Abstract
The electrochemical performance of NiO–SDC/SDC anode was studied. The anode was compacted by pressing, after which sintering was conducted in a conventional furnace at 1200 °C. A high-energy ball mill was used to mix the Sm0.2Ce0.8O1.9 (SDC) nanopowder and NiO. A pressing technique was applied to fabricate the NiO–SDC/SDC anode cells. The effect of different compaction pressures (200, 300, and 400 MPa) on the performance of the anodes was investigated via electrochemical impedance spectroscopy at an intermediate temperature range (600–800 °C). The nanoindentation technique and Archimedes method, which were used to measure stiffness and bulk density, respectively, revealed that increases in porosity were correlated with decreases in compaction pressure. High electrochemical performance can be achieved if the compaction pressure is decreased and the operating temperature is increased because of hydrogen spillover during the operation.
1 Introduction
Nanoscience has received increasing attraction during the 21st century, but original developments date back to as early as the 19th century [Citation1,Citation2]. Materials in different fields can be classified on the atomic and molecular scales [Citation3,Citation4]. Nanotechnology and nanoscience have wide applications, specifically in fuel cell technology.
The intermediate temperature solid oxide fuel cells (IT-SOFCs) have appealing features because of the absence of ohmic losses, and such features are particularly crucial in high-temperature applications [Citation5 Citation2013;Citation7]. SOFC is an environmentally friendly technology with low emission of SOx (sulfur oxides) and NOx (nitrogen oxides) and nearly no emission of CO2. Therefore, the possibility of acid rain and air pollution can decrease with the application of this technology. Moreover, the high-quality heat produced by SOFC can provide refrigeration and air-conditioning systems [Citation8,Citation9]. Among various materials, doped ceria solid electrolytes have become popular because of the presence of Sm cations and oxygen vacancies, by which ion transmission becomes thermally active during operation [Citation10]. Samaria-doped ceria (SDC) also has a high power density of about 1 W cm−2 at 600 °C similar to other materials, such as YSZ [Citation11 Citation2013;Citation13]. NiO is among the most significant anode materials because of its high electron transition during the oxidation process. In addition, NiO has low cost and has better chemical stability compared with other intermediate temperature SOFCs [Citation14,Citation15]. The fabrication process greatly affects the grain size of the powders [Citation10,Citation14]. Most fabrication methods have noticeable disadvantages, such as screen printing problem in the control of the ink viscosity, even if they are industrially available [Citation16,Citation17]. Nonetheless, in the ball-milling process, fewer problems have been encountered because the boundary connection among elements has increased, thereby resulting in small grain size and high surface area [Citation18 Citation2013;Citation20]. Co-pressing can subsequently lead to fabrication of bulk anode-supported cells at different pressures [Citation21]. This pressing procedure seems to be an efficient way of producing thin ceramic films and can affect the porosity and densification of the product [Citation22,Citation23]. Furthermore, sintering is a good fabrication technique to obtain good atomic combination between the electrolyte and the electrode powders. After sintering, the nanoindentation technique and Archimedes method were used to evaluate the stiffness and bulk density of samples, respectively, at different pressures (200–400 MPa). Thus, the mechanical and physical properties of the samples were determined. Electrochemical impedance spectroscopy (EIS) is typically used to measure the polarization resistance of the compacted cells by showing the response of the cells to a current density at a given applied voltage and at different operating temperatures.
The current work determined the effect of compaction pressure and operating temperature on the electrical performance of non-symmetrical NiO–SDC/SDC anode in IT-SOFC sintered in a conventional furnace.
2 Materials and methods
The raw materials, namely, NiO and SDC (Sigma Aldrich, USA), with particle sizes below 50 and 60 nm, respectively, were used at a weight ratio of 60:40 wt.%. To prepare the anode powders, the ball-milling process (Fritsch Pulverisette 6, Germany) was applied for 20 h at 200 rpm.
