Abstract
Red emitting phosphors based on titanite, (Ca1−x−yEuxNay)TiSiO5+δ (x = 0.05, 0.07, 0.10; y = 0, 0.01, 0.03, 0.05), were synthesized by the conventional solid state reaction method, and their photoluminescence properties were investigated. Among (Ca1−xEux)TiSiO5+δ (x = 0.05, 0.07, 0.10), (Ca0.93Eu0.07)TiSiO5+δ (x = 0.07) showed the highest emission intensity of 14% in comparison with the commercial (Y0.98Eu0.02)2O2S phosphor. Introduction of monovalent Na+ into the (Ca0.93Eu0.07)TiSiO5+δ lattice also enhanced the emission intensity by preventing the reduction of Ti4+ to Ti3+. Consequently, the (Ca0.90Eu0.07Na0.03)TiSiO5+δ phosphor enhanced the emission intensity up to 22%. From the thermal analysis results, it is revealed that the thermal stability of the present (Ca0.90Eu0.07Na0.03)TiSiO5+δ phosphor was much higher than that of the commercial one.
1 Introduction
White light-emitting diodes (WLEDs) are used for various applications such as solid state lighting sources and display devices, because of the high brightness, low energy consumption, and long lifetime [Citation1]. Among several ways for fabricating the WLEDs, one that is composed of a near-ultraviolet (NUV) LED chip and red, green, and blue phosphors applied on the chip has been widely studied, because this type of WLED has high color-rendering index. Eu3+-doped Y2O2S oxysulfide is popularly used as a red phosphor for the WLEDs; however, its thermal stability is low. In addition, oxysulfide compounds are decomposed to oxides at high temperatures, accompanying with generation of toxic sulfur oxides. In contrast, oxide compounds are considered to be ideal materials for such phosphors from a practical perspective, because oxide compounds usually hold higher thermal stability than other compounds such as oxysulfide.
It is well known that the luminescent properties of phosphors significantly depend on the interaction between the activators in the host material. Generally, luminescent emission intensity enhances with increasing the concentration of the activator in the host lattice. However, redundant amount of the activator would cause concentration quenching and lowering the luminescent emission intensity, because the decrease in the mean distance between the activators often induces non-radiative deactivation. Therefore, it is important to choose the crystal structure of the host material for designing a novel phosphor with high luminescent efficiency.
Among various candidates for new host materials, our research group has focused on several compounds with layered structure. In the layered structure, energy transfer between the excited luminescent ions across the anion groups was blocked by the long distance of rare earth ions of activators in the crystal structure. Therefore, such phosphors with the layered structure would be resistant to the concentration quenching, even if a large amount of activator was introduced into the host lattice. Considering this, our research group has successfully synthesized several novel phosphors with layered structure, and has revealed that these layered compounds are suitable for host materials of phosphors with high luminescent property [Citation2–Citation5].
In this study, we focused on a CaTiSiO5 with a titanite structure (space group: P21/c), which is thermally stable (melting point: 1373 °C [Citation6]) and consists of abundant elements, such as Ca, Ti, Si, and O, as a host material for phosphor. In the titanite structure, chains of corner-shared TiO6 units are cross-linked by SiO4 units and compose rigid [TiOSiO4]2− frameworks. These frameworks enclose the Ca2+ ions in 7-fold coordination [Citation7]. Therefore, by introducing Eu3+ ion into the titanite structure, red emitting phosphors would be provided with heat-resistance property. Here, in the literature, Pr3+ doped titanite single crystals have been synthesized by Czochralski method, and partial reduction of Ti4+ to Ti3+, which was induced by substitution of divalent Ca2+ ions with trivalent Pr3+ ions, was inhibited by codoping Na+ [Citation7]. It is well known that generation of Ti3+ may decrease the photoluminescence emission intensity, due to d–d transition of Ti3+ in red light region. From these concepts, in order to develop the red emitting phosphors with high thermal stability, the titanite (Ca1−x−yEuxNay)TiSiO5+δ powders were prepared by introducing Eu3+ and Na+ into the CaTiSiO5 with a conventional solid state reaction method. The photoluminescence properties of (Ca1−x−yEuxNay)TiSiO5+δ were then investigated.
