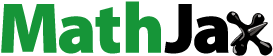
Abstract
Laminated Ti/Al2O3 composite was fabricated by tape casting and vacuum hot-pressing sintering with intent to research mechanical properties including the fracture strength and toughness. The results showed that the flexural strength and fracture toughness improved according to the amount of layers. When the amount of layers was 35, the flexural strength and fracture toughness reached 361 MPa and 9.72 MPa m1/2, respectively. Based on the observation of microstructures, it was concluded that the improvement of mechanical properties was attributed to various crack propagation (such as crack deflection, crack bifurcation, crack termination and interface dissociation). The characterization of EDX and XRD at interfaces indicated that the initiative diffusion of O2− from Al2O3 to Ti layer led to the passive diffusion of Al3+ (aggregating near the interface) and reacted with Ti to form Ti3Al, Ti[O] and AlTiO2 solid solution.
1 Introduction
At present, laminated ceramic composite is reported to enhance fracture toughness of ceramic composite that can be used in applications ranging from cutting tool to aircraft components [Citation1–Citation3]. Laminated metal/ceramic composite has attracted considerable attention due to its special structure and excellent mechanical properties [Citation4,Citation5]. As is reported, because of crack deflection, laminated metal/ceramic composite performs higher mechanical properties than monolithic metal/ceramic composite [Citation6–Citation9]. In addition, owing to the strong reducibility of metal, element diffusion of metal/ceramic interface and interfacial reaction has a great influence on microstructures of composite [Citation10,Citation11].
Fig. 2 Flexural strength and fracture toughness of laminated Ti/Al2O3 composite with different amount of layers.
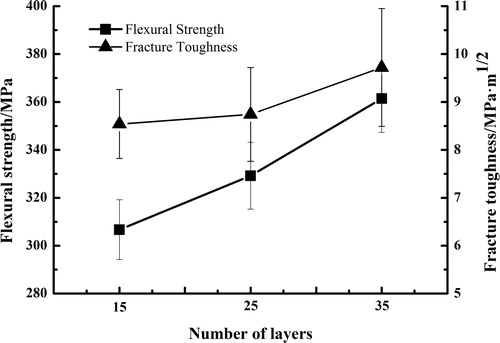
Fig. 4 The crack branch, crack deflection (a) and crack deflection angle (b) of laminated Ti/Al2O3 composite after the bending test.
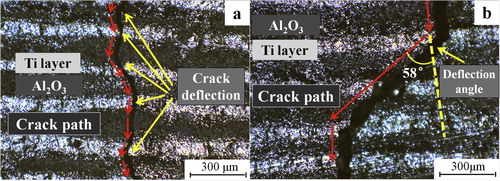
Fig. 5 Crack bifurcation (a), crack termination (b) and interface dissociation (c) in crack propagation paths.
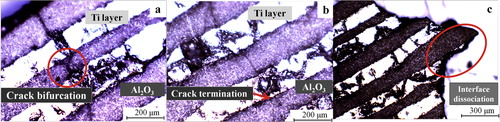
Fig. 6 SEM micrographs (a–c) of sample fracture and surface and element diffusion analysis of EDX: (d) Ti Kα1; (e) Al Kα1 and (f) O Kα1.
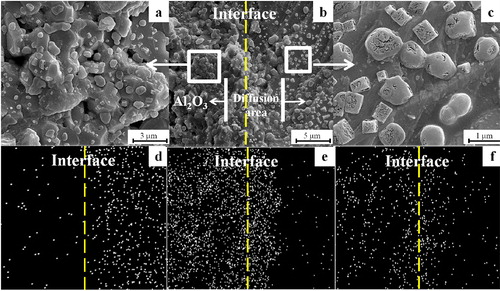
By the reason of similar thermal expansion and good chemical compatibility of Ti and Al2O3, Ti/Al2O3 ceramic composite has attracted much attention due to the cheap raw materials, simple preparation process and superior properties. It is used as promising materials for cutting tools, anti-corrosion coating and electrical devices due to the good mechanical properties and superior creep resistance [Citation12]. However, an irreconcilable conflict between hardness and strength existed in this type of tool materials. In order to solve the problem, laminated Ti/Al2O3 composite is synthesized instead of monolithic composite in this paper.
Although many studies had been conducted on the metal/Al2O3 laminated composite [Citation13], the fabrication, mechanical properties and crack propagation behaviors of laminated Ti/Al2O3 composite is rarely investigated. In this study, the mechanical properties (flexural strength and fracture toughness) of laminated Ti/Al2O3 composite with different amount of layers are researched. The fracture behaviors of laminated composite are detected through load/displacement curve and observation of crack propagation paths in laminated Ti/Al2O3 composite. In the end, the element distribution of interface between Ti and Al2O3 layers were characterized.
