D.HierroM.FajardoI.SierraMicroporous Mesoporous Mater.8920065868
- J.F.DiazK.J.BalkusJr.J. Mol. Catal. B: Enzym.21996115126
- L.Washmon-KrielV.L.JimenezK.J.BalkusJr.J. Mol. Catal. B: Enzym.102000453469
- J.DeereE.MagnerJ.G.WallB.K.HodnettChem. Commun. (Camb.)4620015466
- A.P.WightM.E.DavisChem. Rev.102200235893614
- A.SteinAdv. Mater.152003763775
- X.G.WangK.S.K.LinJ.C.C.ChanS.ChengJ. Phys. Chem. B109200517631769
- M.M.CollinsonMikrochim. Acta1291998149165
- V.S.Y.LinC.Y.LaiJ.HuangS.A.SongS.XuJ. Am. Chem. Soc.12320011151011511
- K.MuraiM.HiguchiT.KinoshitaK.NagataK.KatoPhys. Chem. Chem. Phys.1520131145411460
- Y.S.LinC.L.HaynesChem. Mater.21200939793986
- U.T.BornscheuerAngew. Chem. Int. Ed.42200333363337
- I.GillE.PastorA.BallesterosJ. Am. Chem. Soc.121199994879496
- W.JinJ.D.BrennanAnal. Chim. Acta4612002136
- K.NakanishiM.TomitaH.NakamuraK.KatoJ. Mater. Chem. B1201363216328
- Y.MasudaS.KugimiyaK.MuraiA.HayashiK.KatoColloids Surf. B: Biointerfaces10120132633
- Y.MasudaS.KugimiyaY.KawachiK.KatoRSC Adv.4201435733580
- K.NakanishiM.TomitaK.KatoRSC Adv.4201447324735
- H.SanbeJ.HaginakaJ. Pharm. Biomed. Anal.30200218351844
- K.TakedaT.KobayashiJ. Membr. Sci.27520066169
- K.TakedaT.KobayashiSci. Technol. Adv. Mater.62005165171
- L.N.VandenbergR.HauserM.MarcusReprod. Toxicol.242007139177
- H.SambeK.HoshinaK.HosoyaJ.HaginakaJ. Chromatogr. A113420061623
- T.StoichevM.S.BaptistaM.C.P.BastoP.N.CarvalhoM.T.S.D.VasconcelosAnal. Bioanal. Chem.3912008425432
- M.KawaguchiR.ItoN.OkanouchiK.SaitoH.NakazawaJ. Chromatogr. B870200898102
- C.M.ChangC.C.ChouM.R.LeeAnal. Chim. Acta53920054147
- A.KimC.R.LiC.F.JinK.W.LeeS.H.LeeK.J.ShonN.G.ParkD.K.KimS.W.KangY.B.ShimJ.S.ParkChemosphere68200712041209
- Y.WatabeT.KondoM.MoritaN.TanakaJ.HaginakaK.HosoyaJ. Chromatogr. A103220044549
- F.S.von SaalP.S.CookeD.L.BuchananP.PaianzaK.A.ThayerS.C.NagelS.ParmigianiW.V.WelshonsToxicol. Ind. Health141998239260
- J.S.FisherK.J.TurnerD.BrownR.M.SharpeEnviron. Health Perspect.1071999397405
- R.SteinmetzN.A.MitchnerA.GrantD.L.AllenR.M.BigsbyN.Ben-JonathanEndocrinology138199717801786
- D.ZhaoJ.FengQ.HuoN.MeloshG.H.FredicksonB.F.ChmelkaG.D.StuckyScience2791998548552
- D.Y.ZhaoQ.S.HuoJ.L.FengB.F.ChmelkaG.D.StuckyJ. Am. Chem. Soc.120199860246036
- W.W.LukensJr.P.YangD.I.MargoleseJ.S.LettowJ.Y.YingG.D.StuckyChem. Mater.122000686696
- J.FanJ.LeiL.WangC.YuB.TuD.ZhaoChem. Commun.17200321402141
- T.OritaK.KatoM.TomitaJ. Ceram. Soc. Jpn.11920112388245
- S.BizM.L.OccelliCatal. Rev. Sci. Eng.401998329407
- V.MeynenP.CoolE.F.VansantMicroporous Mesoporous Mater.1252009170223
- C.K.KrishnanT.HayashiM.OguraAdv. Mater.20200821312136
- O.Y.GutiérrezF.PérezG.A.FuentesX.BokhimiT.KlimovaCatal. Today1302008292301
- P.H.PandyaR.V.JasraB.L.NewalkarP.N.BhattMicroporous Mesoporous Mater.7720056777
- L.J.HarrisE.SkakeyskyA.McPhersonJ. Mol. Biol.2751998861872