2 Experimental
(Ca1−x−yEuxNay)TiSiO5+δ (x = 0, 0.05, 0.07, 0.10; y = 0, 0.01, 0.03, 0.05) solids were prepared by a conventional solid state reaction technique. Stoichiometric amounts of CaCO3, TiO2 (rutile), SiO2, Eu2O3, and Na2CO3 powders were mixed in an agate mortar, followed by mechanical mixing using a planetary-type ball-milling apparatus (PULVERISETTE 7, Fritsch GmbH) at a rotation speed of 300 rpm for 3 h. The homogenous mixture was calcined at 1300 °C for 4 h under flowing the synthetic air (N2:O2 = 79:21). Before characterization, all samples were gently ground in an agate mortar.
The samples were characterized by X-ray powder diffraction (XRD; SmartLab, Rigaku) with Cu-Kα radiation (40 kV and 30 mA). The lattice volumes of (Ca1−x−yEuxNay)TiSiO5+δ were calculated from the XRD peak angles, which were refined using α-Al2O3 as a standard. X-ray photoelectron spectroscopy (XPS; PHI5000 VersaProbeII, ULVAC-PHI) was performed at room temperature with Al-Kα radiation (1486.6 eV). The charging effect on the binding energies was corrected with respect to the C 1s peak (284.6 eV). Particle morphologies were observed by a scanning electron microscopy (SEM; SS-550, Shimadzu). Photoluminescence excitation and emission spectra were measured at room temperature using a spectrofluorophotometer (RF-5300PC, Shimadzu) with a Xe lamp as light source. The emission spectra were obtained for excitation at 393 nm, and the excitation spectra were recorded for the emission at 614 nm. Relative emission intensities of the (Ca1−x−yEuxNay)TiSiO5+δ phosphors were evaluated by comparing the integrated area of the emission peak at 614 nm for Eu3+ with that of the emission peak at 626 nm for Eu3+ of a commercial (Y0.98Eu0.02)2O2S phosphor (for excitation at 333 nm). Internal quantum efficiencies of some phosphors were measured on a spectrofluorometer (FP-8500, Jasco) with a fluorescence integrate sphere unit (ILF-835, Jasco) using a Xe lamp as light source, and the data were corrected by deuterium and halogen lamps. Differential thermal analysis (DTA; DTA-60AH, Shimadzu) was carried out in the temperature range between 50 and 1000 °C at a heating rate of 10 °C min−1 in the synthetic air atmosphere, where a α-Al2O3 powder in a platinum crucible was used as the counter reference material for the measurements. In addition, the prepared sample and the commercial (Y0.98Eu0.02)2O2S phosphor were heated at 1000 °C for 4 h under flowing synthetic air (N2:O2 = 79:21).
3 Results and discussion
shows the XRD patterns of the (Ca1−xEux)TiSiO5+δ samples. The samples with x ≤ 0.07 were indexed by a titanite single phase. On the other hand, for the sample with x = 0.10, a Eu2Ti2O7 was observed as an impurity phase in addition to the titanite phase. The lattice volume dependence on the Eu3+ content (x) of the (Ca1−xEux)TiSiO5+δ samples is presented in . The lattice volume decreased with increasing amount of the Eu3+ content (x) in the composition range of x ≤ 0.07, while it became approximately constant for the range of 0.07 ≤ x ≤ 0.10. These results indicate that a proportion of the Ca2+ ions in the CaTiSiO5 lattice was substituted with Eu3+, because ionic radius of Eu3+ (0.101 nm, 7-fold coordination) [Citation8] is smaller than that of Ca2+ (0.106 nm, 7-fold coordination) [Citation8].