2 Experimental procedure
Al2O3 (99.99% pure, an average particle size of 1 mm) and Ti (99.99% pure, an average particle size of 5 mm) powders were used as raw materials of tape casting. Firstly, the organic binder sol of 5 wt% PVB (polyvinyl butyral) was dissolved by ethanol with plasticizing agent of 5 wt% PEG2000 (polyethylene glycol). Then powders of 20 wt% Ti (or Al2O3) were mixed in the organic binder sol and dispersed by ultrasonic for 2 h respectively. Secondly, the mixed sol was put into tape casting mold at room temperature for 6 h. Then, the green films were cut into slices (a) and stacked periodically (b) to remove organic binder at 650 °C for 2 h. A graphite die 45 mm in inner diameter was filled with the green films, followed by sintering using vacuum hot-pressing apparatus (VVPgr-80-2300, China) at 1420 °C under an applied pressure of 30 MPa for 60 min. Finally, the samples (c) were cut into bars with a width of 5 mm to detect the mechanical properties and microstructure.
The size of the samples with different amount of layers (15, 25 and 35) for mechanical testing was 3 mm × 5 mm × 30 mm, 5 mm × 5 mm × 30 mm and 7 mm × 5 mm × 30 mm. The flexural strength of laminated composite was achieved by three-point bending method with a crosshead speed of 0.5 mm min−1 and span of 30 mm at room temperature using a electromechanical universal testing machine (CMT5504, MTS SYSTEMS, Co., Ltd., China). Fracture toughness was determined by single-edge notched beam test with a crosshead speed of 0.05 mm min−1. Crack propagation paths were observed by metallographic microscope (BA310MET, China). The microstructure was analyzed by a scanning electron microscopy (SEM) (FEI QUANTA FEG 250, United States) equipped with energy dispersive X-ray analysis (EDX).
3 Results and discussion
Flexural strength and fracture toughness of laminated composite with different amount of layers are shown in . It can be observed that the broken line graph emerges gradually increasing tendency of flexural strength and fracture toughness with the increase of layers. When the amount of layers is 35, the flexural strength and fracture toughness of laminated Ti/Al2O3 composite reach 361 MPa and 9.72 MPa m1/2, respectively, which are 1.24 and 2.03 times that of pure Al2O3 ceramic. The results of mechanical property testing identified that strength and toughness are closely related to the amount of layers. Chartier et al. [Citation14] and Zuo et al. [Citation15] proposed a simple formula of the residual compressive stress (σ1) which is as follows,(1)
(1) where E1, E2, α1, α2, ν1, ν2, d1 and d2 are the young modulus, thermal expansion coefficient, Poisson's ratio and layer thickness of ceramic and metal, respectively. ΔT and n are the difference temperature between the actual and the joining temperature and the amount of composite layers. It can be concluded that the increase of n induces the enhancement of residual compressive stress, which leads to the improvement of mechanical properties of laminated composite.
is the load–displacement curve of laminated Ti/Al2O3 composite composed of 35 layers. Distinguished from the typical brittleness fracture behavior of pure Al2O3 ceramic, laminated Ti/Al2O3 composite indicates a non-catastrophic fracture method. After the maximum flexural load, the curve of load–displacement changes into step-like load instead of typical brittle fracture.
The crack propagation paths of laminated sample are clearly observed by metallographic microscope after three-point bending test. As shown in a, a crack propagates through the specimen with five obvious crack deflections appearing in Ti layers, which is mainly attributed to the plastic deformation in Ti layers to deflect the crack propagation path [Citation16]. An obvious crack deflection angle observed in b was 58°, according to the trigonometric function relationship, which means that the length of actual crack propagation path is 1.88 times that of theoretical vertical crack without any deflection (always occurred in monolithic ceramic). The critical stress of crack tip is estimated using Griffith's formula [Citation17]:(2)
(2) where σc is the critical stress of crack tip, E is Young's modulus, γ and γp are the constants of fracture surface energy of materials, and c is the length of crack. Due to the extension of length of crack propagation paths (shown in b), according to the Griffith's formula, the critical stress reduced to be 0.72 times that of theoretical vertical crack propagation paths. Therefore, the larger crack deflection angle, which is the same as longer length of crack propagation paths, will decrease more critical stress of crack tip and make laminated Ti/Al2O3 composite more difficult to rupture. The results of mechanical testing and estimation of Griffith's formula both demonstrate that the crack deflections and longer length of crack propagation paths were effective to improve mechanical properties of laminated composite.
Besides crack deflection, the crack bifurcation, crack termination and interface dissociation still exist in crack propagation paths (). The crack bifurcation, which can extend crack propagation paths doubly, is observed in laminated Ti/Al2O3 composite (a). Because of the energy consumption of tensile metal Ti layer, critical stress of crack tip decreases gradually with the crack traversing through each layer. When critical stress of crack tip is insufficient to break through Al2O3 layer, crack is terminated (b) and it prevents catastrophic rupture of laminated composite. Likewise, interface dissociation (c) apparently occurs in interface of adjacent layers to lengthen crack propagation paths along with interface. In order to evaluate the consumption of fracture energy in interface dissociation, the observation of interfacial diffusion region and characterization of interfacial reaction products were necessary.