Similar to the method used in a previous work [Citation14], the NiO–SDC mixed powders were added to a polyethylene glycol binder and then poured into a die to produce thick, bulk, circular pellets (thickness: 2 mm; diameter: 13 mm). Finally, a thin layer of SDC was applied all over the anode surface in the die. The press (100 kN; Zwick-Roell, Germany) was then used to compact the whole powders at different pressures (200, 300, and 400 MPa). The bulk dense pellet underwent sintering for 1 h at a holding heating temperature of 1200 °C and at a heating rate of 2 °C/min. The density of the green pellets was measured using Archimedes’ method [Citation24]. Field emission scanning electron microscopy (FE-SEM-QUANTAX, Malaysia) was applied to determine the morphology of the NiO–SDC/SDC (anode/electrolyte) cells. A nanoindenter (IBIS-Fisher-Cripps, Australia) was applied on heated samples to determine their stiffness. Additionally, the Archimedes method was used to evaluate the bulk density of the samples. EIS (Nova software, version 1.9) was performed to estimate the cells’ response to the passage of the current. The operating procedure was the same as in a previous work [Citation14]. The frequency range was from 0.01 to 105 Hz at 97% H2–3% H2O. The amplitude was 10 mV. The operating temperatures were 600, 700, and 800 °C.
3 Results and discussions
The morphology of the samples compacted at three different pressure levels and then sintered at a conventional furnace (at a holding temperature of 1200 °C) was obtained via FE-SEM (). shows that the density is lower than the expected value (4.76 g/cm3) at 200 MPa (low pressure). However, at 300 and 400 MPa, the densities are 5.467 and 6.34 g/cm3, respectively. Thus, increasing the compaction pressure leads to particle deformation and to a decrease in the distance among particles, thereby ultimately increasing the bulk density [Citation25,Citation26]. When the compaction pressure increased (tight constraints were observed among particles), the pore size decreased, and more particle-to-particle contact points among grains appeared [Citation27].
Fig. 1 FE-SEM morphology of the pellets of the anode, i.e. NiO–SDC: (a) 200 MPa, (c) 300 MPa, and (e) 400 MPa; and electrolyte, i.e. SDC: (b) 200 MPa, (d) 300 MPa, and (f) 400 MPa.

indicates that the stiffness (S = dp/dh) (measured by the nanoindentation test) and bulk density of the samples increased with increasing compaction pressure [Citation15]. The bulk density corresponded with sample porosity, thereby showing that increasing the compaction pressure decreases pellet porosity. The stiffness results were analyzed, and the materials displayed resistance to plastic flow (by definition) during the indentation test. As the compaction pressure increased, the resistance of the materials against deformation also increased (). This effect was achieved because of the increase in stiffness, which may be due to the amount of porosity in the bulk materials. In other words, porosity may drastically decrease stiffness in most ceramics. Hence, a small amount of porosity could lead to a significant decrease in sample stiffness for both top (SDC; electrolyte) and bottom (NiO–SDC; anode) for all samples. The silver-coated cells (silver/NiO–SDC/SDC/silver) were fired at 150 °C for 1 h [Citation22]. Similar to previous works [Citation10,Citation22], the response of the samples to the current density and voltage was measured through data transferred to the EIS software by a current collector. When the operating temperature reached a high value (from 600 to 800 °C), Ni reduction was completed. In , the silver-coated samples were inserted inside a quartz tube, which was then placed inside a high-temperature furnace to evaluate the electrochemical properties of the samples [Citation28]. The total resistance versus operating temperature and compaction pressure is shown in .
Fig. 2 Density and contact stiffness of the sintered samples in conventional furnace at different pressures (200, 300 and 400 MPa).

Fig. 3 Schematic of the electrochemical test: (a) high temperature furnace; (b) quartz tube with silver coated sample inside; (c) silver coated sample with sample-holder.
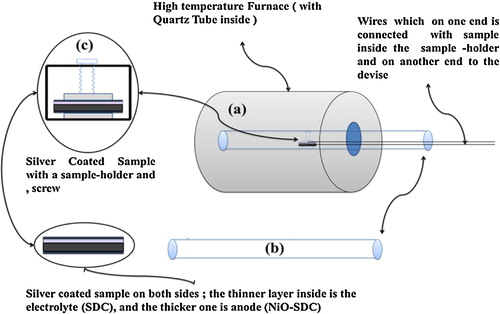
When the operating temperature and the compaction pressure increased and decreased, respectively, the total electrode polarization decreased, thereby improving electrical performance. At the interfacial sites of the electrodes and the electrolytes, the porosity of the anode had a significant effect on hydrogen diffusion into the three-phase boundary (TPB), where the electrodes, electrolyte, and gas form an interface [Citation29,Citation30]. Eq. Equation(1)(1) shows the total resistance of the sintered samples [Citation28,Citation31].