depicts the photoluminescence excitation (a) and emission (b) spectra of the samples (x = 0.05, 0.07, 0.10). A weak broad band between 310 and 355 nm, and several sharp bands in wavelengths from 355 to 500 nm were recognized in the excitation spectra of all samples. The weak broad band corresponded to the charge transfer transition between Ti4+ and Eu3+ [Citation9], and the sharp bands originated in f–f transitions of Eu3+ between ground state and excited levels of 4f6 configuration. In the emission spectra, all peaks originated in the transitions from the 5D0 excited level to the 7FJ (J = 0, 1, 2, 3, and 4) ground levels of Eu3+. The emission peak corresponding to the 5D0–7F2 electric dipole transition at 614 nm was more dominant than those attributed to the 5D0–7F1 magnetic dipole transitions at 592 and 598 nm, indicating that the Eu3+ ions occupied the Ca2+ site with no inversion symmetry. Relative emission intensities of the (Ca1−xEux)TiSiO5+δ (x = 0.05, 0.07, 0.10) phosphors are plotted in . The relative emission intensity increased with increasing the Eu3+ content (x) for the (Ca1−xEux)TiSiO5+δ (x = 0.05 and 0.07) samples, while that for (Ca0.90Eu0.10)TiSiO5+δ (x = 0.10) decreased due to the formation of the Eu2Ti2O7 impurity phase. Consequently, the highest emission intensity was obtained for the (Ca0.93Eu0.07)TiSiO5+δ (x = 0.07) of 14% among the (Ca1−xEux)TiSiO5+δ (x = 0.05, 0.07, 0.10) phosphors. However, there is a possibility that d–d transition in the red region, occurring from Ti3+ ions, decreased the red emission intensity of (Ca0.93Eu0.07)TiSiO5+δ (x = 0.07). Trivalent Ti3+ ions were partially generated from tetravalent Ti4+ due to the charge compensation mechanism caused by the partial substitution of Ca2+ with higher valent Eu3+. Accordingly, codoping of monovalent Na+ is expected to enhance the photoluminescence property of the (Ca0.93Eu0.07)TiSiO5+δ phosphor by inhibiting the reduction of Ti4+ to Ti3+.
Fig. 3 Photoluminescence excitation (a) and emission (b) spectra of the (Ca1−xEux)TiSiO5+δ phosphors.
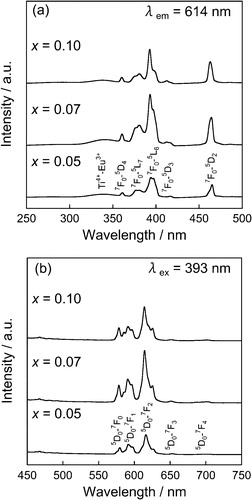
shows the XRD patterns of the (Ca0.93−yEu0.07Nay)TiSiO5+δ phosphors. The titanite single phase was obtained for all samples. depicts the lattice volume of the (Ca0.93−yEu0.07Nay)TiSiO5+δ (y = 0, 0.01, 0.03, 0.05) phosphors. Considering the difference of ionic radii of the cations [Ca2+ (0.106 nm) and Na+ (0.112 nm) for 7-fold coordination site, and Ti4+ (0.0605 nm) and Ti3+ (0.067 nm) for 6-fold coordination site] [Citation8], doping Na+ into the Ca2+ site of (Ca0.93Eu0.07)TiSiO5+δ would induce lattice expansion and lattice shrinkage simultaneously. As shown in , the lattice volume increased with increasing amounts of Na+ ions (y) for the (Ca0.93−yEu0.07Nay)TiSiO5+δ (y = 0.01, 0.03) samples. This fact indicates that the lattice expansion effect was predominant in this composition range and the Ti3+ was gradually oxidized to Ti4+. On the other hand, the lattice volume became approximately constant for the sample with 0.03 ≤ y ≤ 0.05. Therefore, it is assumed that the solid solutions were formed for the (Ca0.93−yEu0.07Nay)TiSiO5+δ (y = 0.01, 0.03) phosphors, and the partial reduction of Ti4+ to Ti3+ was inhibited, because of the charge compensation mechanism. For the sample of y = 0.05, since there were no extra lines evident in the XRD pattern (), it is considered that a trace amount of impurities containing Na+ ions were formed.