3.1 Interfacial characterization
The fracture surface is detected by SEM and EDX, and the SEM micrograph with element distribution analyses of EDX is shown in . a and c is the partial magnification micrographs of b; the well-grown grain of Al2O3 and Ti spreading either side of the interface with distinct interface is observed clearly. With the purpose of researching diffusion phenomenon at the interfaces, laminated Ti/Al2O3 composite is determined by map element distribution analyses of EDX (d–f). The distribution of Al, Ti and O element shows different diffusion tendencies, it suggests that O traversed through interface for a further distance than Al, whereas Ti is kept in place.
The interface diffusion products of laminated Ti/Al2O3 composite are characterized by XRD patterns and the result is shown in . Besides the well-defined peaks of Ti and Al2O3, Ti–Al inter-metallic compounds (Ti3Al), titanium oxide (Ti[O]) and Al–Ti–O solid solution (AlTiO2) are found. Therefore, it is verified that laminated Ti/Al2O3 composite is composed of Ti3Al, Ti[O] (such as TiO and TiO2) and AlTiO2 solid solution phases among Ti and Al2O3 major phases. The results of EDX () and XRD () reveal that O2− diffused from Al2O3 layer to Ti layer and had an oxidation reaction with Ti element to form Ti[O]. In order to keep the charge balance of Al2O3 layer, Al3+ diffuse passively through interface and react with Ti to form Ti3Al inter-metallic compounds and AlTiO2 solid solution.
4 Conclusions
The mechanical properties of Ti/Al2O3 composite were improved owing to the laminated metal/ceramic structure. The results of mechanical properties tests indicated that the flexural strength and fracture toughness changed according to the amount of layers, and the maximal experimental values reached to 361 MPa and 9.72 MPa m1/2, respectively. Based on the observation of microstructure, it was concluded that the improvement of mechanical properties was attributed to various crack propagations (such as crack deflection, crack bifurcation, crack termination and interface dissociation). What is more, the characterization of EDX and XRD at interfaces indicated that the initiative diffusion of O2− from Al2O3 to Ti layer leads to passive diffusion of Al3+ (aggregating near interface) to react with Ti to form Ti3Al, Ti[O] and AlTiO2 solid solution.
Acknowledgement
Authors appreciate the financial support of the National Natural Science Foundation of China under the Grant No. 51372099, the Natural Science Foundation of Shandong Province (Grant No. ZR2012EML05) and the Doctoral Fund of University of Jinan (XBS1310). Authors also appreciate the financial support by Program for Scientific research innovation team in colleges and universities of Shandong Province.
Notes
Peer review under responsibility of The Ceramic Society of Japan and the Korean Ceramic Society.
References
- G.F.WangZ.LuC.W.WangQ.W.RenK.F.ZhangPowder Technol.2142011188193
- P.ZhouP.HuX.H.ZhangW.B.HanScr. Mater.642011276279
- T.SekinoY.J.HunJ. Ceram. Soc. Jpn.10862000541547
- P.KerdkoolS.NiyomwasProc. Eng.322012642648
- K.H.ZuoD.L.JiangQ.L.LinY.P.ZengMater. Sci. Eng. A – Struct.4432007296300
- W.J.CleggK.KendallN.M.AlfordT.W.ButtonJ.D.BirchallNature34741990455457
- S.TariolleF.ThévenotT.ChartierJ.L.BessonJ. Eur. Ceram. Soc.25200536393647
- G.B.YangaY.J.LiuG.J.QiaoJ.F.YangH.J.WangMater. Sci. Eng. A – Struct.49200823272332
- C.WuZ.WangG.P.ShiQ.G.LiY.MaL.H.LiuJ. Univ. Jinan – Sci. Technol.28120142731
- X.ZhangG.LuM.J.HoffmannR.MetselaarJ. Eur. Ceram. Soc.151995225232
- G.DehmC.ScheuM.RuhleR.RajActa Mater.4631998759772
- W.T.KwonJ.S.ParkS.W.KimS.KangInt. J. Mach. Tools Manuf.442004341346
- Z.ChenJ.J.MecholskyJ. Am. Ceram. Soc.765199312581264
- T.ChartierD.MerleJ.L.BessonJ. Eur. Ceram. Soc.151995101107
- K.H.ZuoD.L.JiangQ.L.LinMater. Lett.60200612651268
- K.HbaiebR.M.McMeekingF.F.LangeInt. J. Solids Struct.44200733283343
- A.A.GriffithPhilos. Trans., Ser. A2211920163198