(1) In the present study, the working area was 1.32 cm2, and R1, R2, and R3 were the resistance values of the silver coat, electrolyte, and anode layer, respectively [Citation14].
At a frequency range of 0.01–105 Hz under 97% H2–3% H2O and with applied amplitude of 10 mV, EIS was applied to investigate the cells’ performance during the operation (). The Nyquist plots (imaginary part versus real part of the Fourier transform of the impedance) in reveal that the improved electrochemical behavior of the non-symmetrical pellets was caused by increasing operating temperature and decreasing ohmic resistance [Citation32,Citation33]. Increasing porosity resulted in a slightly higher ohmic resistance value at high compaction pressures compared with ohmic resistance value at low compaction pressures [Citation34]. The ionic conductivity of the non-symmetrical anode increased with increasing operating temperature [Citation31].
Fig. 5 Plot of electrochemical impedance spectroscopy (EIS) of the pellets under 97% H2–3% H2O: (a) 200 MPa, (b) 300 MPa, and (c) 400 MPa.
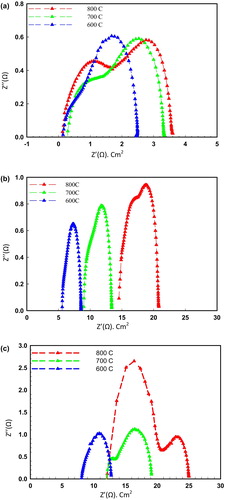
Consequently, electrochemical conductivity was enhanced at high porosity levels [Citation10,Citation35]. For gas diffusion to occur, a high porosity of bulk layers is needed because more NiO molecules could be converted to Ni with increasing temperature and decreasing bulk density [Citation28,Citation31]. The reduction may lead to slight volume shrinkage, thereby improving the porosity of the layers [Citation10,Citation22]. The effect of porosity on the electrical conductivity of SOFC was discussed in detail in our previous works [Citation14,Citation26]. However, the sintered samples at high compaction pressures exhibited remarkable electrical improvement than those at low compaction pressures [Citation10]. An increase in ion migration may explain the decrease in the total polarization resistance at a high operating temperature () [Citation10]. As stated previously [Citation14], the rate of O−2 reaction with H+ and OH− would control the anode performance [Citation10]. Hydrogen spillover determines the catalytic activity of the anode during the operation [Citation11,Citation25].
4 Conclusion
An investigation of NiO–SDC/SDC non-symmetrical anode cells, which were compacted at three different pressure levels and sintered in a conventional furnace, showed that compaction pressure played a critical role in ensuring good performance because low density may lead to good ionic transaction. The operating temperature also showed a crucial effect on the performance of the anode-supported cells. At constant pressures, an increase in operating temperature can lead to high electrochemical performance because the conversion of NiO into Ni during the operation (reduction process) would increase the porosity of the bulk cells. The FE-SEM images indicated that with decreasing pore size, the reduction process increases the contact between Ni and SDC particles, thereby increasing the performance of the NiO–SDC/SDC cells. In the nanoindentation test, the increase in stiffness of the samples with increasing compaction pressure may be due to the low extent of sample porosity. Although high-pressure levels could improve the mechanical properties of materials, a slightly higher amount of porosity should be present for ion spillover. The Archimedes method revealed that samples under higher compaction pressures exhibited greater density than those under lower compaction pressures. Low pressure and high operating temperature would improve electrochemical performance (low resistance) of conventional furnace-sintered SOFC anodes.
Acknowledgements
The authors would like to acknowledge Universiti Kebangsaan Malaysia and the Malaysian Government for research sponsorship under project funding UKM-TK-AP-05-2013.
Notes
Peer review under responsibility of The Ceramic Society of Japan and the Korean Ceramic Society.