depicts the photoluminescence excitation (a) and emission (b) spectra of the (Ca0.93−yEu0.07Nay)TiSiO5+δ phosphors. As is the case with the (Ca0.93Eu0.07)TiSiO5+δ (y = 0) phosphor, a weak broad band (310–355 nm) and several sharp bands (355–500 nm) were observed in the excitation spectra for Na+ doped samples due to the charge transfer transition between Ti4+ and Eu3+ [Citation9] and the f–f transitions of Eu3+, respectively. The emission peaks corresponding to the transitions from the 5D0 excited level to the 7FJ (J = 0, 1, 2, 3, and 4) ground levels of Eu3+ were recognized in the emission spectra for these samples. The relative emission intensities of the (Ca0.93−yEu0.07Nay)TiSiO5+δ phosphors are shown in . The relative emission intensity increased with increasing of Na+ content (y) for the (Ca0.93−yEu0.07Nay)TiSiO5+δ (y = 0.01, 0.03) phosphors, and the highest emission intensity (22%) was obtained at y = 0.03. In contrast, the emission intensity decreased at the sample of y = 0.05, compared with that of y = 0.03. The internal quantum efficiency (QE) of the (Ca0.90Eu0.07Na0.03)TiSiO5+δ (x = 0.07, y = 0.03) was measured to be 22%, which was higher than that of the (Ca0.93Eu0.07)TiSiO5+δ (x = 0.07) (15%) without Na+ doping.
Fig. 7 Photoluminescence excitation (a) and emission (b) spectra of the (Ca0.93−yEu0.07Nay)TiSiO5+δ phosphors.
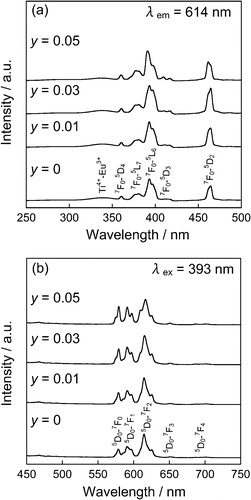
In order to confirm the decrease of Ti3+ ion by introducing Na+, the XPS spectra of Ti 2p core-level for the (Ca0.90Eu0.07Na0.03)TiSiO5+δ (x = 0.07, y = 0.03) and (Ca0.93Eu0.07)TiSiO5+δ (x = 0.07) phosphors were measured, and the results were given in . For the (Ca0.93Eu0.07)TiSiO5+δ (x = 0.07) ((b)), Ti 2p3/2 and Ti 2p1/2 peaks of trivalent Ti3+ (457.6 eV and 463.0 eV) [Citation10] were clearly observed, in addition to those of tetravalent Ti4+ (458.7 eV and 464.4 eV) [Citation11]. By introducing Na+, however, the peak intensities of the trivalent Ti3+ (Ti 2p3/2: 457.6 eV, Ti 2p1/2: 463.0 eV) [Citation10] decreased, as shown in (a). In fact, the ratio of the trivalent Ti3+ in the Ti3+/4+ ions was only 4% for the (Ca0.90Eu0.07Na0.03)TiSiO5+δ (x = 0.07, y = 0.03), which is explicitly lower than that for the (Ca0.93Eu0.07)TiSiO5+δ (x = 0.07) (14%). These results clearly indicate that the trivalent Ti3+ in the (Ca0.93Eu0.07)TiSiO5+δ was evidently oxidized to tetravalent Ti4+ by codoping of Na+ ion.
Fig. 9 XPS spectra of Ti 2p core-level for the (Ca0.90Eu0.07Na0.03)TiSiO5+δ (a) and (Ca0.93Eu0.07)TiSiO5+δ (b) phosphors.
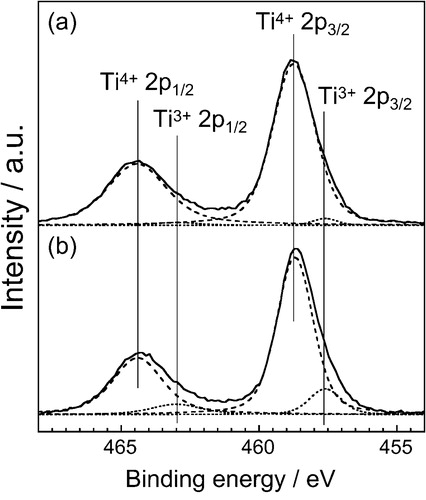
The SEM photographs for the (Ca0.90Eu0.07Na0.03)TiSiO5+δ (x = 0.07, y = 0.03) (a) and the (Ca0.93Eu0.07)TiSiO5+δ (x = 0.07) (b) phosphors are shown in . The particles of the (Ca0.90Eu0.07Na0.03)TiSiO5+δ were larger and smoother than those of the (Ca0.93Eu0.07)TiSiO5+δ, indicating that the Na2CO3 additive in the raw materials also functioned as a flux.
For investigating the thermal stability, DTA was measured for the (Ca0.90Eu0.07Na0.03)TiSiO5+δ and the commercial (Y0.98Eu0.02)2O2S phosphors, as shown in . For the prepared (Ca0.90Eu0.07Na0.03)TiSiO5+δ phosphor, neither evident exothermic nor endothermic behaviors were observed, and the titanite phase was maintained after heating at 1000 °C. For the commercial (Y0.98Eu0.02)2O2S phosphor, however, two exothermic peaks were clearly observed, due to the desorption of some surface adsorbates (H2O, CO2, etc.) at 330 °C and the decomposition of Y2O2S structure to Y2O3 and Y2O2SO4 phases at 850 °C (see the inset in ). From these results, it is evident that the prepared (Ca0.90Eu0.07Na0.03)TiSiO5+δ phosphor possesses considerably higher thermal stability in comparison with the commercial one.
4 Conclusion
Red emitting phosphor powders, (Ca1−x−yEuxNay)TiSiO5+δ, were synthesized by the conventional solid state reaction method and the photoluminescence properties were investigated. The optimal concentration of Eu3+ (x) was determined to be x = 0.07, at which the emission intensity was 14% compared to the commercial (Y0.98Eu0.02)2O2S phosphor. Furthermore, monovalent Na+ ion was also doped into the Ca2+ site of the (Ca0.93Eu0.07)TiSiO5+δ phosphor, in order to prevent the reduction of Ti4+ to Ti3+, which was induced by partial substitution of Ca2+ with Eu3+. As the result, the (Ca0.90Eu0.07Na0.03)TiSiO5+δ phosphor showed the highest emission intensity of 22%. From the thermal analysis results, it was evident that the present (Ca0.90Eu0.07Na0.03)TiSiO5+δ phosphor has higher thermal stability compared to the commercial (Y0.98Eu0.02)2O2S red phosphor.
Notes
Peer review under responsibility of The Ceramic Society of Japan and the Korean Ceramic Society.
References
- E.F.SchubertJ.K.KimScience308200512741278
- Y.MayamaK.KoyabuT.MasuiS.TamuraN.ImanakaJ. Alloys Compd.4182006243246
- S.W.KimT.MasuiN.ImanakaElectrochemistry772009611613
- S.W.KimT.MasuiN.ImanakaChem. Lett.402011498500
- Y.ZuoS.W.KimT.MasuiN.ImanakaECS J. Solid State Sci. Technol.32014R79R82
- R.C.DevriesR.RoyE.F.OsbornJ. Am. Ceram. Soc.381955158171
- A.CaprezP.MikhailJ.HulligerJ. Cryst. Growth1831998205216
- R.D.ShannonActa Crystallogr. Sect. A321976751767
- K.L.FrindellM.H.BartlA.PopitschG.D.StuckyAngew. Chem.114200210011004
- M.XingW.FangM.NasirY.MaJ.ZhangM.AnpoJ. Catal.2972013236243
- J.B.ZhongY.LuW.D.JiangQ.M.MengX.Y.HeJ.Z.LiY.Q.ChenJ. Hazard. Mater.168200916321635