References
- N.ZanganehS.ZanganehA.RajabiM.AllahkaramiR.Rahbari GhahnavyehA.MoghaddasM.AieneravaieN.AsadizanjaniS.SadrnezhaadJ. Coord. Chem.672014555562
- A.RajabiM.AieneravaieV.DorostiS.SadrnezhaadJ. Mater. Technol.292014227231
- A.PilkingtonL.L.LeeC.K.ChanS.RamakrishnaJ. Technol. Forecast. Soc.762009118127
- N.ZanganehA.RajabiM.TorabiM.AllahkaramiA.MoghaddasS.SadrnezhaadJ. Mol. Struct.10742014250254
- F.GrecoH.L.FrandsenA.NakajoM.F.MadsenJ. Eur. Ceram. Soc.34201426952704
- N.M.SammesR.BoveY.DuJ. Mater. Eng. Perform.152006463467
- A.B.StambouliE.TraversaJ. Renew. Sust. Energ. Rev.62002433455
- M.KawanoH.YoshidaK.HashinoH.IjichiS.SudaK.KawaharaT.InagakiJ. Power Sources17320074552
- L.NieQ.WangJ.LiuJ. Mater. Manuf. Processes29201411531156
- M.SeyednezhadA.RajabiA.MuchtarM.R.SomaluJ. Ceram. Int.41201556635669
- F.HanR.MückeT.Van GestelA.LeonideN.H.MenzlerH.P.BuchkremerD.StöverJ. Power Sources2182012157162
- M.R.SomaluV.YufitN.P.BrandonInt. J. Hydrogen Energy38201295009510
- L.SetzI.SantacruzM.ColomerS.Mello-CastanhoR.MorenoJ. Mater. Res. Bull.462011983986
- M.RazaviM.RahimipourA.RajabiJ. Mater. Technol.282013145154
- A.RajabiM.J.GhazaliA.R.DaudJ. Mater. Des.67201595106
- X.LiJ.LuH.WangJ. Sci. Sinter.432011305312
- D.WangZ.GengC.ZhangX.ZhouX.LiuJ. Energ. Chem.232014601608
- A.RajabiM.J.GhazaliJ.SyarifA.DaudChem. Eng. J.2552014445452
- H.LiT.LeiJ.ZhaoQ.ShangZ.LinJ. Mater. Manuf. Processes201516
- E.SalahiA.RajabiJ. Mater. Sci. Technol.1–6201612121217
- D.BortzmeyerDry pressing of ceramic powdersR.A.TerpstraP.P.A.C.PexA.H.VriesJ. Ceram. Proc.1995Springer102146
- M.SeyednezhadA.RajabiA.MuchtarM.R.SomaluJ. Mater. Manuf. Processes31201513011305
- Y.WuG.-Y.KimJ. Mater. Process. Technol.2162015484491
- M.R.SomaluV.YufitD.CummingE.LorenteN.P.BrandonInt. J. Hydrogen Energy36201155575566
- G.MatulaT.JardielR.JimenezB.LevenfeldA.VárezJ. Arch. Mater. Sci. Eng.3220082124
- A.HoseinpurM.JalalyM.Sh.BafghiJ.V.KhakiM.SakakiInt. J. Refract. Met. Hard Mater.542016251259
- M.RenNovel polymeric architectures through controlled/living polymerization, click chemistry and supramolecular interactions (PhD Thesis)2013The University of MelbourneAustralia Retrieve from https://minerva-access.unimelb.edu.au/handle/11343/38668
- B.B.PatilV.GanesanS.H.PawarJ. Alloys Compd.4602008680687
- S.TekeliJ. Mater. Des.282007713716
- S.A.Muhammed AliA.MuchtarA.Bakar SulongN.MuhamadE.Herianto MajlanJ. Ceram. Int.39201358135820
- T.Tadesse MollaH.L.FrandsenR.BjørkD.W.NiE.OlevskyN.PrydsJ. Eur. Ceram. Soc.2012
- J.FergusR.HuiX.LiD.P.WilkinsonJ.ZhangSolid Oxide Fuel Cells: Materials Properties and Performance2008CRC Press
- T.DeyA.DeyP.C.GhoshM.BoseA.K.MukhopadhyayR.N.BasuJ. Mater. Des.532014182191
- K.ChenX.ChenZ.LüN.AiX.HuangW.SuJ. Electrochim. Acta54200913551361
- B.BuerglerY.SantschiM.FelberbaumL.GaucklerCeram. Eng. Sci. Proc.2720083745